Down-regulation of xylan biosynthetic GhGT47Bs in cotton impedes fibre elongation and secondary wall thickening during fibre transition
PLANT BIOTECHNOLOGY JOURNAL(2024)
摘要
Cotton provides abundant natural fibres for the textile industry. Cotton fibre development can be divided into five stages: fibre initiation, elongation, transition, secondary cell wall (SCW) thickening and maturation. Fibre initiation and elongation have been extensively studied, but the transition stage remains less investigated. Although cellulose accounts for >90% of mature fibres, non-cellulosic polysaccharides also contribute to fibre development. Little is known about the roles of these carbohydrates during this process. The hemicellulosic polysaccharide xylan peaked in 17 days postanthesis (dpa) fibres in four cotton species with contrasting fibre characteristics, indicating that xylan may function in the transition stage. GhFSN1 and GhMYB46_D13 positively regulate SCW synthesis in cotton fibres (Huang et al., 2021; Zhang et al., 2018), and overexpression of GhFSN1 or GhMYB46_D13 up-regulated the expression of a set of cell wall-related genes. Two GhGT47B genes (Gh_A13G2031 and Gh_D13G2434, designated as GhGT47B_A13 and GhGT47B_D13, respectively) caught our attention as they are annotated as homologues of AtFRA8, which is involved in xylan biosynthesis in Arabidopsis (Chen et al., 2020; Zhong et al., 2005). A yeast one-hybrid analysis coupled with transactivation assay confirmed that both GhGT47B genes can be activated by GhFSN1 and GhMYB46_D13 (Figure S1a–d). RT-qPCR analysis showed that GhGT47B expression peaked in 15, 18 and 20 dpa cotton fibres, and they represent the most abundantly expressed GhGT47B subclade during fibre transition (Figures S2a and S3). Subcellular localization assays indicated that both GhGT47B proteins were located in the Golgi, where xylan is synthesized (Figure S2b). Next, we silenced both GhGT47B genes in cotton. Fibre phenotype analysis in the T1 and T2 generations revealed that mature fibres of RNAi lines were significantly shorter than those of the wild type (Figure S4). We confirmed these results in two independent RNAi lines of the T3 generation (Figure 1a–c). In addition, the cell wall thickness of mature cotton fibres of RNAi lines was significantly thinner than that of wild type (Figure 1d,e). Fibre quality parameters analyses showed that the length, breaking strength, elongation and micronaire value of fibres in RNAi lines significantly decreased (Table S1). Moreover, the degree of twisting of most cotton fibres of GhGT47B RNAi lines was largely reduced (Figure 1f,g), suggesting that the breaking strength in RNAi fibres declined. We next examined xylan abundance in the RNAi lines by immunolabelling. We found weaker fluorescence intensity of 15 dpa and 21 dpa cotton fibre cell walls of RNAi compared with wild type (Figure 1h). Monosaccharide composition analysis validated that the xylose content significantly decreased in RNAi fibres (Figure 1k). Further NMR analysis showed that the xylan structure in wild-type cotton fibre and Arabidopsis stem appeared largely similar. Interestingly, there is a new peak in the spectrum of cotton (highlighted in the blue rectangular box), which is absent in the Arabidopsis xylan (Figure 1i). Nevertheless, the structure of xylan reducing end sequence (RES) in cotton fibre and Arabidopsis stem is different. G1, R1 and X1 are peaks represented by different monosaccharides constituting the RES. R1 and X1 of cotton fibre can hardly be detected, but the G1 peak is more pronounced, while there is little difference in the height of G1, R1 and X1 peaks in Arabidopsis stem (Figure 1i). Through calculation, the relative abundance of RES in the GhGT47B RNAi lines was 21% lower than that of wild type, implicating GhGT47B activity in the synthesis of xylan RES in cotton fibre. To explore whether the decrease of xylan content impacts cellulose synthesis, we used cellulose-specific fluorescent dye pontamine fast scarlet 4B to stain the fibre resin slices. The fluorescence of 15 dpa, 21 dpa and mature cotton fibres of RNAi transgenic lines was obviously fainter than those of wild type (Figure 1j). These results were corroborated by crystalline cellulose measurements (Figure 1l) and indicate that cotton fibres produce less cellulose when GhGT47B is down-regulated. To further investigate genes and pathways affected by GhGT47B, we sequenced the transcriptome of 18 dpa fibre of GhGT47B RNAi cotton and the wild type. Gene Ontology (GO) enrichment analysis showed that several terms involved in cell wall synthesis and modification were enriched, confirming that GhGT47B is associated with cell wall biogenesis and/or organization (Figure S5a–d). Further analysis showed that genes related to synthesis of xylan backbone, side chain modification and genes involved in cellulose synthesis are among the differentially expressed genes (Figure S5e,f). It is plausible cell wall change is sensed by a monitoring pathway that then regulates growth to ensure sufficient strength during fibre development. Based on the transcriptome analysis, we summarized the mode in which down-regulation of GhGT47B might affect cell wall components (Figure S6). Fibre development requires many cell wall-related genes. Exploring these genes will provide insights into cell wall modifications, with the aim to ultimately make unique cotton fibres to better suit our needs. Our findings support the involvement of xylan in fibre development and might work as a template to manipulate xylan synthesis to fine-tune cell wall composition for fibre improvement. WX and WZ designed the research. YJG, YNG, FC, JL, MQ and ML performed the experiments. WX, WZ, YJG and SP analysed the data. YJG and WX wrote the manuscript. This work was supported by the National Natural Science Foundation of China (grant numbers: 31671735 and 31970516) and the Foundation of Hubei Hongshan Laboratory (grant number: 2021hszd014). SP acknowledges the financial aid of a Villum Foundation (25915). The authors declare no conflict of interest. Figure S1 GhGT47B promoters can be activated by GhFSN1 and GhMYB46_D13. Figure S2 Expression pattern and subcellular localization analysis of GhGT47B. Figure S3 Expression analysis of GhGT47B_A13 and GhGT47B_D13 during fibre transition. Figure S4 Phenotypic analysis of first generation (T1) and T2 GhGT47B RNAi cotton. Figure S5 RNA-Seq analysis of 18 dpa fibres of GhGT47B RNAi cotton. Figure S6 Mode in which down-regulation of GhGT47B affects cell wall components. Table S1 Comparison of fibre quality parameters. Please note: The publisher is not responsible for the content or functionality of any supporting information supplied by the authors. Any queries (other than missing content) should be directed to the corresponding author for the article.
更多查看译文
关键词
cotton fiber,fiber transition stage,xylan biosynthesis,GhGT47B,cellulose,cell wall integrity
AI 理解论文
溯源树
样例
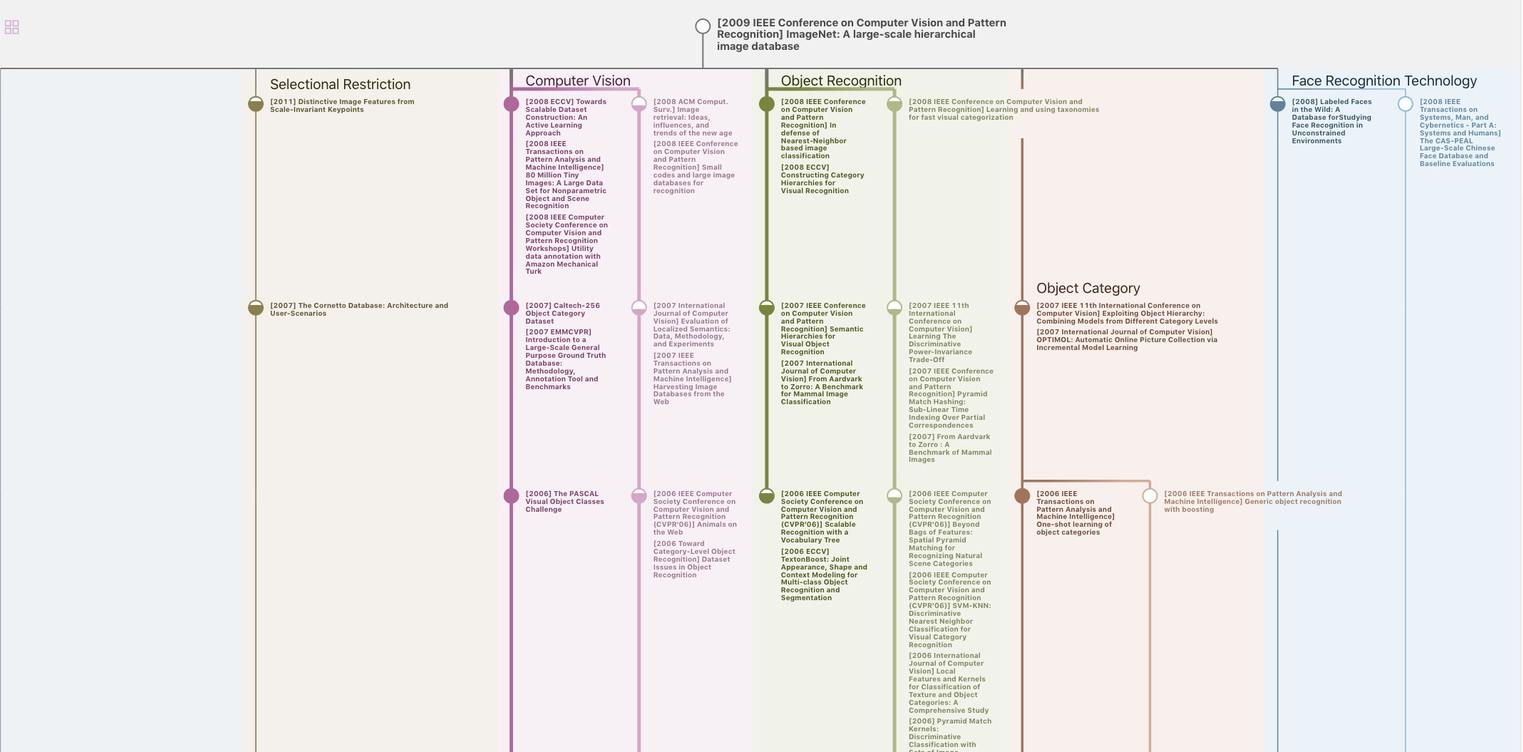
生成溯源树,研究论文发展脉络
Chat Paper
正在生成论文摘要