(Invited) Tuning Photocatalytic Activity with Energetic and Kinetic Asymmetry at Coating-Stabilized Particulate Semiconductors
Meeting abstracts(2023)
摘要
Semiconductor photocatalysts coevolve reductive and oxidative reactions at nanoscale proximity. The local charge separation and local coevolution have unique advantages: i) photocatalysts can operate in neutral pH water or water vapor instead of strong acid or base; ii) most semiconductors form a self-passivating layer at corroded surfaces to prevent corrosion propagation; and iii) nanoparticulate photoabsorbers or nanocrystalline films have achieved near-unity quantum efficiency, promising scale-up solar-to-chemical conversion. Despite the near-unity quantum efficiency achieved for SrTiO 3 particles, the Edisonian approach is applied to the vast majority of photocatalysts, thus limiting progress. Technologically important semiconductors of 1.1 – 2.3 eV bandgaps and properly adjusted band edges, such as GaP and Si, can be ball milled into powder, followed by chemical passivation and protective coating processes. Thus, we introduce the Hu-lab-invented charge-transport coatings to protect semiconductors, such as silicon and gallium indium phosphide. We tune their photocatalytic activity by varying and measuring the energetics and kinetics at local reactive sites, in the following case studies. First, we combine photoelectrochemical cells with local potential-sensing probe microscopy to elucidate photocatalytic processes. In particular, we focus on local energetics and kinetics of multiple concurrent redox processes, i.e., two-redox chemistry. Figure 1A illustrates such a three-terminal photo-electro-catalytic cell setup, where the photoelectrode materials are photocatalysts by themselves. We showcase the efficient charge-separation design by fabricating photocatalysts with particulate semiconductors (e.g., commercial CdS powders, GaInP 2 epitaxial layers, and ball-milled GaP nanoparticles), TiO 2 coatings, and Rh cocatalysts for the concurring reduction of water and oxidation of reversible redox mediators or sacrificial reagents. We discovered that nanoscale site energetics are mutually dependent on local charge-transfer kinetics. [1] Besides, all known visible-light active photocatalysts of 1.1 – 2.3 eV bandgap photo-oxidize or corrode in water. [2] We discovered multi-functional protective coatings that i) allow for both electrons and holes to transport at tunable energy levels; [3] ii) shift band edges favorably, [4] ; iii) reduce charge trapping and recombination, [5] and iv) tune water-oxidation selectivity between O 2 and hydrogen peroxide production. [6] We show that in the presence of quinone redox couples in a bicarbonate solution, CO is produced with a 1-atm CO 2 -free Ar-purged headspace where the only source of CO 2 is the (bi)carbonate anions, whilst the quinone redox couples can systematically vary the band edge positions of GaInP photoabsorbers. As shown in Figure 1B , CO evolution rate for both Fe(CN) 6 3-/4- and H 2 BQ/BQ are higher at pH 7 than pH 8.5. The local CO 2 concentration at pH 8.5 limited CO production rates. This observation corroborates our hypothesis that photocatalytic redox reactions locally drive pH swing to release CO 2 at the oxidative sites, whereas the nearby reductive sites reduce in-situ generated CO 2 into CO2R products. We envision direct solar fuel production from natural resources such as sunlight, bicarbonates from the ocean, or moisture in the air in a durable particle reactor. [7] We also processed wood and leaves into pulps and performed photocatalytic H 2 production from the CdS/TiO 2 /Rh/CoO x panel ( Figure 1C ) using bottom LED illumination, as the glucose solution became colored. In summary, we show the rational design of photocatalysts. These coating-stabilized semiconductor particles present a scalable pathway for making H 2 while effectively activating water and biomass as the two most abundant oxidants. We address the timescale mismatch between light absorption and catalysis by effectively accumulating electrons and holes at the respective reductive and oxidative sites. [8] The study paves the way for utilizing biomass to obtain bioenergy with carbon capture and storage. References: [1] Z. Pan, R. Yanagi, S. Hu, et al. Energy & Environmental Science 2020 . [2] S. Hu, in Handbook on Inorganic Photochemistry , Springer, 2022 . [3] T. Zhao, R. Yanagi, S. Hu, Proceedings of the National Academy of Sciences 2021 , 118 . [4] X. Shen, T. Zhao, S. Hu, et al. Advanced Energy Materials 2022 , 12 , 2201314. [5] X. Chen, X. Shen, S. Shen, M. O. Reese, S. Hu, ACS Energy Letters 2020 , 5 , 1865-1871. [6] J. Li, D. Solanki, Q. Zhu, X. Shen, G. Callander, J. Kim, Y. Li, H. Wang, S. Hu, Journal of Materials Chemistry A 2021 , 9 , 18498-18505. [7] X. Shen, R. Yanagi, D. Solanki, H. Su, Z. Li, C-X Xiang, S. Hu., Frontiers in Energy Research 2021 , 9:7997762021. [8] R. Yanagi, T. Zhao, D. Solanki, Z. Pan, S. Hu, ACS Energy Letters 2022 , 7 , 432-452. Figure 1
更多查看译文
关键词
photocatalytic activity,semiconductors,coating-stabilized
AI 理解论文
溯源树
样例
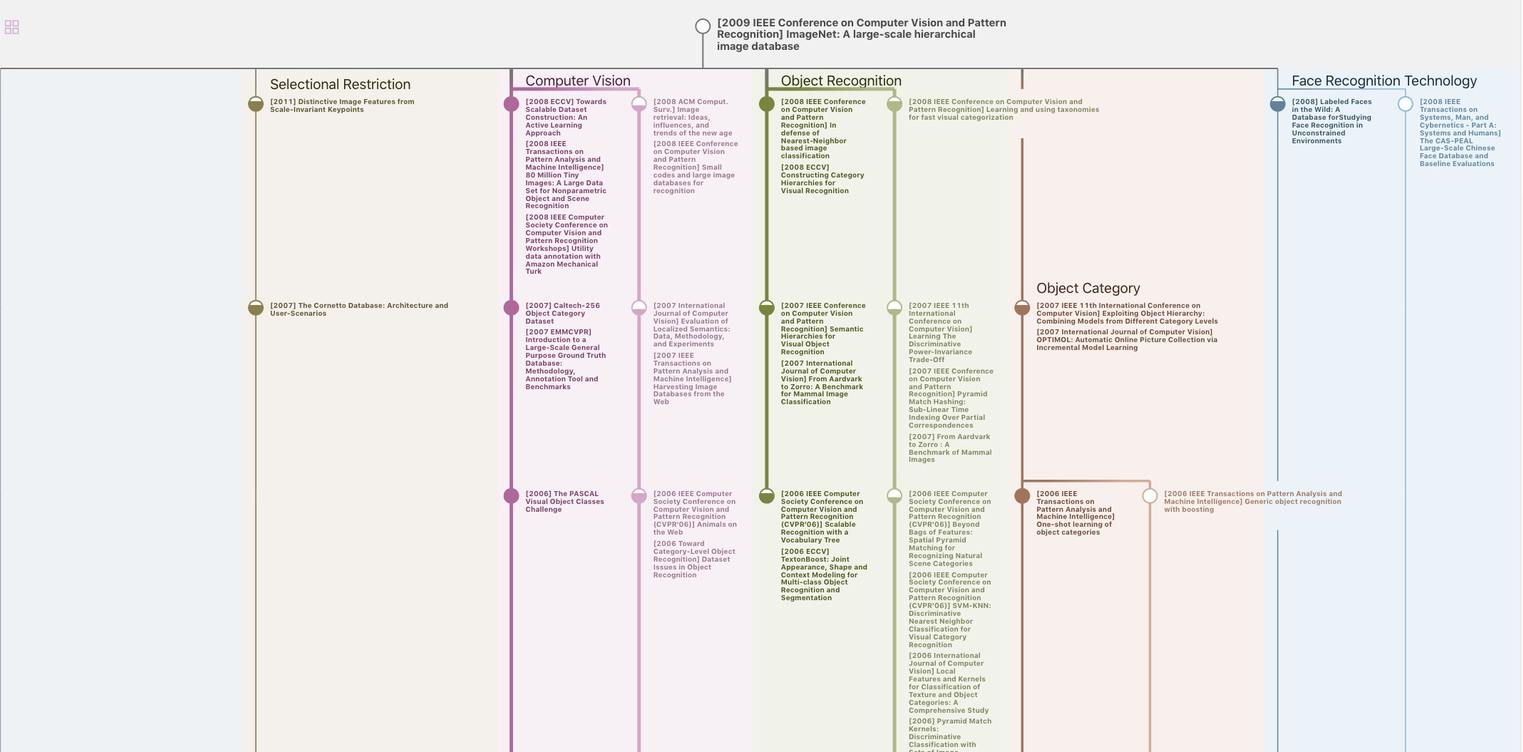
生成溯源树,研究论文发展脉络
Chat Paper
正在生成论文摘要