sTREM2 is associated with amyloid‐related p‐tau increases and glucose hypermetabolism in Alzheimer's disease
EMBO Molecular Medicine(2023)
摘要
Report9 January 2023Open Access Source DataTransparent process sTREM2 is associated with amyloid-related p-tau increases and glucose hypermetabolism in Alzheimer's disease Davina Biel Davina Biel orcid.org/0000-0002-2597-1992 Institute for Stroke and Dementia Research (ISD), University Hospital, LMU Munich, Munich, Germany Contribution: Conceptualization, Data curation, Formal analysis, Investigation, Writing - original draft, Writing - review & editing Search for more papers by this author Marc Suárez-Calvet Marc Suárez-Calvet orcid.org/0000-0002-2993-569X Barcelonaβeta Brain Research Center (BBRC), Pasqual Maragall Foundation, Barcelona, Spain IMIM (Hospital del Mar Medical Research Institute), Barcelona, Spain Servei de Neurologia, Hospital del Mar, Barcelona, Spain Centro de Investigación Biomédica en Red de Fragilidad y Envejecimiento Saludable (CIBERFES), Madrid, Spain Contribution: Writing - review & editing Search for more papers by this author Paul Hager Paul Hager Institute of Radiology and Artificial Intelligence and Informatics in Medicine, TU Munich, Munich, Germany Contribution: Data curation, Writing - review & editing Search for more papers by this author Anna Rubinski Anna Rubinski Institute for Stroke and Dementia Research (ISD), University Hospital, LMU Munich, Munich, Germany Contribution: Writing - review & editing Search for more papers by this author Anna Dewenter Anna Dewenter Institute for Stroke and Dementia Research (ISD), University Hospital, LMU Munich, Munich, Germany Contribution: Writing - review & editing Search for more papers by this author Anna Steward Anna Steward Institute for Stroke and Dementia Research (ISD), University Hospital, LMU Munich, Munich, Germany Contribution: Writing - review & editing Search for more papers by this author Sebastian Roemer Sebastian Roemer Institute for Stroke and Dementia Research (ISD), University Hospital, LMU Munich, Munich, Germany Department of Neurology, University Hospital, LMU Munich, Munich, Germany Contribution: Writing - review & editing Search for more papers by this author Michael Ewers Michael Ewers Institute for Stroke and Dementia Research (ISD), University Hospital, LMU Munich, Munich, Germany German Center for Neurodegenerative Diseases (DZNE), Munich, Germany Contribution: Writing - review & editing Search for more papers by this author Christian Haass Christian Haass German Center for Neurodegenerative Diseases (DZNE), Munich, Germany Munich Cluster for Systems Neurology (SyNergy), Munich, Germany Chair of Metabolic Biochemistry, Biomedical Center (BMC), Faculty of Medicine, LMU Munich, Munich, Germany Contribution: Data curation, Writing - review & editing Search for more papers by this author Matthias Brendel Matthias Brendel orcid.org/0000-0002-9247-2843 German Center for Neurodegenerative Diseases (DZNE), Munich, Germany Munich Cluster for Systems Neurology (SyNergy), Munich, Germany Department of Nuclear Medicine, University Hospital, LMU Munich, Munich, Germany Contribution: Conceptualization, Writing - review & editing Search for more papers by this author Nicolai Franzmeier Corresponding Author Nicolai Franzmeier [email protected] orcid.org/0000-0001-9736-2283 Institute for Stroke and Dementia Research (ISD), University Hospital, LMU Munich, Munich, Germany Munich Cluster for Systems Neurology (SyNergy), Munich, Germany Department of Psychiatry and Neurochemistry, Institute of Neuroscience and Physiology, The Sahlgrenska Academy, University of Gothenburg, Gothenburg, Germany Contribution: Conceptualization, Data curation, Formal analysis, Investigation, Writing - original draft, Writing - review & editing Search for more papers by this author for the Alzheimer's Disease Neuroimaging Initiative (ADNI) the Alzheimer's Disease Neuroimaging Initiative (ADNI) Data used in preparation of this article were obtained from the Alzheimer's Disease Neuroimaging Initiative (ADNI) database (adni.loni.usc.edu). As such, the investigators within the ADNI contributed to the design and implementation of ADNI and/or provided data but did not participate in analysis or writing of this report. A complete listing of ADNI investigators can be found at: http://adni.loni.usc.edu/wp-content/uploads/how_to_apply/ADNI_Acknowledgement_List.pdf Search for more papers by this author Davina Biel Davina Biel orcid.org/0000-0002-2597-1992 Institute for Stroke and Dementia Research (ISD), University Hospital, LMU Munich, Munich, Germany Contribution: Conceptualization, Data curation, Formal analysis, Investigation, Writing - original draft, Writing - review & editing Search for more papers by this author Marc Suárez-Calvet Marc Suárez-Calvet orcid.org/0000-0002-2993-569X Barcelonaβeta Brain Research Center (BBRC), Pasqual Maragall Foundation, Barcelona, Spain IMIM (Hospital del Mar Medical Research Institute), Barcelona, Spain Servei de Neurologia, Hospital del Mar, Barcelona, Spain Centro de Investigación Biomédica en Red de Fragilidad y Envejecimiento Saludable (CIBERFES), Madrid, Spain Contribution: Writing - review & editing Search for more papers by this author Paul Hager Paul Hager Institute of Radiology and Artificial Intelligence and Informatics in Medicine, TU Munich, Munich, Germany Contribution: Data curation, Writing - review & editing Search for more papers by this author Anna Rubinski Anna Rubinski Institute for Stroke and Dementia Research (ISD), University Hospital, LMU Munich, Munich, Germany Contribution: Writing - review & editing Search for more papers by this author Anna Dewenter Anna Dewenter Institute for Stroke and Dementia Research (ISD), University Hospital, LMU Munich, Munich, Germany Contribution: Writing - review & editing Search for more papers by this author Anna Steward Anna Steward Institute for Stroke and Dementia Research (ISD), University Hospital, LMU Munich, Munich, Germany Contribution: Writing - review & editing Search for more papers by this author Sebastian Roemer Sebastian Roemer Institute for Stroke and Dementia Research (ISD), University Hospital, LMU Munich, Munich, Germany Department of Neurology, University Hospital, LMU Munich, Munich, Germany Contribution: Writing - review & editing Search for more papers by this author Michael Ewers Michael Ewers Institute for Stroke and Dementia Research (ISD), University Hospital, LMU Munich, Munich, Germany German Center for Neurodegenerative Diseases (DZNE), Munich, Germany Contribution: Writing - review & editing Search for more papers by this author Christian Haass Christian Haass German Center for Neurodegenerative Diseases (DZNE), Munich, Germany Munich Cluster for Systems Neurology (SyNergy), Munich, Germany Chair of Metabolic Biochemistry, Biomedical Center (BMC), Faculty of Medicine, LMU Munich, Munich, Germany Contribution: Data curation, Writing - review & editing Search for more papers by this author Matthias Brendel Matthias Brendel orcid.org/0000-0002-9247-2843 German Center for Neurodegenerative Diseases (DZNE), Munich, Germany Munich Cluster for Systems Neurology (SyNergy), Munich, Germany Department of Nuclear Medicine, University Hospital, LMU Munich, Munich, Germany Contribution: Conceptualization, Writing - review & editing Search for more papers by this author Nicolai Franzmeier Corresponding Author Nicolai Franzmeier [email protected] orcid.org/0000-0001-9736-2283 Institute for Stroke and Dementia Research (ISD), University Hospital, LMU Munich, Munich, Germany Munich Cluster for Systems Neurology (SyNergy), Munich, Germany Department of Psychiatry and Neurochemistry, Institute of Neuroscience and Physiology, The Sahlgrenska Academy, University of Gothenburg, Gothenburg, Germany Contribution: Conceptualization, Data curation, Formal analysis, Investigation, Writing - original draft, Writing - review & editing Search for more papers by this author for the Alzheimer's Disease Neuroimaging Initiative (ADNI) the Alzheimer's Disease Neuroimaging Initiative (ADNI) Data used in preparation of this article were obtained from the Alzheimer's Disease Neuroimaging Initiative (ADNI) database (adni.loni.usc.edu). As such, the investigators within the ADNI contributed to the design and implementation of ADNI and/or provided data but did not participate in analysis or writing of this report. A complete listing of ADNI investigators can be found at: http://adni.loni.usc.edu/wp-content/uploads/how_to_apply/ADNI_Acknowledgement_List.pdf Search for more papers by this author Author Information Davina Biel1, Marc Suárez-Calvet2,3,4,5, Paul Hager6, Anna Rubinski1, Anna Dewenter1, Anna Steward1, Sebastian Roemer1,7, Michael Ewers1,8, Christian Haass8,9,10, Matthias Brendel8,9,11,†, Nicolai Franzmeier *,1,9,12,† and 1Institute for Stroke and Dementia Research (ISD), University Hospital, LMU Munich, Munich, Germany 2Barcelonaβeta Brain Research Center (BBRC), Pasqual Maragall Foundation, Barcelona, Spain 3IMIM (Hospital del Mar Medical Research Institute), Barcelona, Spain 4Servei de Neurologia, Hospital del Mar, Barcelona, Spain 5Centro de Investigación Biomédica en Red de Fragilidad y Envejecimiento Saludable (CIBERFES), Madrid, Spain 6Institute of Radiology and Artificial Intelligence and Informatics in Medicine, TU Munich, Munich, Germany 7Department of Neurology, University Hospital, LMU Munich, Munich, Germany 8German Center for Neurodegenerative Diseases (DZNE), Munich, Germany 9Munich Cluster for Systems Neurology (SyNergy), Munich, Germany 10Chair of Metabolic Biochemistry, Biomedical Center (BMC), Faculty of Medicine, LMU Munich, Munich, Germany 11Department of Nuclear Medicine, University Hospital, LMU Munich, Munich, Germany 12Department of Psychiatry and Neurochemistry, Institute of Neuroscience and Physiology, The Sahlgrenska Academy, University of Gothenburg, Gothenburg, Germany † These authors contributed equally to this work *Corresponding author. Tel: +49 89 4400 46162; E-mail: [email protected] EMBO Mol Med (2023)15:e16987https://doi.org/10.15252/emmm.202216987 PDFDownload PDF of article text and main figures.PDF PLUSDownload PDF of article text, main figures, expanded view figures and appendix. Peer ReviewDownload a summary of the editorial decision process including editorial decision letters, reviewer comments and author responses to feedback. ToolsAdd to favoritesDownload CitationsTrack CitationsPermissions ShareFacebookTwitterLinked InMendeleyWechatReddit Figures & Info Abstract Microglial activation occurs early in Alzheimer's disease (AD) and previous studies reported both detrimental and protective effects of microglia on AD progression. Here, we used CSF sTREM2 to investigate disease stage-dependent drivers of microglial activation and to determine downstream consequences on AD progression. We included 402 patients with measures of earliest beta-amyloid (CSF Aβ1-42) and late-stage fibrillary Aβ pathology (amyloid-PET centiloid), as well as sTREM2, p-tau181, and FDG-PET. To determine disease stage, we stratified participants into early Aβ-accumulators (Aβ CSF+/PET−; n = 70) or late Aβ-accumulators (Aβ CSF+/PET+; n = 201) plus 131 controls. In early Aβ-accumulators, higher centiloid was associated with cross-sectional/longitudinal sTREM2 and p-tau181 increases. Further, higher sTREM2 mediated the association between centiloid and cross-sectional/longitudinal p-tau181 increases and higher sTREM2 was associated with FDG-PET hypermetabolism. In late Aβ-accumulators, we found no association between centiloid and sTREM2 but a cross-sectional association between higher sTREM2, higher p-tau181 and glucose hypometabolism. Our findings suggest that a TREM2-related microglial response follows earliest Aβ fibrillization, manifests in inflammatory glucose hypermetabolism and may facilitate subsequent p-tau181 increases in earliest AD. Synopsis In Alzheimer's disease (AD), microglial activation has been linked to both detrimental and protective effects on disease progression. This study used CSF sTREM2 to investigate disease stage-dependent drivers of microglial activation and to determine downstream consequences on AD progression. In participants with early beta-amyloid (Aβ) accumulation, higher amyloid-PET was associated with higher cross sectional/longitudinal CSF sTREM2 and CSF p-tau181 levels. In participants with early Aβ accumulation, sTREM2 mediated the association between amyloid-PET and CSF p-tau181 increases. Higher CSF sTREM2 levels were associated with FDG-PET glucose hypermetabolism in early Aβ accumulation but with glucose hypometabolism in late Aβ accumulation. The paper explained Problem Besides beta-amyloid (Aβ) and tau accumulation, microglial activation plays a role in the pathogenesis of Alzheimer's disease (AD). Previous studies reported both detrimental and protective effects of microglia on AD progression, thus, it is critical to investigate at which AD stages microglial activation could be protective or detrimental to evaluate microglia as a treatment target. To address this, we used CSF sTREM2 (i.e., soluble Triggering receptor expressed on myeloid cells 2) to investigate disease stage-dependent drivers of microglial activation and to determine downstream consequences on AD biomarker progression. Results We first stratified groups into early and late Aβ-accumulators to investigate disease stage-dependent effects of CSF sTREM2 on AD progression. To that end, Aβ CSF positive but still amyloid-PET negative participants were classified as early Aβ-accumulators and participants that were both Aβ CSF and PET positive were classified as late Aβ-accumulators. We found that in early Aβ-accumulators, higher amyloid-PET was associated with cross-sectional/longitudinal CSF sTREM2 and CSF p-tau181 increases, suggesting reactive microglial and p-tau increases in response to earliest Aβ fibrillization. Further, higher CSF sTREM2 mediated the association between amyloid-PET and cross-sectional/longitudinal CSF p-tau181 increases and higher CSF sTREM2 was associated with FDG-PET hypermetabolism in line with previous findings of increased glucose consumption of activated microglia. In late Aβ-accumulators, we found no association between amyloid-PET and CSF sTREM2 but a cross-sectional association between higher CSF sTREM2, higher CSF p-tau181 and glucose hypometabolism, suggesting that sTREM2 parallels tau and neurodegeneration rather than Aβ once fully developed Aβ pathology is present. Impact Our findings suggest that sTREM2-related microglial activation occurs in response to earliest Aβ fibrillization, manifests in inflammatory glucose hypermetabolism and may facilitate subsequent p-tau increases in earliest AD, while previous reports of protective sTREM2 effects may occur in later AD stages. Introduction Alzheimer's disease (AD) is characterized by the accumulation of beta-amyloid (Aβ), tau, microglial activation, metabolic brain changes, neurodegeneration, and cognitive decline (Jack et al, 2018). According to the amyloid cascade hypothesis, Aβ accumulation triggers the subsequent development and spreading of hyperphosphorylated tau aggregates which is followed by neurodegeneration, metabolic decline, and eventually dementia (Karran et al, 2011; La Joie et al, 2020; Haass & Selkoe, 2022; Strom et al, 2022). Previously it was shown that Aβ-related increases in soluble hyperphosphorylated tau (i.e., p-tau), detectable in plasma (Janelidze et al, 2021; Moscoso et al, 2021) and cerebrospinal fluid (CSF) (Mattsson-Carlgren et al, 2020), precede tau aggregation. We have shown recently that soluble p-tau increases may in fact drive tau aggregation and spread across interconnected brain regions (Pichet Binette et al, 2022) therefore, soluble p-tau increases may be a key link between Aβ deposition and tau aggregation in AD. However, the underlying mechanisms that link Aβ and subsequent increases in soluble p-tau in CSF or plasma are not well understood. Here, activation of microglia, the brains innate immune system, may play a key role in modulating these initial events in the amyloid cascade (Pascoal et al, 2021). The Triggering Receptor Expressed on Myeloid Cell 2 (TREM2) regulates the change of microglia from a homeostatic state to a disease associated state (Keren-Shaul et al, 2017; Krasemann et al, 2017) and is a well-established in vivo proxy for microglial activation in AD (Suarez-Calvet et al, 2016, 2019; Ewers et al, 2019, 2020; Franzmeier et al, 2020). Yet, previous studies have yielded conflicting findings on a detrimental or protective role of microglial activation or TREM2-related microglial responses in AD. For instance, recent in vitro studies reported that activated microglia can induce tau hyperphosphorylation and spread (Maphis et al, 2015) and that activated microglia can release tau seeds which can induce tau aggregation (Brelstaff et al, 2021). Similarly, studies in sporadic AD patients found that a TREM2-related microglial response is strongly correlated with soluble p-tau but not with Aβ levels (Suárez-Calvet et al, 2019) and that a TREM2-related microglial response may promote the development of aggregated tau pathology in AD, as measured via tau-PET (Vogels et al, 2019; Pascoal et al, 2021). Indeed, a recent post-mortem study investigating the mediating effect of microglial activation on the Aβ to tau association in brain tissue revealed a mediation effect of 33% of microglia for the relationship between Aβ and tau (Casaletto et al, 2022). This suggests that microglial activation may be associated with tau hyperphosphorylation and therefore contribute to the development of tau pathology in AD. In addition, activated microglia have been shown to consume high levels of glucose in AD mouse models and AD patients (Xiang et al, 2021), which may manifest in hypermetabolic brain changes that are observed in early-stage AD, when neurodegeneration and ensuing glucose hypometabolism are not yet apparent (Oh et al, 2016; Gordon et al, 2018). Thus, glucose hypermetabolism in early AD may not reflect a compensatory mechanism, as suggested previously, but rather reflect activated microglia and neuroinflammation (Ashraf et al, 2015; Arenaza-Urquijo et al, 2017). On the contrary, in symptomatic sporadic AD patients and patients with autosomal dominantly inherited AD, a higher TREM2-related microglial response has been associated with attenuated cognitive decline, amyloid accumulation and neurodegeneration (Ewers et al, 2019, 2020; Morenas-Rodriguez et al, 2022). This suggests a possible protective effect of chronic microglial activation on neuronal integrity and cognition that occurs once pathologic brain changes are severe enough to result in clinically manifested cognitive deficits. Similarly, an animal model has shown that TREM2 loss of function is associated with increased Aβ seeding, further suggesting a protective effect of microglia on Aβ pathology development (Parhizkar et al, 2019). It is therefore of utmost importance for clinical trials trying to target microglial activation as a disease modifying approach to understand (i) what drives microglial activation in AD, (ii) whether and when microglial activation is beneficial or detrimental and (iii) whether the directionality of microglial effects on AD progression depends on disease stage. In the present study, we used CSF soluble TREM2 (sTREM2) as an in vivo marker of TREM2-related microglial responses in a well-characterized sample of AD patients and controls to investigate drivers of microglial activation across early versus late-stage Aβ accumulation in AD and its effects on the development of downstream p-tau and metabolic brain changes. Specifically, we included data of 402 cognitively normal (CN) and mild cognitive impaired (MCI) participants from the ADNI database with available CSF Aβ1-42, p-tau181, and sTREM2, as well as amyloid-PET, and 18F-fluorodeoxyglucose PET (FDG-PET). FDG-PET is well established for assessing cerebral glucose uptake and FDG-PET-assessed hypermetabolism has been previously linked to microglial activation (Xiang et al, 2021). To determine disease stage, we classified patients into Aβ CSF+/PET− (early Aβ-accumulators) and Aβ CSF+/PET+ (late Aβ-accumulators) following a previously established approach that allows stratifying individuals by showing earliest signs of Aβ accumulation (i.e., Aβ CSF+/PET−) versus showing fully developed amyloid pathology (Aβ CSF+/PET+) (Palmqvist et al, 2017). A total of 131 participants without evidence of Aβ pathology were included as healthy controls (Aβ CSF−/PET−). A subset of participants had available longitudinal p-tau181 and sTREM2 assessments, based on which we calculated annual sTREM2 and p-tau181 change rates. Our specific aims were to assess first, whether earliest signs of Aβ accumulation (i.e., in Aβ CSF+/PET−) are associated with a TREM2-related microglial response and second, whether this initial Aβ-driven microglial activation facilitates subsequent increases in soluble hyperphosphorylated tau (i.e., p-tau181). Third, we assessed whether earliest TREM2-related microglial responses are reflected in increased FDG-PET-assessed glucose metabolism, given that activated microglia consume large amounts of glucose (Xiang et al, 2021). Here, we expected a higher TREM2-related microglial response to be associated with glucose hypermetabolism in patients with earliest Aβ accumulation, where neurodegeneration is not yet apparent, versus hypometabolism in chronic AD phases within late Aβ-accumulators. Results We stratified participants by evidence for early- versus late-stage Aβ pathology, using a previously established approach that combines CSF assessments of soluble Aβ1-42 and amyloid-PET assessments of fibrillar Aβ (Palmqvist et al, 2017). CSF Aβ abnormality is assumed to reflect early Aβ dysmetabolism and precedes amyloid-PET positivity reflecting mostly fibrillary forms of Aβ (Palmqvist et al, 2016). Therefore, we grouped participants into (i) early Aβ-accumulators (i.e., Aβ CSF+/PET−; n = 70; CN = 30; MCI = 40) with evidence for reduced Aβ1-42 in CSF but no suprathreshold fibrillar Aβ pathology on PET versus (ii) late Aβ-accumulators (i.e., Aβ CSF+/PET+; n = 201; CN = 41; MCI = 160), with evidence for abnormal Aβ in both CSF and PET. An additional pool of 131 cognitively normal subjects without evidence of abnormal Aβ on either CSF or PET was included as a control group. Longitudinal CSF data were available for a subset of participants for sTREM2 (early Aβ/late Aβ/controls n = 21/75/35) and p-tau181 (early Aβ/late Aβ/controls n = 20/75/35) with an average follow-up time from baseline CSF assessment of 1.99 ± 0.09 years. Descriptive baseline statistics stratified by groups are shown in Table 1. Table 1. Demographic and clinical data stratified by group. Controls (Aβ CSF−/PET−) Early Aβ-accumulators (Aβ CSF+/PET−) Late Aβ-accumulators (Aβ CSF+/PET+) P-value Cross-sectional N 131 70 201 Diagnostic (CN/MCI) 131/0 30/40 41/160 < 0.001 Sex (male/female) 64/67 47/23 112/89 0.045 Age in years 72.67 (6.39) 71.65 (7.68) 73.60 (6.53) 0.093 Years of education 16.85 (2.43)a 17.13 (2.24)a 16.00 (2.74)b,c < 0.001 CSF Aβ1-42 (pg/ml) 1,553.122 (281.109)a,c 777.819 (147.169)a,b 656.42 (174.79)b,c < 0.001 CSF p-tau181 (pg/ml) 20.241 (7.059)a,c 15.825 (7.493)a,b 32.807 (14.780)b,c < 0.001 CSF sTREM2 (pg/ml) 4,099.933 (1,980.197)c 2,955.118 (1,751.746)a,b 3,984.107 (2,194.033)c < 0.001 Amyloid-PET (centiloid) −9.0315 (12.656)a −2.901 (13.530)a 78.228 (33.871)b,c < 0.001 FDG-PET global z-score - −0.132 (0.649) −0.251 (0.532) 0.13 FDG-PET meta-ROI z-score - −0.214 (0.826)a −0.546 (0.723)c 0.002 Longitudinal N 35 20 75 Follow-up CSF p-tau181 (mean years) 1.96 (0.13)c 2.05 (0.08)a,b 1.99 (0.07)c 0.002 N 35 21 75 Follow-up CSF sTREM2 (mean years) 1.96 (0.13)c 2.05 (0.08)b 1.99 (0.07) 0.004 Values are presented as mean (SD); P-values were derived from ANOVAs for continuous measures and from Chi-squared tests for categorical measures. Mean values significantly (P < 0.05, post hoc tests) different from—. a Controls. b Early Aβ-accumulators. c Late Aβ-accumulators. Early but not late-stage Aβ accumulation is associated with higher CSF sTREM2 We tested first whether evidence for earliest Aβ abnormality in CSF but not yet in PET (i.e., early Aβ accumulators) is associated with a sTREM2-related microglial response and p-tau181 increases. To this end, we used linear regression to determine the association between amyloid-PET as a marker of fibrillary Aβ pathology (i.e., centiloid) and sTREM2 in early Aβ-accumulators. Here, higher centiloid at baseline was associated with higher cross-sectional p-tau181 (β = 0.259, T = 2.199, P = 0.032; Fig 1A, top panel) and higher sTREM2 (β = 0.254, T = 2.268, P = 0.027; Fig 1B, top panel). Further, higher sTREM2 levels were associated with higher p-tau181 levels (β = 0.587, T = 5.425, P < 0.001). We obtained congruent results using longitudinal CSF data, showing that higher centiloid at baseline was associated with faster subsequent change rates in p-tau181 (β = 0.550, T = 2.975, P = 0.010; Fig 1C, top panel) and faster changes in sTREM2 (β = 0.535, T = 3.725, P = 0.002; Fig 1D, top panel). In addition, higher baseline sTREM2 levels were associated with faster subsequent change rates in p-tau181 (β = 0.938, T = 6.286, P < 0.001). Using bootstrapped mediation analyses with 1,000 iterations, we additionally found that the association between higher centiloid and higher p-tau181 was fully mediated by sTREM2 in early Aβ accumulators, both for cross-sectional p-tau181 (average causal mediation effect [ACME]: B = 0.133, 95% CI = 0.0039 to 0.27, P = 0.038; Fig 1E, top panel) as well as subsequent p-tau181 change rates (ACME: B = 0.450, 95% CI = 0.1352 to 0.82, P = 0.004; Fig 1F, top panel). Testing the reverse mediation models, i.e., whether p-tau181 mediates the effect of centiloid on sTREM2 yielded a similar mediation effect for cross-sectional sTREM2 levels (ACME: B = 0.132, CI = 0.0084 to 0.26, P = 0.030) but a much lower mediation effect for subsequent sTREM2 change rates in longitudinal analyses (ACME: B = 0.273, CI = 0.0437 to 0.55, P = 0.014). These results suggest that earliest Aβ accumulation may induce a reactive TREM2-related microglial response which may in turn facilitate p-tau181 increases. Figure 1. Cross-sectional and longitudinal analysis of the association between amyloid-PET (in centiloid), CSF p-tau181, and CSF sTREM2 in early Aβ-accumulators (i.e., Aβ CSF+/PET−; n = 70) and late Aβ-accumulators (i.e., Aβ CSF+/PET+; n = 201) A–D. Cross-sectional linear regressions between centiloid and p-tau181 (A) and centiloid and sTREM2 (B). Longitudinal linear regressions between centiloid and change in p-tau181 (C) and centiloid and change in sTREM2 (D). Standardized beta-estimates (β), T-values, and P-values were derived from linear regressions. E–F. Cross-sectional mediation analyses with centiloid as predictor, sTREM2 as mediator, and p-tau181 as dependent variable (E). Longitudinal mediation analyses with centiloid as predictor, sTREM2 as mediator, and change in p-tau181 as dependent variable (F). Beta-estimates (B) and P-values for each path are displayed on the respective arrow. The average causal mediation effect (ACME) and the average direct effect (ADE) are displayed under each mediation triangle. Data information: Early Aβ-accumulators are displayed in green, and late Aβ-accumulators in orange. All models are controlled for age, sex, education, and clinical status. Source data are available online for this figure. Source Data for Figure 1 [emmm202216987-sup-0004-SDataFig1.zip] Download figure Download PowerPoint When assessing the above-described analyses in late Aβ-accumulators, no association was found between centiloid and sTREM2, neither for cross-sectional sTREM2 (β = 0.040, T = 0.565, P = 0.573; Fig 1B, bottom panel) nor for longitudinal sTREM2 change rates (β = 0.051, T = 0.450, P = 0.654; Fig 1D, bottom panel). This suggests that sTREM2 increases are no longer driven by Aβ once fully developed fibrillar Aβ pathology is present. Yet, there was an association between higher baseline sTREM2, higher baseline p-tau181 (β = 0.441, T = 7.004, P < 0.001) and longitudinal changes in p-tau181 (β = 0.439, T = 3.777, P < 0.001), suggesting that sTREM2 is more strongly coupled to p-tau181 in late Aβ-accumulators. Higher centiloid was associated with higher cross-sectional p-tau181 (β = 0.203, T = 2.995, P = 0.003; Fig 1A, bottom panel), but no association was found between baseline centiloid and subsequent p-tau181 change rates (n = 75, β = 0.137, T = 1.165, P = 0.248; Fig 1C, bottom panel). Given that there was no association between centiloid and sTREM2 in late Aβ-accumulators, we did not detect a mediation effect of sTREM2 for the association between centiloid and p-tau181, neither cross-sectionally (ACME: B = 0.017, CI = −0.0444 to 0.08, P = 0.560; Fig 1E, bottom panel), nor longitudinally (ACME: B = 0.003, CI = −0.108 to 0.10, P = 0.960; Fig 1F, bottom panel). Together, these findings suggest that earliest fibrillization of Aβ is associated with reactive sTREM2 increases, which may in turn precede increases in p-tau181 in early Aβ-accumulators, while sTREM2 dynamics may uncouple from the extent of fibrillar Aβ pathology at later stages but rather parallel p-tau181 increases. All analyses remained consistent when including APOE4 genotype as a covariate (except for the cross-sectional A
更多查看译文
关键词
alzheimer disease,amyloid‐related,glucose hypermetabolism
AI 理解论文
溯源树
样例
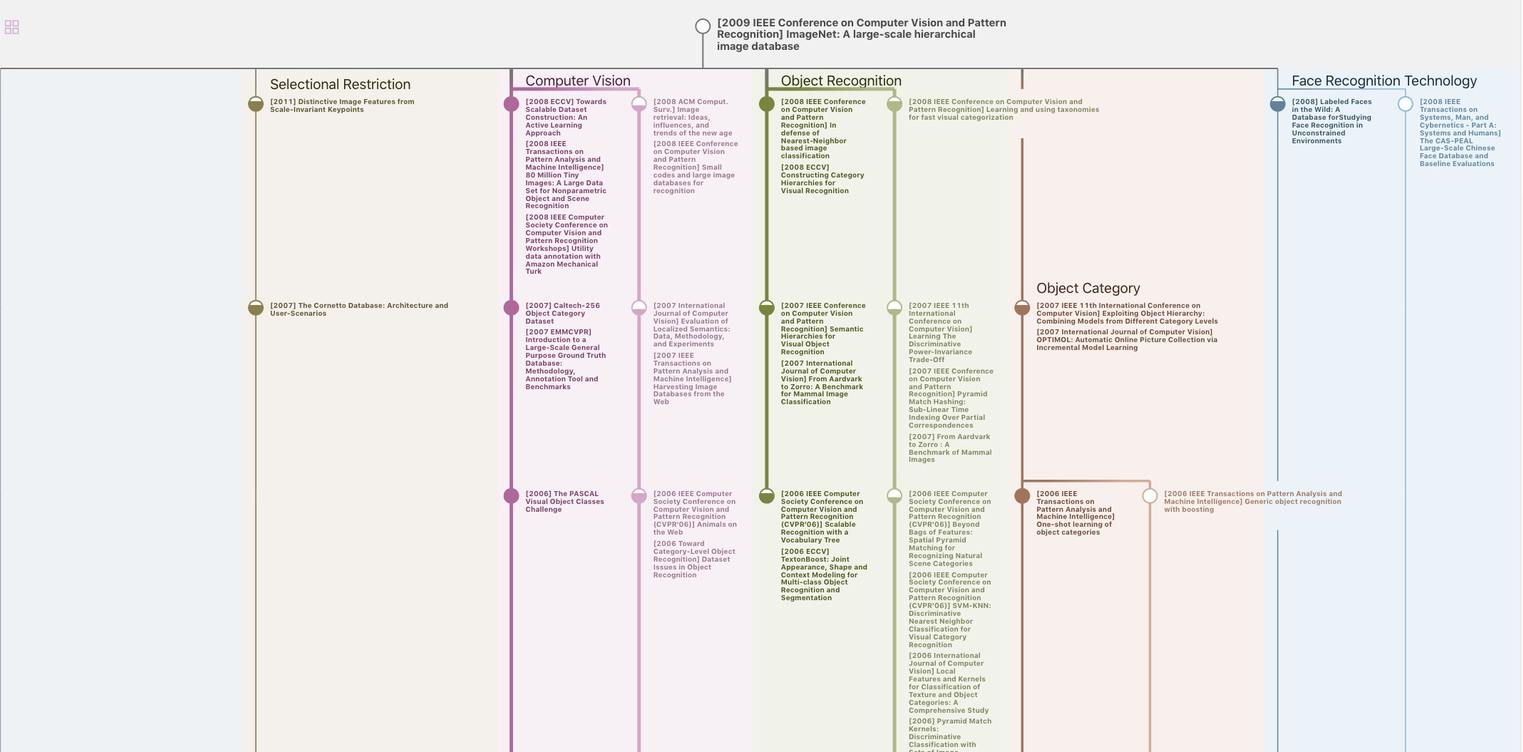
生成溯源树,研究论文发展脉络
Chat Paper
正在生成论文摘要