Conjugate Heat Transfer in High-Speed External Flows: A Review
Journal of Thermophysics and Heat Transfer(2023)
摘要
No AccessSurvey PapersConjugate Heat Transfer in High-Speed External Flows: A ReviewMikaela T. Lewis and Jean-Pierre HickeyMikaela T. Lewis https://orcid.org/0000-0002-8812-482XUniversity of Waterloo, Waterloo, Ontario, Canada*Ph.D. Student, Department of Mechanical and Mechatronics Engineering, N2L 3G1; (Corresponding Author).Search for more papers by this author and Jean-Pierre Hickey https://orcid.org/0000-0002-6944-3964University of Waterloo, Waterloo, Ontario, Canada†Associate Professor, Department of Mechanical and Mechatronics Engineering; also Waterloo Institute for Sustainable Aeronautics (WISA); .Search for more papers by this authorPublished Online:25 Jul 2023https://doi.org/10.2514/1.T6763SectionsRead Now ToolsAdd to favoritesDownload citationTrack citations ShareShare onFacebookTwitterLinked InRedditEmail About References [1] Errera M. P., Moretti R., Mayeur J., Gelain M., Tessé L., Lamet J. M. and Laroche E., “A Numerical Predictive Model for Conjugate Heat Transfer with Radiation,” International Journal of Heat and Mass Transfer, Vol. 160, Oct. 2020, Paper 120155. https://doi.org/10.1016/j.ijheatmasstransfer.2020.120155 Google Scholar[2] Qian K., Liu T., He F., Wang M., Tang L. and Zhou J., “Numerical Investigation on Radiation Effect in Transpiration Cooling,” Journal of Physics: Conference Series, Vol. 2097, No. 1, 2021, Paper 012011. https://doi.org/10.1088/1742-6596/2097/1/012011 Google Scholar[3] Huergo Perea D., “High-Order Methods for the CFD Simulations of Hypersonic Flows over a Reentry Capsule,” Master Thesis, Escuela Técnica Superior de Ingeniería Aeronáutica y del Espacio, Madrid, 2022. Google Scholar[4] Crocker R. C., “Direct Numerical Simulation of Ablative Boundaries in Turbulent and Laminar Flows,” Ph.D. Thesis, Univ. of Vermont, Burlington, VT, 2015, https://scholarworks.uvm.edu/graddis. Google Scholar[5] Mei Z., Shi C., Fan X. and Wang X., “Coupled Simulation for Reentry Ablative Behavior of Hypersonic Vehicles,” IOP Conference Series: Materials Science and Engineering, Vol. 892, No. 1, 2020, Paper 012028. https://doi.org/10.1088/1757-899X/892/1/012028 Google Scholar[6] Appar A., Kumar R. and Naspoori S. K., “Conjugate Flow-Thermal Analysis of a Hypersonic Reentry Vehicle in the Rarefied Flow Regime,” Physics of Fluids, Vol. 34, No. 2, 2022, Paper 026107. https://doi.org/10.1063/5.0082783 Google Scholar[7] Rom M. and Müller S., “Derivation and Analysis of a 1D Porous Medium Flow Solver Embedded in a Two-Domain Model for 2D and 3D Transpiration Cooling,” International Journal of Heat and Mass Transfer, Vol. 195, Oct. 2022, Paper 123127, https://www.sciencedirect.com/science/article/pii/S0017931022005981. https://doi.org/10.1016/j.ijheatmasstransfer.2022.123127 Google Scholar[8] Zhao X., Sun Z., Tang L. and Zheng G., “Coupled Flow-Thermal-Structural Analysis of Hypersonic Aerodynamically Heated Cylindrical Leading Edge,” Engineering Applications of Computational Fluid Mechanics, Vol. 5, No. 2, 2011, pp. 170–179. https://doi.org/10.1080/19942060.2011.11015361 CrossrefGoogle Scholar[9] Zhang S., Chen F. and Liu H., “Time-Adaptive, Loosely Coupled Strategy for Conjugate Heat Transfer Problems in Hypersonic Flows,” Journal of Thermophysics and Heat Transfer, Vol. 28, No. 4, 2014, pp. 635–646. https://doi.org/10.2514/1.T4278 LinkGoogle Scholar[10] Chen F., Liu H. and Zhang S., “Coupled Heat Transfer and Thermo-Mechanical Behavior of Hypersonic Cylindrical Leading Edges,” International Journal of Heat and Mass Transfer, Vol. 122, July 2018, pp. 846–862. https://doi.org/10.1016/j.ijheatmasstransfer.2018.02.037 CrossrefGoogle Scholar[11] Dorfman A. and Renner Z., “Conjugate Problems in Convective Heat Transfer: Review,” Mathematical Problems in Engineering, Vol. 2009, Oct. 2009, pp. 1–27. https://doi.org/10.1155/2009/927350 Google Scholar[12] Zhang K., Hickey J.-P. and Vlasea M., “An Analysis Framework of Additively Manufactured Deterministic Porous Structures for Transpiration Cooling,” Journal of Materials Engineering and Performance (accepted for publication). Google Scholar[13] Kuntz D. W., Hassan B. and Potter D. L., “Predictions of Ablating Hypersonic Vehicles Using an Iterative Coupled Fluid/Thermal Approach,” Journal of Thermophysics and Heat Transfer, Vol. 15, No. 2, 2001, pp. 129–139. https://doi.org/10.2514/2.6594 LinkGoogle Scholar[14] Dorfman A. S., Conjugate Problems in Convective Heat Transfer, CRC Press, Taylor & Francis Group, London, Parts 1 and 2, 2010. Google Scholar[15] John B., Senthilkumar P. and Sadasivan S., “Applied and Theoretical Aspects of Conjugate Heat Transfer Analysis: A Review,” Archives of Computational Methods in Engineering, Vol. 26, No. 2, 2019, pp. 475–489. https://doi.org/10.1007/s11831-018-9252-9 CrossrefGoogle Scholar[16] Yang X., Xiao G., Du Y., Liu L., Wei D. and Gui Y., “Heat Transfer with Interface Effects in High-Enthalpy and High-Speed Flow: Modelling Review and Recent Progress,” Applied Thermal Engineering, Vol. 195, Aug. 2021, Paper 116721. https://doi.org/10.1016/j.applthermaleng.2021.116721 Google Scholar[17] McNamara J. J. and Friedmann P. P., “Aeroelastic and Aerothermoelastic Analysis in Hypersonic Flow: Past, Present, and Future,” AIAA Journal, Vol. 49, No. 6, 2011, pp. 1089–1122. https://doi.org/10.2514/1.J050882 LinkGoogle Scholar[18] Griffin K. P., Fu L. and Moin P., “Velocity Transformation for Compressible Wall-Bounded Turbulent Flows with and Without Heat Transfer,” Proceedings of the National Academy of Sciences, Vol. 118, No. 34, 2021, Paper e2111144118. https://doi.org/10.1073/pnas.2111144118 Google Scholar[19] Younes K. and Hickey J.-P., “Mean Velocity Scaling of High-Speed Turbulent Flows Under Nonadiabatic Wall Conditions,” AIAA Journal, Vol. 61, No. 4, 2023, pp. 1532–1539. https://doi.org/10.2514/1.J062547 LinkGoogle Scholar[20] John B., Kusuma Chandrashekhara S. and Panneerselvam V., “Conjugate Heat Transfer Study of Hypersonic Flow Past a Cylindrical Leading Edge Composed of Functionally Graded Materials,” Proceedings of the Institution of Mechanical Engineers, Part C: Journal of Mechanical Engineering Science, Vol. 236, No. 8, 2022, pp. 4394–4411. https://doi.org/10.1177/09544062211048179 CrossrefGoogle Scholar[21] Naved I., Hermann T. and McGilvray M., “Numerical Simulation of Transpiration Cooling for a High-Speed Vehicle with Substructure,” AIAA Journal, Vol. 59, No. 8, 2021, pp. 3043–3053. https://doi.org/10.2514/1.J059771 AbstractGoogle Scholar[22] Tekure V. and Venkatasubbaiah K., “Effect of Mach Number and Plate Thickness on the Flow Field and Heat Transfer Characteristics of Supersonic Turbulent Flow over a Flat Plate at Different Thermal Boundary Conditions,” European Journal of Mechanics, B/Fluids, Vol. 88, July 2021, pp. 160–177. https://doi.org/10.1016/j.euromechflu.2021.04.002 CrossrefGoogle Scholar[23] Wang R., Wang Z., Zheng H. and Song H., “Comparison of Strategies for Coupled Flow-Thermal Analysis of Thermal Protection System at Hypersonic Flight Condition,” International Journal of Aeronautical and Space Sciences, Vol. 21, No. 2, 2020, pp. 347–362. https://doi.org/10.1007/s42405-019-00217-x CrossrefGoogle Scholar[24] De Marinis D., Bonelli F. and Pascazio G., “An Immersed Boundary Approach for Hypersonic Flows,” Italian Association of Aeronautics and Astronautics, XXV International Congress, Associazione Italiana di Aeronautica e Astronautica, 2019, pp. 1–8, https://www.researchgate.net/publication/343820521. Google Scholar[25] Reinert J. D., Dwivedi A. and Candler G. V., “Verification of a Conjugate Heat Transfer Tool with US3D,” AIAA Scitech 2019 Forum, AIAA Paper 2019-1892, 2019. https://doi.org/10.2514/6.2019-1892 LinkGoogle Scholar[26] Sahoo N., Kulkarni V. and Peetala R. K., “Conjugate Heat Transfer Study in Hypersonic Flows,” Journal of the Institution of Engineers (India): Series C, Vol. 99, No. 2, 2018, pp. 151–158. https://doi.org/10.1007/s40032-017-0353-2 CrossrefGoogle Scholar[27] Zhao X., Meganathan A. and Zhang S., “Computational Approach for Aeroheating with Thermally Coupled Fields,” Journal of Thermophysics and Heat Transfer, Vol. 31, No. 3, 2017, pp. 489–499. https://doi.org/10.2514/1.T4633 LinkGoogle Scholar[28] Veeraragavan A., Beri J. and Gollan R. J., “Use of the Method of Manufactured Solutions for the Verification of Conjugate Heat Transfer Solvers,” Journal of Computational Physics, Vol. 307, Feb. 2016, pp. 308–320. https://doi.org/10.1016/j.jcp.2015.12.004 CrossrefGoogle Scholar[29] Miller B. A., “Loosely Coupled Time Integration of Fluid-Thermal-Structural Interactions in Hypersonic Flows,” Ph.D. Thesis, Ohio State Univ., Columbus, OH, 2015. Google Scholar[30] Peetala R. K., “Conjugate Heat Transfer Analysis in Hypersonic Applications,” Ph.D. Thesis, Indian Inst. of Technology, Guwahati, India, 2014. Google Scholar[31] Chandra Murty M., Manna P. and Chakraborty D., “Conjugate Heat Transfer Analysis in High Speed Flows,” Proceedings of the Institution of Mechanical Engineers, Part G: Journal of Aerospace Engineering, Vol. 227, No. 10, 2013, pp. 1672–1681. https://doi.org/10.1177/0954410012464920 Google Scholar[32] Hosters N., Klaus M., Behr M. and Reimerdes H. G., “Application of a Partitioned Field Approach to Transient Aerothermal Problems in Rocket Nozzles,” Computers & Fluids, Vol. 88, Dec. 2013, pp. 795–803. https://doi.org/10.1016/j.compfluid.2013.06.014 CrossrefGoogle Scholar[33] Oppattaiyamath G., Reddy N., Jammy S. and Kulkarni V., “Conjugate Heat-Transfer Analysis for Hypersonic Flow over Finite Thickness Flat Plate,” Journal of Aerospace Engineering, Vol. 26, No. 4, 2013, pp. 708–714. CrossrefGoogle Scholar[34] Crowell A. R., Miller B. A. and McNamara J. J., “Computational Modeling for Conjugate Heat Transfer of Shock-Surface Interactions on Compliant Skin Panels,” Collection of Technical Papers—AIAA/ASME/ASCE/AHS/ASC Structures, Structural Dynamics and Materials Conference, AIAA Paper 2011-2017, 2011. https://doi.org/10.2514/6.2011-2017 Google Scholar[35] Ferrero P. and D’Ambrosio D., “A Numerical Method for Conjugate Heat Transfer Problems in Hypersonic Flows,” 40th AIAA Thermophysics Conference, AIAA Paper 2008-4247, 2008. https://doi.org/10.2514/6.2008-4247 LinkGoogle Scholar[36] Rahaim C. P., Kassab A. J. and Cavalleri R. J., “Coupled Dual Reciprocity Boundary Element/Finite Volume Method for Transient Conjugate Heat Transfer,” Journal of Thermophysics and Heat Transfer, Vol. 14, No. 1, 2000, pp. 27–38. https://doi.org/10.2514/2.6506 LinkGoogle Scholar[37] Hassan B., Kuntz D. and Potter D., “Coupled Fluid/Thermal Prediction of Ablating Hypersonic Vehicles,” 36th AIAA Aerospace Sciences Meeting and Exhibit, AIAA Paper 1998-168, Jan. 1998. Google Scholar[38] Kontinos D., “Coupled Thermal Analysis Method with Application to Metallic Thermal Protection Panels,” Journal of Thermophysics and Heat Transfer, Vol. 11, No. 2, 1997, pp. 173–181. https://doi.org/10.2514/2.6249 LinkGoogle Scholar[39] Salem R., Errera M. and Marty J., “Adaptive Diffusive Time-Step in Conjugate Heat Transfer Interface Conditions for Thermal-Barrier-Coated Applications,” International Journal of Thermal Sciences, Vol. 145, Nov. 2019, Paper 106048. https://doi.org/10.1016/j.ijthermalsci.2019.106048 Google Scholar[40] Wang J., Wang M. and Li Z., “A Lattice Boltzmann Algorithm for Fluid-Solid Conjugate Heat Transfer,” International Journal of Thermal Sciences, Vol. 46, No. 3, 2007, pp. 228–234, https://www.sciencedirect.com/science/article/pii/S129007290600072X. https://doi.org/10.1016/j.ijthermalsci.2006.04.012 CrossrefGoogle Scholar[41] Tarokh A., Mohamad A. A. and Jiang L., “Simulation of Conjugate Heat Transfer Using the Lattice Boltzmann Method,” Numerical Heat Transfer, Part A: Applications, Vol. 63, No. 3, 2013, pp. 159–178. https://doi.org/10.1080/10407782.2012.725009 CrossrefGoogle Scholar[42] Li J., Wang J., Yang L. and Shu C., “A Hybrid Lattice Boltzmann Flux Solver for Integrated Hypersonic Fluid-Thermal-Structural Analysis,” Chinese Journal of Aeronautics, Vol. 33, No. 9, 2020, p. 2295–2312. https://doi.org/10.1016/j.cja.2020.04.020 CrossrefGoogle Scholar[43] Bertin J. J. and Cummings R. M., “Critical Hypersonic Aerothermodynamic Phenomena,” Annual Review of Fluid Mechanics, Vol. 38, Jan. 2006, pp. 129–157. https://doi.org/10.1146/annurev.fluid.38.050304.092041 CrossrefGoogle Scholar[44] Marvin J. G., “Turbulence Modeling for Hypersonic Flows,” NASA TM-101079, 1989. Google Scholar[45] Favre A., “Turbulence: Space-Time Statistical Properties and Behavior in Supersonic Flows,” Physics of Fluids, Vol. 26, No. 10, 1983, pp. 2851–2863. https://doi.org/10.1063/1.864049 CrossrefGoogle Scholar[46] Roy C. J. and Blottner F. G., “Review and Assessment of Turbulence Models for Hypersonic Flows,” Progress in Aerospace Sciences, Vol. 42, Nos. 7–8, 2006, pp. 469–530. https://doi.org/10.1016/j.paerosci.2006.12.002 CrossrefGoogle Scholar[47] Syed I., “Modelling Aerothermal Heating with Conjugate Heat Transfer,” Master’s Thesis, Univ. of Waterloo, Waterloo, Ontario, Canada, 2022. Google Scholar[48] Papalexandris M. V., “On the Applicability of Stokes’Hypothesis to Low-Mach-Number Flows,” Continuum Mechanics and Thermodynamics, Vol. 32, No. 4, 2020, pp. 1245–1249. https://doi.org/10.1007/s00161-019-00785-z CrossrefGoogle Scholar[49] Huang P. G., “Compressible Turbulent Channel Flows: DNS Results and Modelling,” Journal of Fluid Mechanics, Vol. 305, Dec. 1995, pp. 185–218. https://doi.org/10.1017/S0022112095004599 CrossrefGoogle Scholar[50] Pope S. B., Turbulent Flows, 1st ed., Cambridge Univ. Press, Cambridge, England, U.K., 2000, pp. 358–386. CrossrefGoogle Scholar[51] Fu L., Karp M., Bose S., Moin P. and Urzay J., “Equilibrium Wall-Modeled LES of Shock-Induced Aerodynamic Heating in Hypersonic Boundary Layers,” Center for Turbulence Research Annual Research Briefs, Vol. 2018, Nov. 2018, pp. 171–181. Google Scholar[52] Tauber M. E., “A Review of High-Speed, Convective, Heat-Transfer Computation Methods,” NASA, Office of Management TR 2914, 1989. Google Scholar[53] van Driest E. R., “Turbulent Boundary Layer in Compressible Fluids,” Journal of Aeronautical Sciences, Vol. 18, No. 3, 1951, pp. 145–160. https://doi.org/10.2514/2.7048 LinkGoogle Scholar[54] Trettel A. and Larsson J., “Mean Velocity Scaling for Compressible Wall Turbulence with Heat Transfer,” Physics of Fluids, Vol. 28, No. 2, 2016, Paper 026102. https://doi.org/10.1063/1.4942022 CrossrefGoogle Scholar[55] Pecnik R. and Patel A., “Scaling and Modelaling of Turbulence in Variable Property Channel Flows,” Journal of Fluid Mechanics, Vol. 823, July 2017, p. R1. https://doi.org/10.1017/jfm.2017.348 Google Scholar[56] Bai T., Griffin K. P. and Fu L., “Compressible Velocity Transformations for Various Noncanonical Wall-Bounded Turbulent Flows,” AIAA Journal, Vol. 60, No. 7, 2022, pp. 4325–4337. https://doi.org/10.2514/1.J061554 LinkGoogle Scholar[57] Kader B. A., “Temperature and Concentration Profiles in Fully Turbulent Boundary Layers,” International Journal of Heat and Mass Transfer, Vol. 24, No. 9, 1981, pp. 1541–1544. https://doi.org/10.1016/0017-9310(81)90220-9 CrossrefGoogle Scholar[58] Guo J., Yang X. I. and Ihme M., “Structure of the Thermal Boundary Layer in Turbulent Channel Flows at Transcritical Conditions,” Journal of Fluid Mechanics, Vol. 934, March 2022, p. A45. https://doi.org/10.1017/jfm.2021.1157 Google Scholar[59] Pouransari H. and Mani A., “Particle-to-Fluid Heat Transfer in Particle-Laden Turbulence,” Physical Review Fluids, Vol. 3, No. 7, 2018, Paper 074304. https://doi.org/10.1103/PhysRevFluids.3.074304 Google Scholar[60] Mouallem J. and Hickey J.-P., “Induction Heating of Dispersed Metallic Particles in a Turbulent Flow,” International Journal of Multiphase Flow, Vol. 132, Nov. 2020, Paper 103414, https://www.sciencedirect.com/science/article/pii/S0301932220305231. https://doi.org/10.1016/j.ijmultiphaseflow.2020.103414 Google Scholar[61] Saieed A., Rahman M. M. and Hickey J.-P., “Role of Viscosity in the Preferential Concentration of Heated, Bidispersed Particles,” International Journal of Multiphase Flow, Vol. 155, Oct. 2022, Paper 104185, https://www.sciencedirect.com/science/article/pii/S0301932222001690. https://doi.org/10.1016/j.ijmultiphaseflow.2022.104185 Google Scholar[62] Mullenix N. and Gaitonde D., “Development of a Conjugate Heat Transfer Simulation Tool for Anisotropic Thermal Protection Systems,” 51st AIAA Aerospace Sciences Meeting Including the New Horizons Forum and Aerospace Exposition, AIAA Paper 2013-0602, 2013. https://doi.org/10.2514/6.2013-602 LinkGoogle Scholar[63] Bryant C. E. and Rutledge J. L., “Conjugate Heat Transfer Simulations to Evaluate the Effect of Anisotropic Thermal Conductivity on Overall Cooling Effectiveness,” Journal of Thermal Science and Engineering Applications, Vol. 13, No. 6, 2021, Paper 061013. https://doi.org/10.1115/1.4050328 Google Scholar[64] Zinchenko V. I., Gol’din V. D. and Zverev V. G., “Numerical Modeling of the Influence of Thermal Protection Materials on Characteristics of Conjugate Heat and Mass Transfer with Spatial Flow Around Blunted Bodies,” High Temperature, Vol. 56, No. 5, 2018, pp. 719–726. https://doi.org/10.1134/S0018151X18040223 CrossrefGoogle Scholar[65] Desrayaud G., Fichera A. and Lauriat G., “Natural Convection Air-Cooling of a Substrate-Mounted Protruding Heat Source in a Stack of Parallel Boards,” International Journal of Heat and Fluid Flow, Vol. 28, No. 3, 2007, pp. 469–482. https://doi.org/10.1016/j.ijheatfluidflow.2006.07.003 Google Scholar[66] Hachem E., Ghraieb H., Viquerat J., Larcher A. and Meliga P., “Deep Reinforcement Learning for the Control of Conjugate Heat Transfer,” Journal of Computational Physics, Vol. 436, July 2021, Paper 110317. https://doi.org/10.1016/j.jcp.2021.110317 Google Scholar[67] Errera M. and El Khoury R., “Numerical Study of Two Optimized Coupling Interface Treatments for Steady Conjugate Heat Transfer Problems,” VII European Congress on Computational Methods in Applied Sciences and Engineering, European Community on Computational Methods in Applied Sciences, 2016, pp. 1–14, https://hal.archives-ouvertes.fr/hal-01414827. Google Scholar[68] Giles M. B., “Nonreflecting Boundary Conditions for Euler Equation Calculations,” AIAA Journal, Vol. 28, No. 12, 1990, pp. 2050–2058. https://doi.org/10.2514/3.10521 LinkGoogle Scholar[69] Poinsot T. J. and Lelef S. K., “Boundary Conditions for Direct Simulations of Compressible Viscous Flows,” Journal of Computational Physics, Vol. 101, No. 1, 1992, pp. 104–129. https://doi.org/10.1016/0021-9991(92)90046-2 CrossrefGoogle Scholar[70] Varanasi C., Murthy J. Y. and Mathur S., “A Meshless Finite Difference Method for Conjugate Heat Conduction Problems,” Journal of Heat Transfer, Vol. 132, No. 8, 2010, pp. 1–13. https://doi.org/10.1115/1.4001363 Google Scholar[71] Farhat C. and Lesoinne M., “Two Efficient Staggered Algorithms for the Serial and Parallel Solution of Three-Dimensional Nonlinear Transient Aeroelastic Problems,” Computer Methods in Applied Mechanics and Engineering, Vol. 182, Nos. 3–4, 2000, pp. 499–515. https://doi.org/10.1016/S0045-7825(99)00206-6 CrossrefGoogle Scholar[72] Giles M. B., “Stability Analysis of Numerical Interface Conditions in Fluid-Structure Thermal Analysis,” International Journal for Numerical Methods in Fluids, Vol. 25, No. 4, 1997, pp. 421–436. https://doi.org/10.1002/(SICI)1097-0363(19970830)25:4<421::AID-FLD557>3.0.CO;2-J CrossrefGoogle Scholar[73] Kazemi Kamyab V., “High Order Time-Accurate Partitioned Simulation of Unsteady Conjugate Heat Transfer; Analysis and Application of Implicit Runge-Kutta Time Integration Schemes,” Ph.D. Thesis, TU-Delft, Delft, The Netherlands, 2013. Google Scholar[74] Liu Q., “Coupling Heat Transfer and Fluid Flow Solvers for Multidisciplinary Simulations,” Ph.D. Thesis, Mississippi State Univ., Starkville, MS, 2003. Google Scholar[75] Keyes D. E., McInnes L. C., Woodward C., Gropp W., Myra E., Pernice M., Bell J., Brown J., Clo A., Connors J., Constantinescu E., Estep D., Evans K., Farhat C., Hakim A., Hammond G., Hansen G., Hill J., Isaac T., Jiao X., Jordan K., Kaushik D., Kaxiras E., Koniges A., Lee K., Lott A., Lu Q., Magerlein J., Maxwell R., McCourt M., Mehl M., Pawlowski R., Randles A. P., Reynolds D., Riviere B., Rude U., Scheibe T., Shadid J., Sheehan B., Shephard M., Siegel A., Smith B., Tang X., Wilson C. and Wohlmuth B., “Multiphysics Simulations: Challenges and Opportunities,” International Journal of High Performance Computing Applications, Vol. 27, No. 1, 2013, pp. 4–83. https://doi.org/10.1177/1094342012468181 CrossrefGoogle Scholar[76] Matthies H. G. and Steindorf J., “Partitioned but Strongly Coupled Iteration Schemes for Nonlinear Fluid-Structure Interaction,” Computers & Structures, Vol. 80, Nos. 27–30, 2002, pp. 1991–1999. https://doi.org/10.1016/S0045-7949(02)00259-6 CrossrefGoogle Scholar[77] Meng F., Banks J. W., Henshaw W. D. and Schwendeman D. W., “A Stable and Accurate Partitioned Algorithm for Conjugate Heat Transfer,” Journal of Computational Physics, Vol. 344, Sept. 2017, pp. 51–85. https://doi.org/10.1016/j.jcp.2017.04.052 Google Scholar[78] Han Z.-X., Dennis B. H. and Dulikravich G. S., “Simultaneous Prediction of External Flow-Field and Temperature in Internally Cooled 3-d Turbine Blade Material,” ASME TURBOEXPO, American Soc. of Mechanical Engineers, Fairfield, NJ, 2000, http://asmedigitalcollection.asme.org/GT/proceedings-pdf/GT2000/78569/V003T01A059/2413909/v003t01a059-2000-gt-0253.pdf. Google Scholar[79] Rahman F., Visser J. A. and Morris R. M., “Capturing Sudden Increase in Heat Transfer on the Suction Side of a Turbine Blade Using a Navier-Stokes Solver,” Journal of Turbomachinery, Vol. 127, No. 3, 2005, pp. 552–556. https://doi.org/10.1115/1.1928287 Google Scholar[80] Meng Z. X., Fan H., Peng K., Zhang W. H. and Yang H. X., “A Hypersonic Aeroheating Calculation Method Based on Inviscid Outer Edge of Boundary Layer Parameters,” Acta Astronautica, Vol. 129, Dec. 2016, pp. 429–437. https://doi.org/10.1016/j.actaastro.2016.08.039 CrossrefGoogle Scholar[81] Feodoritova O. B., Krasnov M. M. and Zhukov V. T., “A Numerical Method for Conjugate Heat Transfer Problems in Multicomponent Flows,” Journal of Physics: Conference Series, Vol. 2028, No. 1, 2021, Paper 012024. https://doi.org/10.1088/1742-6596/2028/1/012024 Google Scholar[82] He M., Bishop P. J., Kassab A. J. and Minardi A., “A Coupled FDM/BEM Solution for the Conjugate Heat Transfer Problem,” Numerical Heat Transfer, Part B: Fundamentals, Vol. 28, No. 2, 1995, pp. 139–154. https://doi.org/10.1080/10407799508928826 CrossrefGoogle Scholar[83] Nagendra K., Tafti D. K. and Viswanath K., “A New Approach for Conjugate Heat Transfer Problems Using Immersed Boundary Method for Curvilinear Grid Based Solvers,” Journal of Computational Physics, Vol. 267, June 2014, pp. 225–246. https://doi.org/10.1016/j.jcp.2014.02.045 CrossrefGoogle Scholar[84] Sun H. and Darmofal D. L., “An Adaptive Simplex Cut-Cell Method for High-Order Discontinuous Galerkin Discretizations of Elliptic Interface Problems and Conjugate Heat Transfer Problems,” Journal of Computational Physics, Vol. 278, Dec. 2014, pp. 445–468. https://doi.org/10.1016/j.jcp.2014.08.035 CrossrefGoogle Scholar[85] De Marinis D., De Tullio M. D., Napolitano M. and Pascazio G., “A Conjugate-Heat-Transfer Immersed-Boundary Method for Turbine Cooling,” Energy Procedia, Vol. 82, Dec. 2015, pp. 215–221. https://doi.org/10.1016/j.egypro.2015.12.025 Google Scholar[86] Das S., Deen N. G. and Kuipers J. A., “Direct Numerical Simulation for Flow and Heat Transfer through Random Open-Cell Solid Foams: Development of an IBM Based CFD Model,” Catalysis Today, Vol. 273, Sept. 2016, pp. 140–150. https://doi.org/10.1016/j.cattod.2016.03.048 Google Scholar[87] Hötte F. and Haupt M. C., “Transient 3D Conjugate Heat Transfer Simulation of a Rectangular GOX-GCH4 Rocket Combustion Chamber and Validation,” Aerospace Science and Technology, Vol. 105, Oct. 2020, Paper 106043. https://doi.org/10.1016/j.ast.2020.106043 Google Scholar[88] Nasuti F., Torricelli A. and Pirozzoli S., “Conjugate Heat Transfer Analysis of Rectangular Cooling Channels Using Modeled and Direct Numerical Simulation of Turbulence,” International Journal of Heat and Mass Transfer, Vol. 181, Dec. 2021, Paper 121849. https://doi.org/10.1016/j.ijheatmasstransfer.2021.121849 Google Scholar[89] Yang G., “Modelling of Turbulent Conjugate Heat Transfer,” Ph.D. Thesis, Univ. of Manchester, Manchester, England, U.K., 2020. Google Scholar[90] Wang P., Li Y., Zou Z. and Zhang W., “Conjugate Heat Transfer Investigation of Cooled Turbine Using the Preconditioned Density-Based Algorithm,” Propulsion and Power Research, Vol. 2, No. 1, 2013, pp. 56–69. https://doi.org/10.1016/j.jppr.2012.10.004 CrossrefGoogle Scholar[91] Wang P., Li Y., Zou Z., Wang L. and Song S., “Influence of Turbulence Model Parameter Settings on Conjugate Heat Transfer Simulation,” Heat and Mass Transfer/Waerme- und Stoffuebertragung, Vol. 50, No. 4, 2014, pp. 521–532. https://doi.org/10.1007/s00231-013-1253-5 CrossrefGoogle Scholar[92] Zhang C., Duan L. and Choudhari M. M., “Direct Numerical Simulation Database for Supersonic and Hypersonic Turbulent Boundary Layers,” AIAA Journal, Vol. 56, No. 11, 2018, pp. 4297–4311. https://doi.org/10.2514/1.J057296 LinkGoogle Scholar[93] Tang H. and Gao Z., “Investigation of Turbulence Models with Compressibility Corrections for Hypersonic Boundary Flows,” Advances in Mechanical Engineering, Vol. 7, No. 12, 2015, Paper 1687814015620319. https://doi.org/10.1177/1687814015620319 Google Scholar[94] Yoder D. A., “Comparison of Turbulent Thermal Diffusivity and Scalar Variance Models,” 54th AIAA Aerospace Sciences Meeting, AIAA Paper 2016-1561, 2016, pp. 1–22. Google Scholar[95] Syed I., Hickey J.-P., Godbolt B. and Farnfield E., “Error Quantification among CFD Solvers for High-Speed, Non-Adiabatic, Wall-Bounded Turbulent Flows,” AIAA Scitech 2021 Forum, AIAA Paper 2021-1842, 2021. https://doi.org/10.2514/6.2021-1842 Google Scholar[96] Roy S. and Sinha K., “Turbulent Heat Flux Model for Hypersonic Shock-Boundary Layer Interaction,” AIAA Journal, Vol. 57, No. 8, 2019, pp. 3624–3629. https://doi.org/10.2514/1.J058334 LinkGoogle Scholar[97] Bukva A., Zhang K., Christopher N. and Hickey J. P., “Assessment of Turbulence Modeling for Massively-Cooled Turbulent Boundary Layer Flows with Transpiration Cooling,” Physics of Fluids, Vol. 33, No. 9, 2021, Paper 095114. https://doi.org/10.1063/5.0062155 Google Scholar[98] Wilcox D. C., Turbulence Modeling for CFD, DCW Industries, Canada, CA, 2006, Chaps. 5 and 6. Google Scholar[99] Indelicato G., Lapenna P. E., Remiddi A. and Creta F., “An Efficient Modeling Framework for Wall Heat Flux Prediction in Rocket Combustion Chambers Using Non Adiabatic Flamelets and Wall-Functions,” International Journal of Heat and Mass Transfer, Vol. 169, April 2021, Paper 120913. https://doi.org/10.1016/j.ijheatmasstransfer.2021.120913 Google Scholar[100] Ma P. C., Wu H., Ihme M. and Hickey J.-P., “Nonadiabatic Flamelet Formulation for Predicting Wall Heat Transfer in Rocket Engines,” AIAA Journal, Vol. 56, No. 6, 2018, pp. 1–14. https://doi.org/10.2514/1.J056539 Google Scholar[101] Gelain M., “Aerothermal Characterisation of a Surface Heat Exchanger Implemented in a Turbofan By-Pass Duct,” Ph.D. Thesis, Université Paris-Saclay, Gif-sur-Yvette, France, 2021, https://tel.archives-ouvertes.fr/tel-03556926. Google Scholar[102] Li Y., Ries F., Nishad K. and Sadiki A., “Predictions of Conjugate Heat Transfer in Turbulent Channel Flow Using Advanced Wall-Modeled Large Eddy Simulation Techniques,” Entropy, Vol. 23, No. 6, 2021, p. 725. https://doi.org/10.3390/e23060725 CrossrefGoogle Scholar[103] Flageul C., Benhamadouche S., Lamballais E. and Laurence D., “DNS of Turbulent Channel Flow with Conjugate Heat Transfer: Effect of Thermal Boundary Conditions on the Second Moments and Budgets,” International Journal of Heat and Fluid Flow, Vol. 55, Oct. 2015, pp. 34–44. https://doi.org/10.1016/j.ijheatfluidflow.2015.07.009 CrossrefGoogle Scholar[104] Wu Z., Laurence D., Iacovides H. and Afgan I., “Direct Simulation of Conjugate Heat Transfer of Jet in Channel Crossflow,” International Journal of Heat and Mass Transfer, Vol. 110, July 2017, pp. 193–208. https://doi.org/10.1016/j.ijheatmasstransfer.2017.03.027 Google Scholar[105] Kuhn S., Braillard O., Ničeno B. and Prasser H. M., “Computational Study of Conjugate Heat Transfer in T-Junctions,” Nuclear Engineering and Design, Vol. 240, No. 6, 2010, pp. 1548–1557. https://doi.org/10.1016/j.nucengdes.2010.02.022 Google Scholar[106] Dahlberg M., Nilsson K.-F., Taylor N. and Faidy C., “Development of a European Procedure for Assessment of High Cycle Thermal Fatigue in Light Water Reactors: Final Report of the NESC-Thermal Fatigue Project 2007,” European Commission Directorate-General Joint Research Centre Inst. for Energy TR EUR 22763 EN, Petten, The Netherlands, 2007, http://www.jrc.ec.europa.eu/. Google Scholar[107] Duan L., Choudhari M. M. and Zhang C., “Pressure Fluctuations Induced by a Hypersonic Turbulent Boundary Layer,” Journal of Fluid Mechanics, Vol. 804, Oct. 2016, pp. 578–607. https://doi.org/10.1017/jfm.2016.548 CrossrefGoogle Scholar[108] Hillcoat S. and Hickey J.-P., “Pressure Fluctuations Under a Turbulent Boundary Layer with Transpiration Cooling,” AIAA Aviation 2021 Forum, AIAA Paper 2021-2605, 2021. https://doi.org/10.2514/6.2021-2605 LinkGoogle Scholar[109] Yang Z., Wang S. and Gao Z., “Studies on Effects of Wall Temperature Variation on Heat Transfer in Hypersonic Laminar Boundary Layer,” International Journal of Heat and Mass Transfer, Vol. 190, July 2022, Paper 122790. https://doi.org/10.1016/j.ijheatmasstransfer.2022.122790 Google Scholar[110] Oddo R., Hill J. L., Reeder M. F., Chin D., Embrador J., Komives J., Tufts M., Borg M. and Jewell J. S., “Effect of Surface Cooling on Second-Mode Dominated Hypersonic Boundary Layer Transition,” Experiments in Fluids, Vol. 62, No. 7, 2021, pp. 1–18. https://doi.org/10.1007/s00348-021-03237-0 CrossrefGoogle Scholar[111] Zhu Y., Zhu W., Gu D., Lee C. and Smith C. R., “Transitional Flow Structures in Heated Hypersonic Boundary Layers,” Physics of Fluids, Vol. 34, No. 5, 2022, Paper 054114. https://doi.org/10.1063/5.0091192 Google Scholar[112] Lysenko V. I. and Maslov A. A., “The Effect of Cooling on Supersonic Boundary-Layer Stability,” Journal of Fluid Mechanics, Vol. 147, Oct. 1984, pp. 39–52. https://doi.org/10.1017/S002211208400197X CrossrefGoogle Scholar[113] Fedorov A., “Transition and Stability of High-Speed Boundary Layers,” Annual Review of Fluid Mechanics, Vol. 43, Jan. 2011, pp. 79–95. https://doi.org/10.1146/annurev-fluid-122109-160750 CrossrefGoogle Scholar[114] Patel A., Boersma B. J. and Pecnik R., “The Influence of Near-Wall Density and Viscosity Gradients on Turbulence in Channel Flows,” Journal of Fluid Mechanics, Vol. 809, Dec. 2016, pp. 793–820. https://doi.org/10.1017/jfm.2016.689 CrossrefGoogle Scholar[115] Wagner A., Schramm J. M., Hannemann K., Whitside R. and Hickey J.-P., “Hypersonic Shock Wave Boundary Layer Interaction Studies on a Flat Plate at Elevated Surface Temperature,” Shock Wave Interactions, RaiNew 2017, edited by Kontis K., Springer, Cham, 2018. https://doi.org/10.1007/978-3-319-73180-3_19 Google Scholar[116] Tekure V. and Venkatasubbaiah K., “A New Correlation of Average Temperature and Maximum Heat Flux for Turbulent Supersonic Flow in a Large Size Channel up to Mach 5,” Aerospace Science and Technology, Vol. 96, Jan. 2020, Paper 105522. https://doi.org/10.1016/j.ast.2019.105522 Google Scholar[117] Aziz A. and Lopez R. J., “Convection-Radiation from a Continuously Moving, Variable Thermal Conductivity Sheet or Rod Undergoing Thermal Processing,” International Journal of Thermal Sciences, Vol. 50, No. 8, 2011, pp. 1523–1531. https://doi.org/10.1016/j.ijthermalsci.2011.03.014 CrossrefGoogle Scholar[118] Dogan M., Sivrioglu M. and Yilmaz O., “Numerical Analysis of Natural Convection and Radiation Heat Transfer from Various Shaped Thin Fin-Arrays Placed on a Horizontal Plate—A Conjugate Analysis,” Energy Conversion and Management, Vol. 77, Jan. 2014, pp. 78–88. https://doi.org/10.1016/j.enconman.2013.09.021 Google Scholar[119] Duchaine F., Corpron A., Pons L., Moureau V., Nicoud F. and Poinsot T., “Development and Assessment of a Coupled Strategy for Conjugate Heat Transfer with Large Eddy Simulation: Application to a Cooled Turbine Blade,” International Journal of Heat and Fluid Flow, Vol. 30, No. 6, 2009, pp. 1129–1141. https://doi.org/10.1016/j.ijheatfluidflow.2009.07.004 CrossrefGoogle Scholar[120] Christopher N., Peter J. M., Kloker M. J. and Hickey J. P., “DNS of Turbulent Flat-Plate Flow with Transpiration Cooling,” International Journal of Heat and Mass Transfer, Vol. 157, Aug. 2020, Paper 119972. https://doi.org/10.1016/j.ijheatmasstransfer.2020.119972 Google Scholar[121] Jiang P. X., Yu L., Sun J. G. and Wang J., “Experimental and Numerical Investigation of Convection Heat Transfer in Transpiration Cooling,” Applied Thermal Engineering, Vol. 24, Nos. 8–9, 2004, pp. 1271–1289. https://doi.org/10.1016/j.applthermaleng.2003.12.023 CrossrefGoogle Scholar[122] Von Wolfersdorf J., “Effect of Coolant Side Heat Transfer on Transpiration Cooling,” Heat and Mass Transfer/Waerme- und Stoffuebertragung, Vol. 41, No. 4, 2005, pp. 327–337. https://doi.org/10.1007/s00231-004-0549-x CrossrefGoogle Scholar[123] Bai X., Yi Y., Liu C., Zhang W. and Nakayama A., “A Simple Conjugate Analysis and its Comparison with Experiment for Heat Transfer Problems Associated with Hot Gas Flows in a Partially Transpiration-Cooled Channel,” International Journal of Heat and Mass Transfer, Vol. 165, Feb. 2021, Paper 120729. https://doi.org/10.1016/j.ijheatmasstransfer.2020.120729 Google Scholar[124] Dahmen W., Gotzen T., Müller S. and Rom M., “Numerical Simulation of Transpiration Cooling Through Porous Material,” International Journal for Numerical Methods in Fluids, Vol. 76, No. 6, 2014, pp. 331–365. https://doi.org/10.1002/fld.3935 CrossrefGoogle Scholar[125] Shi J. X. and Wang J. H., “A Numerical Investigation of Transpiration Cooling with Liquid Coolant Phase Change,” Transport in Porous Media, Vol. 87, No. 3, 2011, pp. 703–716. https://doi.org/10.1007/s11242-010-9710-9 CrossrefGoogle Scholar[126] Efimov K. N., Ovchinnikov V. A. and Yakimov A. S., “Numerical Study of the Effect of Rotation on the Behavior of the Conjugate Heat and Mass Transfer on the Surface of a Spherically Blunted Cone Exposed to a Hypersonic Flow at an Angle of Attack with Ablation from the Surface,” High Temperature, Vol. 56, No. 2, 2018, pp. 239–246. https://doi.org/10.1134/S0018151X18020074 CrossrefGoogle Scholar[127] Crowell A. R., Miller B. A. and McNamara J. J., “Robust and Efficient Treatment of Temperature Feedback in Fluid-Thermal-Structural Analysis,” AIAA Journal, Vol. 52, No. 11, 2014, pp. 2395–2413. https://doi.org/10.2514/1.J052820 LinkGoogle Scholar[128] Dechaumphai P., Thornton E. A. and Wieting A. R., “Fluid-Thermal-Structural Study of Aerodynamically Heated Leading Edges,” Journal of Spacecraft and Rockets, Vol. 26, No. 4, 1989, pp. 201–209. https://doi.org/10.2514/3.26055 LinkGoogle Scholar[129] Wieting A. R., Dechaumphai P., Bey K. S., Thornton E. A. and Morgan K., “Application of Integrated Fluid-Thermal-Structural Analysis Methods,” Thin-Walled Structures, Vol. 11, Nos. 1–2, 1991, pp. 1–23. https://doi.org/10.1016/0263-8231(91)90008-7 CrossrefGoogle Scholar[130] Park C., Howe J. T., Jaffe R. L. and Candler G. V., “Review of Chemical-Kinetic Problems of Future NASA Missions. II—Mars Entries,” Journal of Thermophysics and Heat Transfer, Vol. 8, No. 1, 1994, pp. 9–23. https://doi.org/10.2514/3.496 LinkGoogle Scholar[131] Gnoffo P. A., “Planetary-Entry Gas Dynamics,” Annual Review of Fluid Mechanics, Vol. 31, No. 1, 1999, pp. 459–494. https://doi.org/10.1146/annurev.fluid.31.1.459 CrossrefGoogle Scholar[132] Yang J. and Liu M., “Numerical Analysis of Hypersonic Thermochemical Non-Equilibrium Environment for an Entry Configuration in Ionized Flow,” Chinese Journal of Aeronautics, Vol. 32, No. 12, 2019, pp. 2641–2654. https://doi.org/10.1016/j.cja.2019.06.004 CrossrefGoogle Scholar[133] Beran P., Stanford B. and Schrock C., “Uncertainty Quantification in Aeroelasticity,” Annual Review of Fluid Mechanics, Vol. 49, No. 1, 2017, pp. 361–386. https://doi.org/10.1146/annurev-fluid-122414-034441 CrossrefGoogle Scholar[134] Montomoli F., D’Ammaro A. and Uchida S., “Uncertainty Quantification and Conjugate Heat Transfer: A Stochastic Analysis,” Journal of Turbomachinery, Vol. 135, No. 3, 2013, Paper 031014. https://doi.org/10.1115/1.4007516 CrossrefGoogle Scholar Previous article Next article FiguresReferencesRelatedDetails What's Popular Articles in AdvanceSupplemental Materials CrossmarkInformationCopyright © 2023 by the American Institute of Aeronautics and Astronautics, Inc. All rights reserved. All requests for copying and permission to reprint should be submitted to CCC at www.copyright.com; employ the eISSN 1533-6808 to initiate your request. See also AIAA Rights and Permissions www.aiaa.org/randp. TopicsAerospace EngineeringAerospace SciencesBoundary LayersComputational Fluid DynamicsFluid DynamicsHeat ConductionHeat TransferThermal Control and ProtectionThermophysics and Heat TransferVortex Dynamics KeywordsDirichlet Boundary ConditionThermal Control and ProtectionAerospace EngineeringImproved Delayed Detached Eddy SimulationReynolds Averaged Navier StokesLaminar to Turbulent TransitionThermal DiffusivityConjugate Heat Transfer AnalysisTurbulent AeroheatingAcknowledgmentsMikaela T. Lewis would like to acknowledge the financial support from the Engineering Excellence Fellowship from the University of Waterloo and the Queen Elizabeth II Graduate Scholarship in Science and Technology.PDF Received18 October 2022Accepted30 May 2023Published online25 July 2023
更多查看译文
关键词
heat transfer,flows,high-speed
AI 理解论文
溯源树
样例
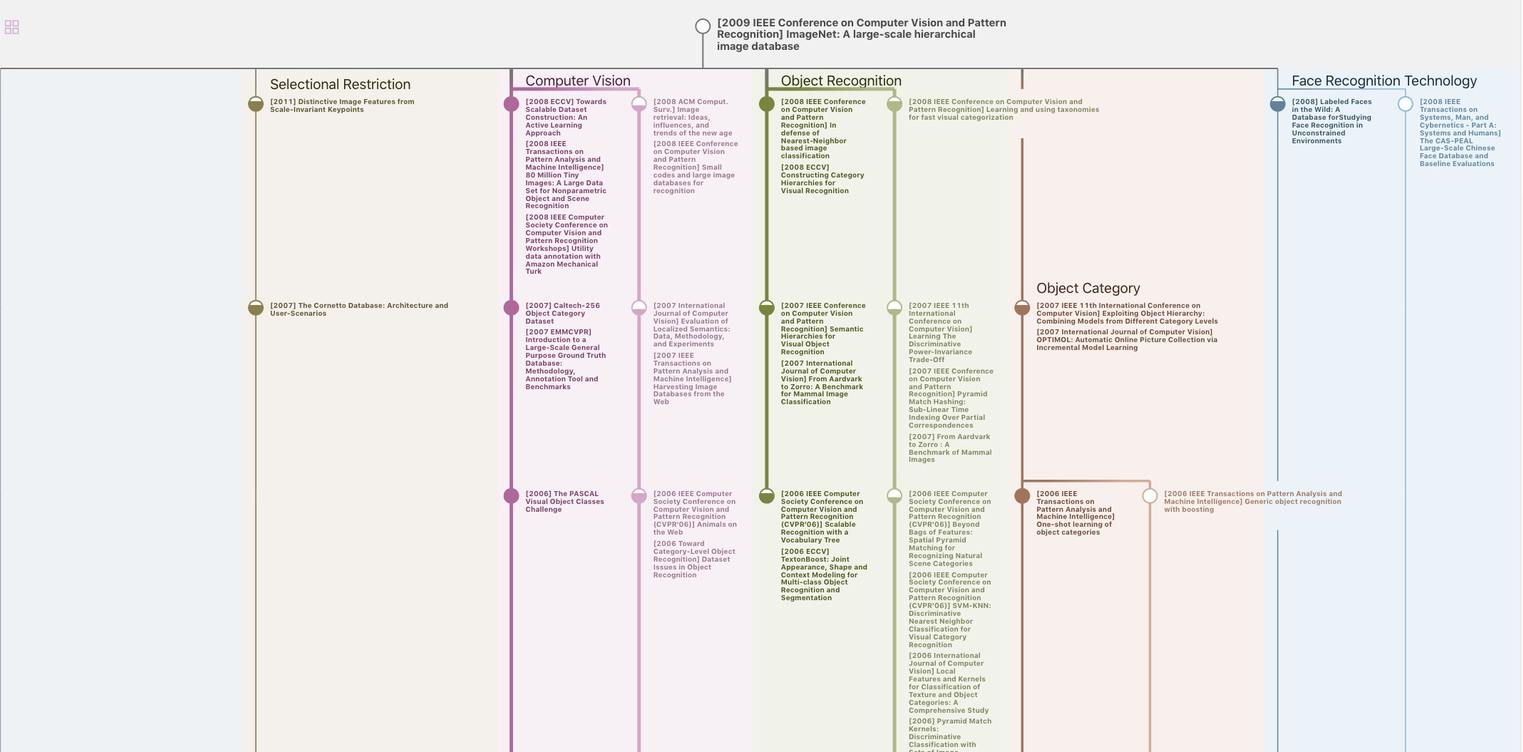
生成溯源树,研究论文发展脉络
Chat Paper
正在生成论文摘要