Novel Therapeutic Modalities: The Future is Now.
Clinical pharmacology and therapeutics(2023)
摘要
In the past 20 years, we have seen a wave of innovative technology and novel drug modalities that may overcome limitations of classical therapeutic approaches, such as small molecules. In 2021 alone, ~ 100,000 journal articles were published on these new therapeutic approaches.1 However, only 10% of recent original research articles (ORAs) published in Clinical Pharmacology & Therapeutics (CPT) focus on novel modalities, which may at least in part be a reflection of the fact that many emerging approaches are still in early stages of discovery and development and that their clinical benefits remain to be established.2 However, the landscape has been changing rapidly and several “new” modalities have been advancing through development and registration in recent years. For example, cell therapy has emerged as a novel cancer treatment approach, with 12 approvals of chimeric antigen receptor (CAR)-T cell therapies since 2017, including 4 in 2022.3 Likewise, the number of new antibody-drug conjugates reaching the clinical trial stage increased by nearly 5-fold in the last 5 years, with 21 currently in late-stage development.4 Whereas there were only a handful of new bispecific antibody (bsAb) clinical candidates per year in the first decade of this century, recent years have seen a remarkable growth for this modality class, with more than 50 bsAbs reaching clinical trials in 2021.5 One example is rozibafusp alfa, a first-in-class bispecific IgG2-peptide fusion designed to inhibit inducible T-cell costimulator ligand and B-cell activating factor, for which the first clinical trial results in patients with rheumatoid arthritis are reported in this issue.6 Additionally, in 2022, the US Food and Drug Administration (FDA) approved 5 new therapeutics that modify gene expression or function (2 gene therapies, 2 gene-modified cell therapies, and 1 small interfering RNA) and a first microbiota therapy for treatment of Clostridium difficile infection.7 In the European Union and United Kingdom, 12 “advanced therapy medicinal products” were marketed as of March 2022 with another 482 in clinical development.8 This revolution in novel modalities requires clinical pharmacology scientists, educators, and practitioners to quickly adapt and develop new skill sets and expertise.9-11 If the modest contribution to date of ORAs focusing on novel modalities to the overall content of CPT2 is also a reflection of a perhaps somewhat limited role of our discipline in the development of nontraditional therapeutics,12 then now is the time to (re)position ourselves more clearly. In this light, the current CPT themed issue (Figure 1) can be seen as a call to action. We invited key opinion leaders to review the current knowledge and experience of the development of novel modalities (including cell and gene therapies for cancers and rare disease, vaccines, targeted protein degraders, oncolytic viruses, and digital therapeutics) and the role of clinical pharmacology throughout the research and development process. The coronavirus disease 2019 (COVID-19) pandemic dramatically affected people's lives and countries' health systems, but it was also an emergency-driven opportunity to develop advanced therapeutic technology from bench to bedside at an unprecedented speed.10, 13 Heaton et al.14 highlight key lessons from the COVID-19 pandemic on the importance of trust, collaboration, and transparency in health care and vaccine production. They stress the importance of innovations in system capacities and advances in technologies, identify 3 key factors that facilitated timely COVID-19 vaccine development, and suggest 3 other areas of opportunity to improve communication and to build trust. Cell and gene therapies (CGTs), such as viral vector-based gene therapies and CAR-T cell therapies, have the potential for transformational efficacy in treating diseases with high unmet needs. However, these novel modalities also tend to have a higher rate of disruption after the conclusion of the pivotal trial and before regulatory submission, and a higher rate of disruptions during regulatory review than conventional therapeutics, such as monoclonal antibodies.15 Therefore, it is crucial to use careful, yet innovative, strategies for the development of CGTs. In a perspective, Jodele and co-workers report that the dose optimization strategy for eculizumab could be beneficial in adeno-associated virus (AAV) gene therapy in thrombotic microangiopathy, suggesting a potential opportunity to apply contemporary knowledge to precision medical care.16 In parallel, in their White Paper, Mitra et al.17 present a comprehensive overview of applications of model-informed drug development approaches in dose selection and innovative trial design strategies for the development of AAV-based gene therapy products. They suggest several novel approaches to address the challenges in gene therapy clinical trials, such as small patient sample size or equivocal natural history of the disease, and present various tools to support future gene therapy development.17 In another White Paper, Mody and collaborators from the International Consortium for Innovation and Quality in Pharmaceutical Development present an industry perspective on best clinical pharmacology practices for the development of CAR-T and T cell receptor T cell therapies, including clinical study design and modeling and simulation strategies, underscoring the unique and critical role of clinical pharmacology.18 This is further exemplified in the study by Masilamani et al.,19 who compare bioanalytical methods for characterization of CAR-T cellular kinetics. Unlike proteins or antibodies, CAR-T are “living cell” therapies whose pharmacokinetics (PKs) are characterized by expansion, distribution, contraction, and persistence. Therefore, this unique modality requires a different approach for quantitation compared with conventional ligand binding assays implemented for most biologics. With regard to the role of health authorities, regulatory agencies encourage early interaction with sponsors to align on key elements of CGT development programs, because each therapy will have a specific risk–benefit profile depending upon its platform and mode of delivery, therapeutic indication, and demography of study participants. The comprehensive review by Walford and coworkers of current FDA and European Medicine Agency (EMA) guidelines relating to CGT entering into the clinic should therefore be a timely resource.20 Targeted protein degradation (TPD) is an emerging therapeutic approach that potentially modulates proteins that are “undruggable” with conventional small molecules.21 Since the first TPD molecule that harnesses the ubiquitin–proteasome system to degrade a target protein was reported, several biotech and pharmaceutical companies entered the field and disclosed programs in preclinical and early clinical development. However, to date, limited information and guidance has been made available with regard to the clinical pharmacology aspects of TPDs. In their State-of-the-Art contribution entitled “Clinical Translation of Targeted Protein Degraders,”22 Kong and Jones from the Dana-Farber Cancer Institute provide an overview of TPDs in clinical trials and a perspective on the lessons learned from their development and emerging human data. This may provide a useful source for clinical pharmacologists working in this rapidly growing field, specifically with regard to PK-pharmacodynamic (PK-PD) profiling, the use of biomarkers, safety, and the emergence of the new class of E3 ligases to overcome the reliance on hijacked E3 ligase that may cause drug resistance. Clustered regularly interspaced short palindromic repeats (CRISPR)/CRISPR-associated protein systems have caused a transformational breakthrough for genomic editing in the clinic. The unique mechanism of action of CRISPR therapy requires new approaches for clinical translation and dose selection. Abdelhady and colleagues review the current status of clinical pharmacology and translation in the CRISPR field, with an emphasis on a mechanistic, quantitative approach to address the uncertainties from other empirical approaches in clinical development and study design.23 Physiologically-based PK (PBPK) and quantitative systems pharmacology (QSP) modeling approaches are also evolving to support development of novel modalities. Parra-Guillen et al.24 describe the development and application of a mechanistic PBPK model linked to tumor response to assess clinical response to an oncolytic virus. Renardy et al.25 demonstrate the potential value of mechanistic models for an entirely different class of novel modalities: microbiome therapies. Describing the kinetics and behavior of live biotherapeutic products (LBPs) poses a unique challenge for quantitative clinical pharmacologists, because they can expand, contract, and colonize the host digestive tract. A cellular kinetic-PD QSP model of an LBP was developed which describes bacterial growth and competition, vancomycin effects, binding and unbinding to the epithelial surface, and production and clearance of butyrate as a therapeutic metabolite. The model provides the basis for model-informed LBP development and can be used to inform decision making around antibiotic pretreatment and dose regimens. Ribba et al.26 show how in silico approaches themselves have become novel modalities in their own right. In their review, they make the case that fundamental clinical pharmacology principles can benefit the development of digital therapeutics (generally known as software applications embedded within accessible technologies–such as smartphones–to treat, manage, or prevent a pathological condition) in three areas: characterization of the mechanism of action, optimization of the intervention, and dosing. We propose that these conclusions can be applied to the development of any novel therapeutic modality and the editorial leadership of CPT expects to publish many more examples of this in the coming years. No funding was received for this work. The author declared no competing interests for this work.
更多查看译文
AI 理解论文
溯源树
样例
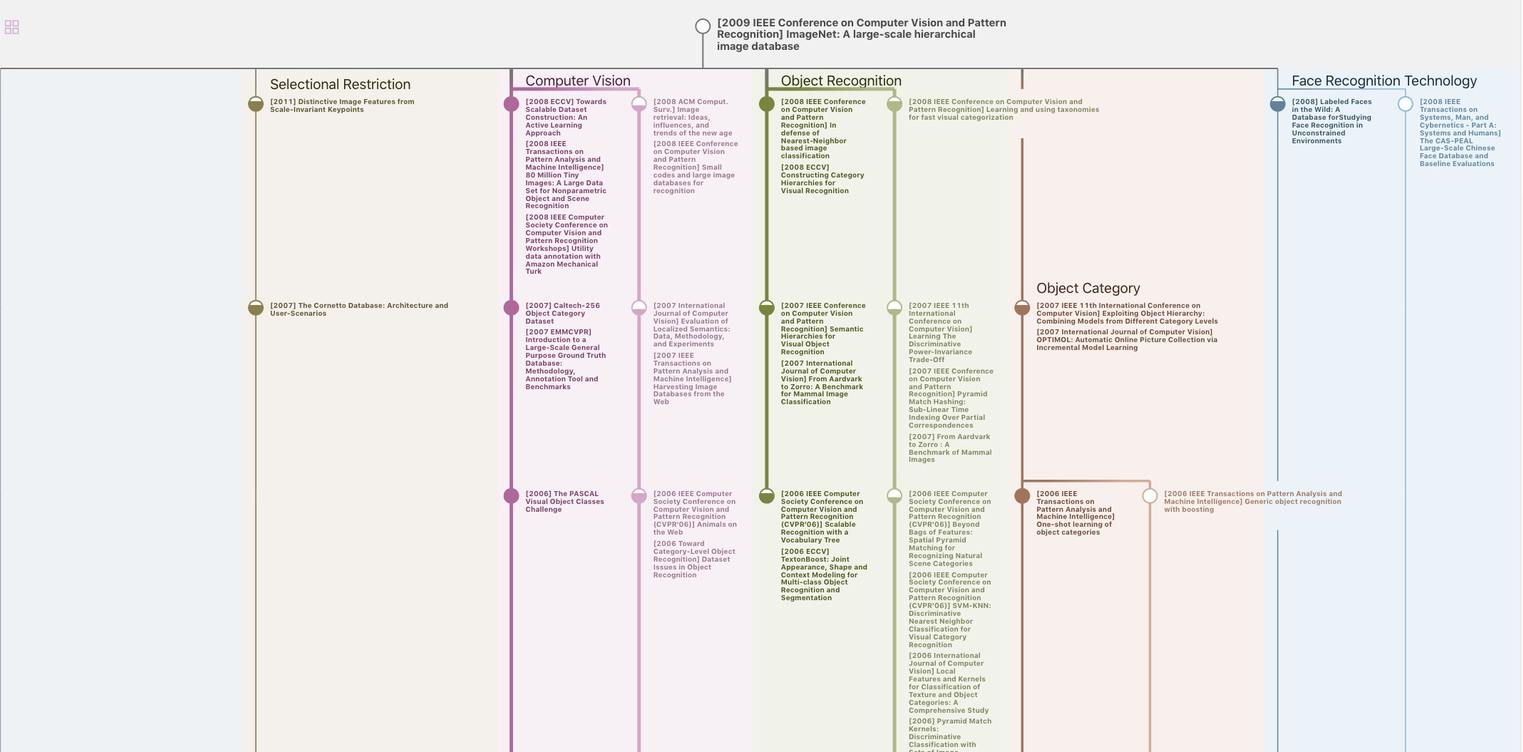
生成溯源树,研究论文发展脉络
Chat Paper
正在生成论文摘要