Design, Synthesis, and Applications of ortho -Sulfur Substituted Arylphosphanes
CCS Chemistry(2023)
摘要
Open AccessCCS ChemistryRESEARCH ARTICLES1 Jul 2022Design, Synthesis, and Applications of ortho-Sulfur Substituted Arylphosphanes Yu Guo, Ying Luo, Shiqiang Mu, Jianke Su, Jian Xu and Qiuling Song Yu Guo Institute of Next Generation Matter Transformation, College of Material Sciences Engineering, Huaqiao University, Xiamen, Fujian 361021 Google Scholar More articles by this author , Ying Luo Institute of Next Generation Matter Transformation, College of Material Sciences Engineering, Huaqiao University, Xiamen, Fujian 361021 Google Scholar More articles by this author , Shiqiang Mu Institute of Next Generation Matter Transformation, College of Material Sciences Engineering, Huaqiao University, Xiamen, Fujian 361021 Google Scholar More articles by this author , Jianke Su Institute of Next Generation Matter Transformation, College of Material Sciences Engineering, Huaqiao University, Xiamen, Fujian 361021 Google Scholar More articles by this author , Jian Xu *Corresponding author: E-mail Address: [email protected] Institute of Next Generation Matter Transformation, College of Material Sciences Engineering, Huaqiao University, Xiamen, Fujian 361021 Google Scholar More articles by this author and Qiuling Song *Corresponding author: E-mail Address: [email protected] Institute of Next Generation Matter Transformation, College of Material Sciences Engineering, Huaqiao University, Xiamen, Fujian 361021 Key Laboratory of Molecule Synthesis and Function Discovery, College of Chemistry, Fuzhou University, Fuzhou, Fujian 350108 School of Chemistry and Chemical Engineering, Henan Normal University, Xinxiang, Henan 453007 Google Scholar More articles by this author https://doi.org/10.31635/ccschem.022.202201944 SectionsSupplemental MaterialAboutAbstractPDF ToolsAdd to favoritesDownload CitationsTrack Citations ShareFacebookTwitterLinked InEmail Ortho-sulfur substituted arylphosphine oxides and arylphosphonates are the precursors of ortho-sulfurated arylphosphanes, which are widely utilized as ligands in transition metal-catalyzed reactions. However, efficient synthetic methods to access such compounds are sparse. Herein, highly valuable ortho-sulfur substituted arylphosphine oxides and arylphosphonates were constructed via difunctionalization of aryne precursors with P(O)H and elemental sulfur. This method avoids the use of transition metal catalysts and corrosive phosphorus chloride. The synthetic utility of this reaction is further demonstrated by the reduction of the synthesized products to access monophosphine ligands and their applications in cross-coupling reactions. Moreover, by introducing an alkyne moiety into the P(O)H substrate, cyclic sulfur-containing phosphines could be obtained in a one-pot reaction, which represents a new P,S structure that is inaccessible by known strategies. Download figure Download PowerPoint Introduction Ortho-sulfur substituted arylphosphanes as well as ortho-sulfur substituted arylphosphine oxides and arylphosphonates are significant as ligands in the fields of catalytic reaction1–5 and organometallic chemistry.6–10 By changing the valence of sulfur, P,S ligands show unique reactivity in organic synthesis. Ortho-sulfonate arylphosphanes can serve as bidentate ligands to coordinate with various metals including Pd, Pt, Ni, and so on (Figure 1a).11–16Ortho-sulfone and ortho-sulfur arylphosphanes show high reactivity in Pd and Au catalytic reaction.17,18 Additionally, sulfoxide has a stereocenter, which makes ortho-sulfoxide substituted arylphosphanes novel chiral phosphine ligands in asymmetric synthesis.19–24 Despite the wide application of ortho-sulfur substituted aryl phosphorus, the synthetic methods to access these molecules are underdeveloped. The classical approach relies on the lithiation of organic sulfur followed by the reaction with phosphorus chloride.25–27 Another approach is lithium diisoproplyamide-promoted Fries-type rearrangement of phosphinothiolates that must start from organic sulfides (Figure 1b).28–31 More recently, Greaney developed an i-PrMgCl-promoted phosphonothiolation of 2-iodophenyl arylsulfonates to synthesize ortho-sulfur substituted arylphosphanes, yet only one example was given.32 Obviously, the above synthetic strategies suffer from poor functional group tolerance, cumbersome operations, and difficulty in diversification of phosphorus chloride. Figure 1 | Ortho-sulfurated phosphorus ligands and synthetic approaches. (a) Selected ligands with ortho-sulfurated aryl phosphorus scaffold; (b) known strategies for the construction of ortho-sulfurated aryl phosphines; (c) phosphonothiolation of arynes with P(O)H and elemental sulfur (this work). Download figure Download PowerPoint Arynes have been widely employed in chemical synthesis as important synthetic intermediates;33–41 specifically, phosphination of arynes has emerged as an efficient strategy to access complicated phosphines, which are valuable ligands.42–47 In spite of the significant progress on the difunctionalization of arynes to render phosphane compounds,42–48 this strategy had not been applied to access ortho-sulfur substituted aryl phosphorus using P(O)H compounds as substrates. Given the importance of ortho-sulfur substituted arylphosphanes in chemical synthesis, catalysis, and organometallic chemistry, the development of effective and practical approaches for their synthesis, particularly without the addition of phosphorus chloride, is an appealing yet challenging goal in phosphorus chemistry. According to retrosynthetic analysis, ortho-sulfur substituted aryl phosphorus may be dissociated into three components: phosphorus, sulfur, and aromatic ring (Figure 1c). Thus, we envisioned an ideal and straightforward approach to combine the three readily accessible and inexpensive synthons in a single vessel reaction in the absence of transition metal reagents and corrosive phosphorus chlorides. This strategy is very challenging because of two potential competing reactions: (1) P(O)H compounds are good protic nucleophiles, which can easily lead to the formation of P-arylation products via the protonation of aryl anion intermediates;45 (2) to inhibit the proton process and suppress the side reaction, a reactive electrophile should be introduced, but P(O)H may react with the electrophile to form a byproduct. Here, we report an unprecedented S–P bond insertion to arynes to access highly valuable ortho-sulfuration aryl phosphorus (Figure 1c). Notably, when employing phosphine-containing alkynes as substrates, cyclic sulfur-containing phosphines could be readily obtained in a single vessel reaction. The synthetic utility of this reaction is further demonstrated by the reduction of the synthesized products to access ortho-sulfur substituted monophosphine ligands and their applications in cross-coupling reactions. Experimental Methods General procedure for synthesis of ortho-sulfurated arylphosphanes from arylphosphine oxides or arylphosphonates A mixture of elemental sulfur (0.2 mmol), KF (0.6 mmol), and 18-Crown-6 (0.6 mmol) were charged into a Schleck tube; then the air was removed and the Schlenk tube was back-filled with N2. To the Schleck tube was added arylphosphine oxides or arylphosphonates (0.24 mmol), aryne precursors (0.5 mmol), and tetrahydrofuran (THF; 2 mL). The reaction mixture was stirred at 60 °C for 12 h. After the solvent was removed under reduced pressure, the residue was purified by silica gel chromatography using petroleum ether/ethyl acetate (PE/EA) (2∶1) to afford the corresponding product. General procedure for synthesis of ortho-selenium substituted arylphosphanes from arylphosphine oxides or arylphosphonates A mixture of selenium (0.4 mmol), KF (0.6 mmol), and 18-Crown-6 (0.6 mmol) were charged into a Schleck tube; then the air was removed and it was back-filled with N2. To the Schleck tube was added arylphosphine oxides or arylphosphonates (0.24 mmol), aryne precursors (0.5 mmol), and THF (2 mL). The reaction mixture was stirred at 60 °C for 12 h. After the solvent was removed under reduced pressure, the residue was purified by silica gel chromatography using PE/EA (2:1) to afford the corresponding product. General procedure for synthesis of phosphacycle from alkylalkynyl phosphine oxides A mixture of elemental sulfur (0.2 mmol), KF (0.6 mmol), and 18-Crown-6 (0.6 mmol) were charged into a Schleck tube; then the air was removed, and it was back-filled with N2. To the Schleck tube was added alkylalkynyl phosphine oxides (0.2 mmol), aryne precursors (0.5 mmol), and THF (2 mL). The reaction mixture was stirred at 60 °C for 12 h. After the solvent was removed under reduced pressure, the residue was purified by silica gel chromatography using PE/EA (2:1) to afford the corresponding product. Results and Discussion To verify our hypothesis, diphenylphosphine oxide ( 1a), and 2-(trimethylsilyl)-phenyl triflate ( 3a) were chosen as model substrates to optimize the reaction. After a series of experiments, when elemental sulfur was employed as a reaction partner with CsF as a base, sulfur-substituted triarylphosphine oxide ( 4a) was obtained in 15% yield (Table 1, entry 1), and its structure was confirmed by X-ray crystal structure analysis. Subsequently, various fluoride sources such as tetrabutylammonium fluoride (TBAF), tetrabutylammonium difluorotriphenylsilicate (TBAT), and KF were evaluated, and the best result was achieved with KF/18-Crown-6, rendering the desired product 4a in 33% yield (entries 2–4). A survey of necessary equivalents was then conducted, and the data revealed that 3 equivalents of KF and 18-Crown-6 furnished the desired product in 40% yield while minimizing the side reactions (entries 5 and 6). Increasing the loading of aryne precursor 3a to 2.5 equiv further improved the yield to 83% (entry 7). Compared to THF, other solvents such as toluene, 1,4-dioxane, dichloromethane (DCM), dimethylformamide (DMF), and dimethyl sulfoxide (DMSO) all afforded reduced yields (entries 8–12; see Supporting Information Table S1). Finally, performing the reaction at lower or higher temperatures gave inferior results (entries 13–15). Control experiments showed that the reaction did not occur in the absence of KF/18-Crown-6 (entry 16). Table 1 | Optimization of Reaction Conditionsa Entry Fluorine Source (equiv) Additive (equiv) Solvent T (°C) Yield (%)b 1 c CsF (2) — THF 60 15 2 c TBAF (2) — THF 60 18 3 c TBAT (2) — THF 60 23 4 c KF (2) 18-Crown-6 (2) THF 60 33 5 c KF (3) 18-Crown-6 (3) THF 60 40 6 c KF (5) 18-Crown-6 (5) THF 60 38 7 KF (3) 18-Crown-6 (3) THF 60 83 8 KF (3) 18-Crown-6 (3) toluene 60 63 9 KF (3) 18-Crown-6 (3) dioxane 60 16 10 c KF (3) 18-Crown-6 (3) DCM 60 Trace 11 c KF (3) 18-Crown-6 (3) DMF 60 NR 12 KF (3) 18-Crown-6 (3) DMSO 60 NR 13 KF (3) 18-Crown-6 (3) THF rt 50 14 KF (3) 18-Crown-6 (3) THF 40 60 15 KF (3) 18-Crown-6 (3) THF 80 63 16 – – THF 60 NR Note: DCM, dichloromethane; DMF, dimethylformamide; DMSO, dimethyl sulfoxide; THF, tetrahydrofuran; rt, room temperature; NR, no reaction. a 1a (0.24 mmol, 1.2 equiv), 2 (0.2 mmol, 1 equiv, 6.4 mg), 3a (0.5 mmol, 2.5 equiv), solvent (2 mL), Schlenk tube, 60 °C under N2 for 12 h. bYield of isolated product. c 1a (0.2 mmol, 1.2 equiv), 3a (0.4 mmol, 2 equiv). Substrate scope With the optimized conditions in hand, the scope of the difunctionalization was explored. As summarized in Scheme 1, this protocol was applicable to a wide range of symmetrical diarylphosphine oxides with either electron-donating or electron-withdrawing substituents on different positions of the benzene rings, affording the corresponding products 4a– 4j in moderate to good yields (51–83%). Similarly, for symmetrical dialkyl phosphine oxides, the appendant ethyl, iso-propyl, benzyl, or cyclohexyl were all well tolerated, giving the corresponding products 4k– 4n in synthetically useful yields (56–68%). H-phosphonates were also suitable substrates in our reaction, and the desired products ( 4o– 4s) were obtained in good yields. The synthetic value of our reaction was further demonstrated by using unsymmetrical P(O)H compounds as substrates. A series of alkylarylphosphine oxides, which bear iso-propyl, cyclohexyl, tert-butyl, or benzyl, were all compatible and afforded the desired products 4t– 4y in moderate yields (63–71%). Notably, many unsymmetrical P(O)H compounds with aliphatic chains or different kinds of functionalities such as methoxy, alkene, alkyne, Boc, and alicyclic groups underwent the reaction smoothly to afford 4z– 4aj in good to excellent yields. P(O)H substrates derived from structurally complicated natural products such as glucose, pregnenolone, citronellol, menthol, and cholesterol, all reacted well and produced the corresponding products in synthetically useful yields ( 4ak– 4ap). These results show that our reaction not only exhibited good functional group tolerance, but also had potential applications in the synthesis of bioactive phosphorus compounds. Scheme 1 | Scope of P(O)H compounds and arynes.a Reaction conditions: a1 (0.24 mmol, 1.2 equiv), 2 (0.2 mmol), 3 (0.5 mmol, 2.5 equiv), KF (0.6 mmol, 3 equiv), 18-Crown-6 (0.6 mmol, 3 equiv), THF (2 mL) at 60 °C under N2 for 12 h. Download figure Download PowerPoint To further extend the scope of the present method, we switched our attention to aryne precursors. Symmetrical aryne precursors with two methyl groups or fluorine atoms reacted very well, affording the desired products 4aq, 4ar, and 4at in good yields; aryne with electron-donating methoxyl gave a slightly lower yield ( 4as). Moreover, 2,3-naphthalyne precursor ( 3au) also performed well, furnishing the expected product 4au in 63% yield. Furthermore, the 3-methoxybenzyne was also obtained 4av (X-ray of 4av see Supporting Information) in 40% yield. For 4aw, four mixtures were obtained. When elemental sulfur was replaced by elemental selenium and tellurium, to our delight, the reaction still worked well without any modifications of the reaction conditions (Scheme 2). The structure of 6a was also confirmed by X-ray crystal structure analysis. Next, the scope of the selenium-involved difunctionalization reaction was evaluated. As shown in Scheme 2, diversified functional groups were tolerated well and afforded the ortho-selenium substituted tertiary phosphine oxides in moderate to good yields. Both symmetrical dialkylphosphine oxides and unsymmetrical P(O)H compounds were converted into the corresponding products readily ( 6a– 6e). Moreover, naturally-occurring alcohol pregnenolone was tolerated well ( 6f). Fluorine and methyl-substituted aryne precursors were compatible with the reaction, albeit affording moderate yields ( 6g and 6h). When we used tellurium instead of selenium, the reaction delivered the desired products in low yields. Scheme 2 | Scope of the difunctionalization reaction with selenium and tellurium.a Reaction conditions: a1 (0.24 mmol, 1.2 equiv), 5 (0.2 mmol), 3 (0.5 mmol, 2.5 equiv), KF (0.6 mmol, 3 equiv), 18-Crown-6 (0.6 mmol, 3 equiv), THF (2 mL) at 60 °C under N2 for 12 h. Download figure Download PowerPoint During the study, we noticed that the aryl group on sulfur or selenium came from another aryne precursor; therefore, we envisioned that the introduction of an electrophilic moiety instead of aryne in the P(O)H compounds would trap the in situ generated sulfur anion to furnish a phosphacycle. Although phosphacycles are found in many organic n-type materials and ligands, traditional synthetic approaches require organometallic reagents and multifunctional compounds, resulting in poor functional group tolerance and prefunctionalization of starting materials. After a series of experiments, we found that alkylalkynyl phosphine oxides were suitable for this intramolecular cyclization reaction to access phosphacycle, and the structure of the product 8e was confirmed by X-ray crystal structure analysis. As shown in Scheme 3, further study on the scope of the reaction revealed that various substituted alkylalkynylphosphine oxides, both aromatic and aliphatic, readily reacted with 2-(trimethylsilyl)-phenyl triflate ( 3a). When the para-position of phenylacetylene is substituted with either electron-donating or electron-withdrawing groups, the reaction delivered the desired products in moderate yields ( 8a– 8f, 68–73%). In addition, aliphatic analogs also provided the target products in comparable yields ( 8g and 8h). To showcase its utility in transforming complicated molecules, alkylalkynylphosphine oxides derived from geraniol and cholesterol were tested, and the desired phosphacycles were obtained in moderate yields ( 8i and 8j, 56% and 60%). Gratifyingly, arynes bearing different substituents were also compatible with the standard reaction conditions, giving the corresponding phosphacycles in decent yields ( 8k– 8m). Scheme 3 | Scope of the P-alkynyl phosphine oxides.a Reaction conditions: a7 (0.2 mmol, 1 equiv), 2 (0.2 mmol, 1 equiv), 3 (0.5 mmol, 2.5 equiv), KF (0.6 mmol, 3 equiv), 18-Crown-6 (0.6 mmol, 3 equiv), THF (2 mL) at 60 °C under N2 for 12 h. Download figure Download PowerPoint To demonstrate the synthetic utility of this difunctionalization reaction, a series of transformations of the synthesized phosphine oxides were carried out. As shown in Scheme 4, reduction of 4a with HSiCl3 afforded ortho-sulfur substituted arylphosphane 9 in almost quantitative yield (Scheme 4a). Selective oxidation followed by the reduction of 4a furnished ortho-sulfone and ortho-sulfoxide substituted phosphorus ligands in excellent yields (92% and 93%; 10 and 11, Scheme 4b,c). Using (diacetoxyiodo)benzene as an oxidant and ammonium carbamate as the ammonia source, 4a was smoothly transformed into NH-sulfoximine 12 (Scheme 4d). The reaction between 9 and PdCl2 gave a palladium complex 13 in 70% yield (Scheme 4e). The X-ray crystal structure of the dichloropalladium complex 13 shows that 9 is bonded to the Pd(II) metal center with the phosphorus and sulfur atoms, thus forming a five-member ring. This palladium complex may be of interest in mechanism studies of palladium chemistry. Lithiation of 11 followed by reaction with Cy2PCl generated diphosphine ligands 14 in good yields (86%, Scheme 4f). Scheme 4 | Synthetic utility. Conditions: (a) Et3N, HSiCl3, toluene, 120 °C; (b) Et3N, HSiCl3, toluene, 120 °C; m-chloroperoxybenzoic acid (m-CPBA), DCM, −78 °C; (c) Et3N, HSiCl3, toluene, 120 °C; m-CPBA, DCM, rt; (d) PhI(OAc)2, NH2COOH.NH3, MeOH, rt; (e) PdCl2, DCM, rt; (f) t-BuLi, THF, −78 °C, Cy2PCl. Download figure Download PowerPoint Since ortho-sulfur substituted arylphosphanes are valuable ligands, we decided to employ our products to transition metal-catalyzed transformations. After a brief screening of the synthesized tertiary phosphines, we found that sulfoxide 11 exhibited high catalytic reactivity in Suzuki–Miyaura cross couplings. For example, when phenylboronic acid ( 15) and 4-methylbromobenzene ( 16) were chosen as substrates, 0.5 mol% of Pd(OAc)2 and 11 were used as catalyst and ligand, the coupling product 17a was obtained in 95% yield. Notably, when carrying out the coupling reaction on gram scale, the loading of Pd(OAc)2 and 11 was further reduced to 0.05 mol % without destruction of the yield. A survey of substrate scope showed that the reaction featured broad functional group compatibility. Steric hindrance appears to not influence the yields because sterically hindered 2-bromo-1,3-dimethylbenzene and 1-bromo-2-isopropylbenzene were both suitable substrates and the desired products ( 17b– 17c) were obtained in excellent yields (90%–86%), respectively. Moreover, sensitive groups such as amino, aldehyde, and cyano were all well tolerated in this catalyst system, the corresponding products 17d– 17f were procured in excellent yields (90–95%), which clearly demonstrated that the products from our reactions have special reactivities as ligands in Suzuki–Miyaura cross-couplings (Scheme 5). Scheme 5 | Application of 11 in Suzuki–Miyaura cross-coupling reactions. Reaction conditions: a15 (1 equiv, 1 mmol), 16 (1.5 equiv, 1.5 mmol), Pd(OAc)2 (0.005 equiv, 0.005 mmol), 11 (0.005 equiv, 0.005 mmol), Cs2CO3 (2 equiv, 2 mmol), dioxane (3 mL) at 80 °C under N2 for 12 h; b15 (1 equiv, 10 mmol), 16 (1.5 equiv, 15 mmol), Pd(OAc)2 (0.0005 equiv, 0.0005 mmol), 11 (0.0005 equiv, 0.0005 mmol), Cs2CO3 (2 equiv, 20 mmol), dioxane (30 mL) at 80 °C under N2 for 18 h. Download figure Download PowerPoint To elucidate the reaction mechanism, several control experiments were performed as depicted in Scheme 6a. First, our model reaction was performed in the standard conditions without elemental sulfur; only the P-arylation product Ph3P(O) was obtained in 56% yield (Scheme 6a, entry 1). Combining Ph3P(O) with elemental sulfur under the optimized conditions did not lead to the desired product 4a (Scheme 6a, entry 2), which indicated that Ph3P(O) was not the key intermediate in this transformation. When Ph2P(O)H was removed from the standard reaction, no new spots were detected by thin layer chromatography (Scheme 6a, entry 3). When Ph2P(O)H was allowed to react with elemental sulfur in the absence of aryne, Ph2P(O)SH 18 was obtained and a 95% crude yield was detected by 31P NMR (Scheme 6a, entry 4). Further combining 18 with 3a gave the final product 4a in 80% yield (Scheme 6a, entry 5), which revealed that 18 probably was the reaction intermediate. To further understand why protonation product Ph3P(O) was not observed in our standard conditions, we performed a competition experiment. As shown in Scheme 6b, only 4a was obtained in the standard conditions, indicating that Ph2P(O)SH ( 18) was much more reactive than Ph2P(O)H in this reaction. According to 31P NMR tracking experiments (Scheme 6c), Ph2P(O)H ( 1a) reacted with element sulfur very quickly, affording Ph2P(O)SH ( 18) in almost quantitative yield within only two minutes. This result indicates that the concentration of Ph2P(O)H was very low during the reaction process. Accordingly, both the low concentration and poor reactivity of Ph2P(O)H resulted in no formation of the protonation product Ph3P(O). Scheme 6 | Mechanistic studies. Download figure Download PowerPoint Based on the above experiments and previous work,49 a plausible mechanism is proposed in Scheme 7. First, reaction of R1R2P(O)H 1 with elemental sulfur gives R1R2P(O)SH 18 under basic condition. Then, the sulfur anion attacks benzyne which is generated in situ from 3a to form aryl anion intermediate A, which subsequently undergoes a phosphorus-involved Fries-type rearrangement to form the intermediates B and C.34,38,50–55 Finally, when R1 was not alkynyl, reaction between C and benzyne followed by a protonation renders the product 4. Alternatively, when R1 was alkynyl, phosphacycle 8 was obtained via intramolecular Michael addition. Scheme 7 | Plausible mechanism. Download figure Download PowerPoint Conclusion We have demonstrated a novel strategy for the synthesis of ortho-sulfurated arylphosphanes via transition metal-free phosphonothiolation of arynes with P(O)H and elemental sulfur. This difunctionalization reaction features a broad substrate scope, good functional group compatibility, and the potential utility in late-stage structural modification of complex natural products. Importantly, our difunctionalization reaction can largely extend the diversity of ligand libraries for ligand optimization in coupling reactions. Additionally, cyclic sulfur-containing phosphines could also be obtained via phosphonothiolation of arynes followed by an intramolecular Michael addition. Supporting Information Supporting Information is available and includes experimental procedures, analytical and spectroscopic data for new compounds, and copies of NMR spectra. Conflict of Interest The authors declare no competing financial interest. Acknowledgments Financial support from National Natural Science Foundation of China (grant nos. 21772046 and 21931013) the Natural Science Foundation of Fujian Province of China (grant nos. 2019J05095 and 2022J02009), the Promotion Program for Young and Middle-aged Teacher in Science and Technology Research of Huaqiao University (grant no. ZQN-705), and Open Research Fund of School of Chemistry and Chemical Engineering, Henan Normal University are gratefully acknowledged. The authors also thank the Instrumental Analysis Center of Huaqiao University for analysis support. Y. Guo thank the Subsidized Project for Cultivating Postgraduates’ Innovative Ability in Scientific Research of Huaqiao University. References 1. Vuoti S.; Autio J.; Haukka M.; Pursiainen J.Effective New Palladium Catalysts for the Suzuki Coupling of Various Haloxylenes to Prepare Sterically Hindered Bi- and Triaryls.Inorg. Chim. Acta2009, 362, 4685–4691. Google Scholar 2. Luo Y.; Ji K.; Li Y.; Zhang L.Tempering the Reactivities of Postulated Alpha-Oxo Gold Carbenes Using Bidentate Ligands: Implication of Tricoordinated Gold Intermediates and the Development of an Expedient Bimolecular Assembly of 2,4-Disubstituted Oxazoles.J. Am. Chem. Soc.2012, 134, 17412–17415. Google Scholar 3. Bayardon J.; Maronnat M.; Langlois A.; Rousselin Y.; Harvey P. D.; Jugé S.Modular P-Chirogenic Phosphine-Sulfide Ligands: Clear Evidence for Both Electronic Effect and P-Chirality Driving Enantioselectivity in Palladium-Catalyzed Allylations.Organometallics2015, 34, 4340–4358. Google Scholar 4. Saikia K.; Dutta D. K.Palladium Complexes of Heterobidentate Ligands: Active Catalysts for Direct Acylation of Aryl Halides with Aldehydes via C(sp2)-H Activation.J. Mol. Catal. A Chem.2015, 408, 20–25. Google Scholar 5. Liu X.; Wu C.; Zhang J.; Shi Y.; Zhang S.; Geng Y.; Tung C.-H.; Wang W.Cobalt-Catalyzed Radical Cyclization of Isocyanides Forming Phenanthridine Derivatives.Org. Chem. Front.2018, 5, 2997–3002. Google Scholar 6. Perez-Lourido P.; Romero J.; Garcia-Vazquez J.; Sousa A.; Maresca K. P.; Rose D. J.; Zubieta J.Synthesis and Characterization of Rhenium Phosphinothiolate Complexes. Crystal and Molecular Structures of [HNEt3][Re{P(C6H4S)3}2], [ReOCl{OP(C6H5)2(C6H4S)}{(P(C6H5)2(C6H4S)}], [Re2O5{P(C6H5)2(C6H4S)}2], and [ReOCl{OP(C6H5)2(2-SC6H3-3-SiMe3)}2].Inorg. Chem.1998, 37, 3331–3336. Google Scholar 7. Pérez-Lourido P.; Romero J.; García-Vázquez J. A.; Castro J.; Sousa A.; Cooper L.; Dilworth J. R.; Richards R. L.; Zheng Y.; Zubieta J. A.Synthesis and Characterisation of Iron and Cobalt Complexes with Phosphinothiolate Ligands.Inorg. Chim. Acta2003, 356, 193–202. Google Scholar 8. Vălean A. M.; Gómez-Ruiz S.; Lönnecke P.; Silaghi-Dumitrescu I.; Silaghi-Dumitrescu L.; Hey-Hawkins E.When Arsine Makes the Difference: Chelating Phosphino and Bridging Arsinoarylthiolato Gallium Complexes.Inorg. Chem.2008, 47, 11284–11293. Google Scholar 9. Huertos M. A.; Cano I.; Bandeira N. A.; Benet-Buchholz J.; Bo C.; van Leeuwen P. W.Phosphinothiolates as Ligands for Polyhydrido Copper Nanoclusters.Chem. Eur. J.2014, 20, 16121–16127. Google Scholar 10. Zhang F.; Song H.; Zhuang X.; Tung C. H.; Wang W.Iron-Catalyzed 1,2-Selective Hydroboration of N-Heteroarenes.J. Am. Chem. Soc.2017, 139, 17775–17778. Google Scholar 11. Ito S.; Munakata K.; Nakamura A.; Nozaki K.Copolymerization of Vinyl Acetate with Ethylene by Palladium/Alkylphosphine-Sulfonate Catalysts.J. Am. Chem. Soc.2009, 131, 14606–14607. Google Scholar 12. Noda S.; Kochi T.; Nozaki K.Synthesis of Allylnickel Complexes with Phosphine Sulfonate Ligands and Their Application for Olefin Polymerization without Activators.Organometallics2009, 28, 656–658. Google Scholar 13. Piche L.; Daigle J. C.; Claverie J. P.A Ruthenium Catalyst Yielding Crosslinked Polyethylene.Chem. Commun.2011, 47, 7836–7838. Google Scholar 14. Leicht H.; Gottker-Schnetmann I.; Mecking S.Incorporation of Vinyl Chloride in Insertion Polymerization.Angew. Chem. Int. Ed.2013, 52, 3963–3966. Google Scholar 15. Pal S.; Kusumoto S.; Nozaki K.Facile Styrene Formation from Ethylene and a Phenylplatinum(II) Complex Leading to an Observable Platinum(II) Hydride.Organometallics2017, 36, 502–505. Google Scholar 16. Seidel F. W.; Tomizawa I.; Nozaki K.Expedient Synthetic Identification of a P-Stereogenic Ligand Motif for the Palladium-Catalyzed Preparation of Isotactic Polar Polypropylenes.Angew. Chem. Int. Ed.2020, 59, 22591–22601. Google Scholar 17. Dodds D. L.; Boele M. D. K.; van Strijdonck G. P. F.; de Vries J. G.; van Leeuwen P. W. N. M.; Kamer P. C. J.Design, Testing and Kinetic Analysis of Bulky Monodentate Phosphorus Ligands in the Mizoroki-Heck Reaction.Eur. J. Inorg. Chem.2012, 2012, 1660–1671. Google Scholar 18. Li J.; Ji K.; Zheng R.; Nelson J.; Zhang L.Expanding the Horizon of Intermolecular Trapping of in Situ Generated Alpha-Oxo Gold Carbenes: Efficient Oxidative Union of Allylic Sulfides and Terminal Alkynes via C–C Bond Formation.Chem. Commun.2014, 50, 4130–4133. Google Scholar 19. Wang D.; Cao P.; Wang B.; Jia T.; Lou Y.; Wang M.; Liao J.Copper(I)-Catalyzed Asymmetric Pinacolboryl Addition of N-Boc-imines Using a Chiral Sulfoxide-Phosphine Ligand.Org. Lett.2015, 17, 2420–2423. Google Scholar 20. Jiang L.; Cao P.; Wang M.; Chen B.; Wang B.; Liao J.Highly Diastereo- and Enantioselective Cu-Catalyzed Borylative Coupling of 1,3-Dienes and Aldimines.Angew. Chem. Int. Ed.2016, 55, 13854–13858. Google Scholar 21. Wang X.; Wang B.; Yin X.; Yu W.; Liao Y.; Ye J.; Wang M.; Hu L.; Liao J.Palladium-Catalyzed Enantioselective Thiocarbonylation of Styrenes.Angew. Chem. Int. Ed.2019, 58, 12264–12270. Google Scholar 22. Liao Y.; Yin X.; Wang X.; Yu W.; Fang D.; Hu L.; Wang M.; Liao J.Enantioselective Synthesis of Multisubstituted Allenes by Cooperative Cu/Pd-Catalyzed 1,4-Arylboration of 1,3-Enynes.Angew. Chem. Int. Ed.2020, 59, 1176–1180. Google Scholar 23. Yin X.; Chen B.; Qiu F.; Wang X.; Liao Y.; Wang M.; Lei X.; Liao J.Enantioselective Palladium-Catalyzed Hydrofluorination of Alkenylarenes.ACS Catal.2020, 10, 1954–1960. Google Scholar 24. Wang M.; Meng X.; Cai C.; Wang L.; Gong H.Synthesis of Benzisothiazoles by a Three-Component Reaction Using Elemental Sulfur and Ammonium as Heteroatom Components Under Transition Metal-Free Conditions.Green Synth. Catal.2022, 3, 168–174. Google Scholar 25. Block E.; Ofori-Okai G.; Zubieta J.2-Phosphino- and 2-Phosphinylbenzenethiols: New Ligand Types.J. Am. Chem. Soc.1989, 111, 2327–2329. Google Scholar 26. Horner L.; Lawson A. J.; Simons G.Ortho-Lithiation of Phenylthioethers- and Some Applications.Phosphorus Sulfur Silicon Relat. Elem.2007, 12, 353–356. Google Scholar 27. Kosgei G. K.; Breen D. J.; Lamb R. W.; Livshits M. Y.; Crandall L. A.; Ziegler C. J.; Webster C. E.; Rack J. J.Controlling Photoisomerization Reactivity Through Single Functional Group Substitutions in Ruthenium Phosphine Sulfoxide Complexes.J. Am. Chem. Soc.2018, 140, 9819–9822. Google Scholar 28. Au-Yeung T.-L.; Chan K.-Y.; Haynes R. K.; Williams I. D.; Yeung L. L.Completely Stereoselective P-C Bond Formation via Base-Induced [1,3]- and [1,2]-Intramolecular Rearrangements of Aryl Phosphinates, Phosphinoamidates and Related Compounds: Generation of P-Chiral β-Hydroxy, β-Mercapto- and α-Amino Tertiary Phosphine Oxides and Phosphine Sulfides.Tetrahedron Lett.2001, 42, 457–460. Google Scholar 29. Qiu Y.; Worch J. C.; Chirdon D. N.; Kaur A.; Maurer A. B.; Amsterdam S.; Collins C. R.; Pintauer T.; Yaron D.; Bernhard S.; Noonan K. J.Tuning Thiophene with Phosphorus: Synthesis and Electronic Properties of Benzobisthiaphospholes.Chem. Eur. J.2014, 20, 7746–7751. Google Scholar 30. Zhang L.; Zhang P.; Li X.; Xu J.; Tang G.; Zhao Y.Synthesis of S-Aryl Phosphorothioates by Copper-Catalyzed Phosphorothiolation of Diaryliodonium and Arenediazonium Salts.J. Org. Chem.2016, 81, 5588–5594. Google Scholar 31. Zhang X.; Shi Z.; Shao C.; Zhao J.; Wang D.; Zhang G.; Li L.Three-Component Coupling Reaction in Water: A One-Pot Protocol for the Construction of P-S-C(sp3) and P-Se-C(sp3) Bonds.Eur. J. Org. Chem.2017, 2017, 1884–1888. Google Scholar 32. Garcia-Lopez J. A.; Cetin M.; Greaney M. F.Double Heteroatom Functionalization of Arenes Using Benzyne Three-Component Coupling.Angew. Chem. Int. Ed.2015, 54, 2156–2159. Google Scholar 33. Yoshida H.; Ohshita J.; Kunai A.Aryne, ortho-Quinone Methide, and ortho-Quinodimethane: Synthesis of Multisubstituted Arenes Using the Aromatic Reactive Intermediates.Bull. Chem. Soc. Jpn.2010, 83, 199–219. Google Scholar 34. Bhunia A.; Yetra S. R.; Biju A. T.Recent Advances in Transition-Metal-Free Carbon-Carbon and Carbon-Heteroatom Bond-Forming Reactions Using Arynes.Chem. Soc. Rev.2012, 41, 3140–3152. Google Scholar 35. Tadross P. M.; Stoltz B. M.A Comprehensive History of Arynes in Natural Product Total Synthesis.Chem. Rev.2012, 112, 3550–3577. Google Scholar 36. Bhojgude S. S.; Bhunia A.; Biju A. T.Employing Arynes in Diels-Alder Reactions and Transition-Metal-Free Multicomponent Coupling and Arylation Reactions.Acc. Chem. Res.2016, 49, 1658–1670. Google Scholar 37. Matsuzawa T.; Yoshida S.; Hosoya T.Recent Advances in Reactions between Arynes and Organosulfur Compounds.Tetrahedron Lett.2018, 59, 4197–4208. Google Scholar 38. Takikawa H.; Nishii A.; Sakai T.; Suzuki K.Aryne-Based Strategy in the Total Synthesis of Naturally Occurring Polycyclic Compounds.Chem. Soc. Rev.2018, 47, 8030–8056. Google Scholar 39. Werz D. B.; Biju A. T.Uncovering the Neglected Similarities of Arynes and Donor-Acceptor Cyclopropanes.Angew. Chem. Int. Ed.2020, 59, 3385–3398. Google Scholar 40. Shi J.; Li L.; Li Y.o-Silylaryl Triflates: A Journey of Kobayashi Aryne Precursors.Chem. Rev.2021, 121, 3892–4044. Google Scholar 41. Cao C. K.; Tretyakov E.; Chen C.Transition-Metal-Free Trifluoromethylthiolation–Acylation of Arynes by Insertion into the C–S Bonds.Green Synth. Catal.2021, 2, 62–65. Google Scholar 42. Imamoto T.; Hirakawa E.; Yamanoi Y.; Inoue T.; Yamaguchi K.; Seki H.Synthesis and Properties of New Five-Membered Heterocyclic Compounds Containing a P-B-S Linkage.J. Org. Chem.1995, 60, 7697–7700. Google Scholar 43. Dhokale R. A.; Mhaske S. B.P-Arylation: Arynes to Aryl-Phosphonates, -Phosphinates, and -Phosphine Oxides.Org. Lett.2013, 15, 2218–2221. Google Scholar 44. Shen C.; Yang G.; Zhang W.Insertion of Arynes into Arylphosphoryl Amide Bonds: One-Step Simultaneous Construction of C-N and C-P Bonds.Org. Lett.2013, 15, 5722–5725. Google Scholar 45. Chen Q.; Yan X.; Du Z.; Zhang K.; Wen C.P-Arylation of Dialkyl Phosphites and Secondary Phosphine Oxides with Arynes.J. Org. Chem.2016, 81, 276–81. Google Scholar 46. Qi N.; Zhang N.; Allu S. R.; Gao J.; Guo J.; He Y.Insertion of Arynes into P-O Bonds: One-Step Simultaneous Construction of C-P and C-O Bonds.Org. Lett.2016, 18, 6204–6207. Google Scholar 47. Huang Y.; Hu Y.; Han Y.; Ou Y.; Huo Y.; Li X.; Chen Q.Direct Synthesis of ortho-Halogenated Arylphosphonates via a Three-Component Reaction Involving Arynes.J. Org. Chem.2021, 86, 7010–7018. Google Scholar 48. Chen J.; Fan R.; Liu Z.; Tan J.Reactions of Organophosphorus Compounds with Arynes: Reactivity and Mechanism.Adv. Synth. Catal.2021, 363, 657–667. Google Scholar 49. Feng M.; Tang B.; Wang N.; Xu H.-X.; Jiang X.Ligand Controlled Regiodivergent C1 Insertion on Arynes for Construction of Phenanthridinone and Acridone Alkaloids.Angew. Chem. Int. Ed.2015, 54, 14960–14964. Google Scholar 50. Yoshida H.; Shirakawa E.; Honda Y.; Hiyama T.Addition of Ureas to Arynes: Straightforward Synthesis of Benzodiazepine and Benzodiazocine Derivatives.Angew. Chem. Int. Ed.2002, 41, 3247–3249. Google Scholar 51. Peña D.; Pérez D.; Guitián E.Insertion of Arynes into σ-Bonds.Angew. Chem. Int. Ed.2006, 45, 3579–3581. Google Scholar 52. Bhojgude S. S.; Biju A. T.Arynes in Transition-Metal-Free Multicomponent Coupling Reactions.Angew. Chem. Int. Ed.2012, 51, 1520–1522. Google Scholar 53. Umezu S.; dos Passos Gomes G.; Yoshinaga T.; Sakae M.; Matsumoto K.; Iwata T.; Alabugin I.; Shindo M.Regioselective One-Pot Synthesis of Triptycenes via Triple-Cycloadditions of Arynes to Ynolates.Angew. Chem. Int.Ed.2017, 56, 1298–1302. Google Scholar 54. Takikawa H.; Nishii A.; Takiguchi H.; Yagishita H.; Tanaka M.; Hirano K.; Uchiyama M.; Ohmori K.; Suzuki K.Intramolecular Benzyne-Phenolate [4+2] Cycloadditions.Angew. Chem. Int. Ed.2020, 59, 12440–12444. Google Scholar 55. Chakrabarty S.; Chatterjee I.; Tebben L.; Studer A.Reactions of Arynes with Nitrosoarenes-An Approach to Substituted Carbazoles.Angew. Chem. Int. Ed.2013, 52, 2968–2971. Google Scholar Previous articleNext article FiguresReferencesRelatedDetails Issue AssignmentNot Yet AssignedSupporting Information Copyright & Permissions© 2022 Chinese Chemical SocietyKeywordsphosphacyclearyneP,S ligandsortho-sulfurated arylphosphanescross-coupling reactionsAcknowledgmentsFinancial support from National Natural Science Foundation of China (grant nos. 21772046 and 21931013) the Natural Science Foundation of Fujian Province of China (grant nos. 2019J05095 and 2022J02009), the Promotion Program for Young and Middle-aged Teacher in Science and Technology Research of Huaqiao University (grant no. ZQN-705), and Open Research Fund of School of Chemistry and Chemical Engineering, Henan Normal University are gratefully acknowledged. The authors also thank the Instrumental Analysis Center of Huaqiao University for analysis support. Y. Guo thank the Subsidized Project for Cultivating Postgraduates’ Innovative Ability in Scientific Research of Huaqiao University. Downloaded 676 times PDF DownloadLoading ...
更多查看译文
关键词
synthesis
AI 理解论文
溯源树
样例
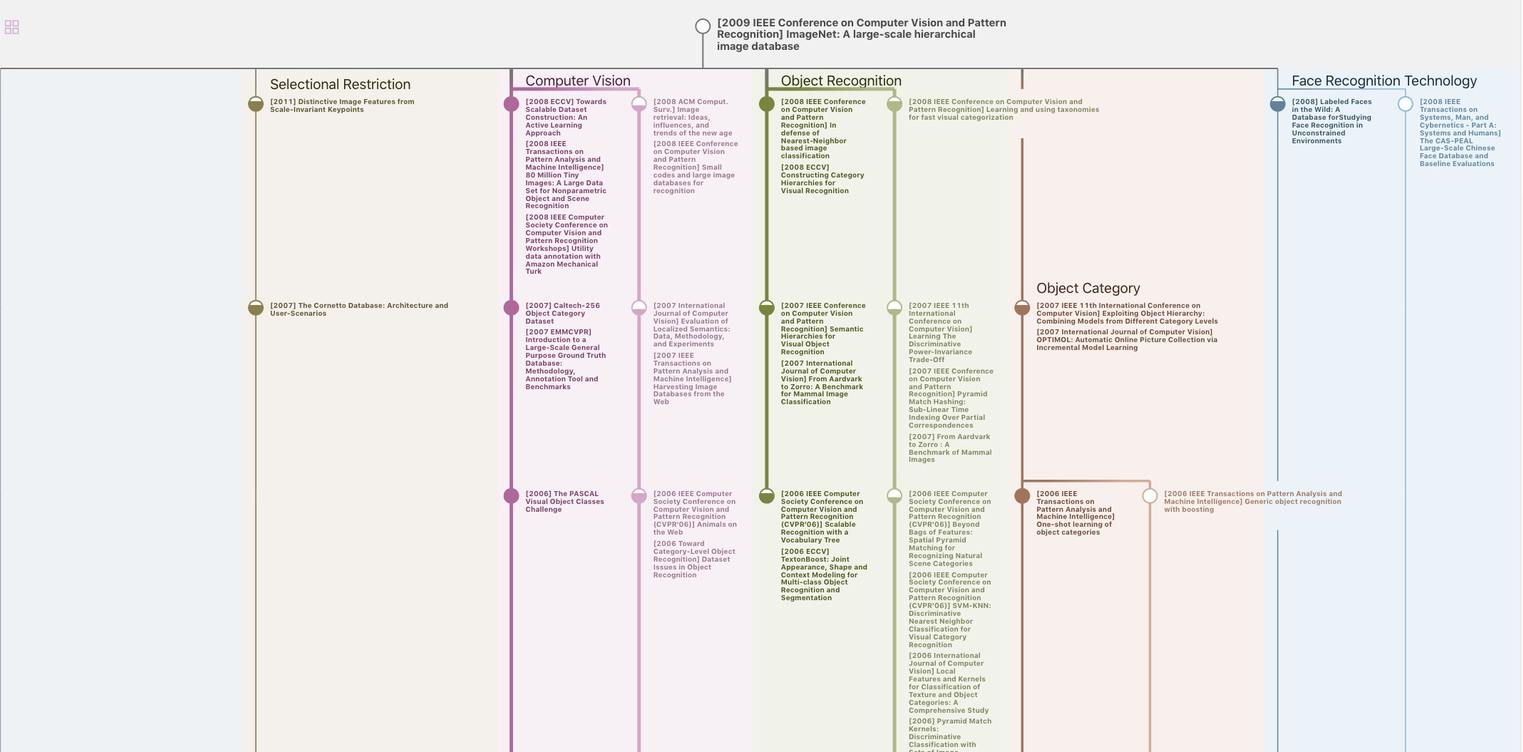
生成溯源树,研究论文发展脉络
Chat Paper
正在生成论文摘要