(Invited) Advanced Modeling of Nanoscale Devices
Meeting abstracts(2023)
摘要
Moore’s scaling law has survived during more than 50 years because the transistor fabrication recipes have been continuously adapted and technology boosters have been gradually introduced, e.g. strain, high- dielectrics, or 3-D FinFETs. The driving force behind these innovations has always been the intuition of clever researchers who benefited from classical technology computer aided design (TCAD) tools. The latter have been used in the semiconductor industry since the end of the 1970’s, when the first 2-D simulations of CMOS devices became feasible on a supercomputer [1]. Over the last 40 years, transistors have undergone tremendous evolutions, their dimensions being reduced by several orders of magnitude, while the physical models at the core of commercially available device simulators have remained the same: electron transport is still described by classical drift-diffusion (DD) equations, which have been modified to capture parts of the quantum mechanical effects affecting nano-transistors, e.g. geometrical confinement tunnelling leakages, or energy quantization [2]. A new generation of advanced TCAD tools that go beyond the DD equations and rely on atomistic quantum mechanical concepts is needed to reproduce the characteristics of today’s nanostructures and to predict the performance of not-yet-fabricated components. Such tools should combine different modelling approaches to be able to treat various device types, from state-of-the-art nano-transistors to photo-detectors based on two-dimensional materials or resistive switching random access memories. For example, to shed light on the behaviour of valence change memory (VCM) cells, which consist of metal-insulator-metal stacks, molecular dynamics (MD), kinetic Monte Carlo (KMC), density functional theory (DFT), and quantum transport (QT) should be allied, as illustrated in the accompanying figure. By doing so, the growth of nano-filaments through realistic oxides embedded between two metallic electrodes can be accurately simulated and the electrical current flowing through computed with, e.g., the Non-equilibrium Green’s Function (NEGF) formalism [3]. In this presentation the multi-method simulation environment shown the accompanying Figure will be briefly reviewed. The main focus will be set on the discussion of few applications, among them transistors and memory cells. References: [1] S. Selberherr, W. Fichtner, and H.W. Potzl, “Minimos - A program package to facilitate MOS device design and analysis”, Proceedings of NASECODE I, 275 (1979). [2] A. Wettstein, A. Schenk, and W. Fichtner, “Quantum device-simulation with the density-gradient model on unstructured grids”, IEEE Trans. On Elec. Dev. 48, 279 (2001). [3] M. Kaniselvan, M. Luisier, and M. Mladenovic, “An Atomistic Modelling Framework for Valence Change Memory Cells”, Solid-State Electronics 199, 108506 (2023). Figure Caption: Multi-method simulation framework dedicated to the investigation of resistive switching devices, here valence change memory (VCM) cells. First, oxide samples with a low defect concentration are constructed withclassical molecular dynamics (MD) using a melt-and-quench procedure. They are thentransferredto akinetic Monte Carlo (KMC) solver that determine s the distribution of oxygen vacancies (V O ,green spheres) within the oxide layer. All input parameters to KMC (di ff usioncoe ffi cient s and generation/recombination rates) are computed with density functional theory(DFT) , which is also used to calculate the Hamiltonian (H) and Overlap (S) matrices of the created VCM structure. Finally, these quantities are passed to a quantum transport (QT) tool to perform ab initiodevice simulations . Figure 1
更多查看译文
关键词
nanoscale devices,advanced modeling
AI 理解论文
溯源树
样例
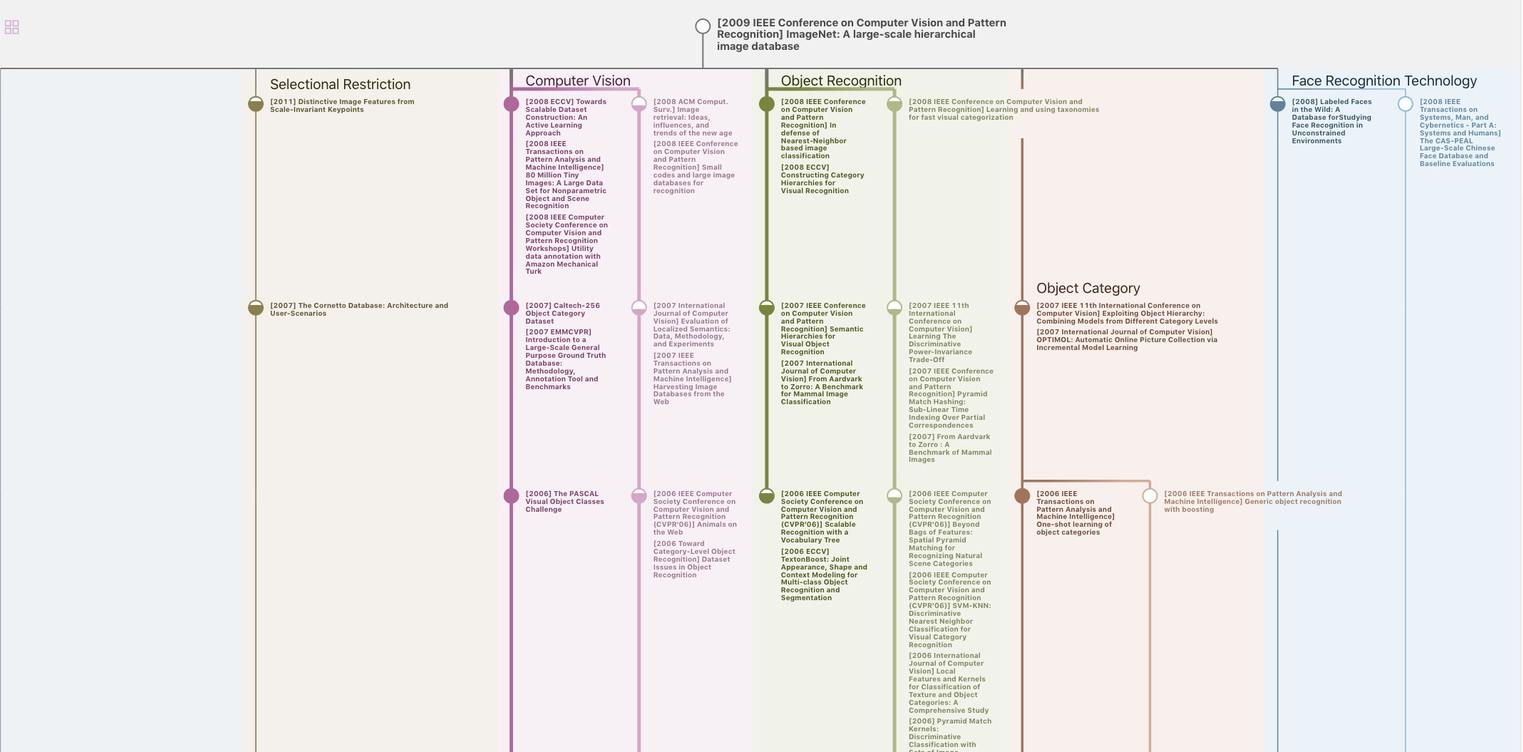
生成溯源树,研究论文发展脉络
Chat Paper
正在生成论文摘要