XIth Cajal Conference: New frontiers in neuron-glial plasticity in health and disease
EUROPEAN JOURNAL OF NEUROSCIENCE(2023)
摘要
The brain is a complex assembly of interconnected neurons where synapses enable the transmission of information. Synapses are progressively established in the brain via mechanisms of neuronal competition, where the neurons that fire together, wire together (Hebb, 1949). In other words, the most efficient synapses are strengthened and maintained throughout life, enabling the brain to have a plastic response to the ever-changing environment. This process, globally known as synaptic plasticity, was thought to involve exclusively pre-synaptic and post-synaptic neurons, through the fine regulation of calcium channels and neurotransmitter receptors, among others. Indeed, the classical view of the synaptic connection is an electrical signal leading to the release of a neurotransmitter from the pre-synaptic neuron, which then binds on receptors in the post-synaptic neuron, ultimately inducing a behavioural response. Obviously, complex learning and memory processes in the mammalian brain are more elaborate than this simplistic view and are rooted in a precise regulation of molecular and cellular players. In the last couple of decades, the concept of plasticity has been expanded, as new evidence has uncovered that, besides neurons, glial cells also take a fundamental role in synapse development, homeostasis and plasticity throughout life. Today, astrocytes, microglia and oligodendrocytes and their precursors are considered essential elements for the regulation of synaptic functions and their malleability. In the XIXth century, the Spanish neuroanatomist Santiago Ramón y Cajal already suggested that learning may be mediated by strengthening synapses to improve the efficiency of communication. The classical perception of modulating brain connectivity is long-term potentiation (LTP), a lasting increase in synaptic strength following high-frequency stimulation of a synapse (Lømo, 2003); and its counterpart long-term depression (LTD). Our increasing understanding of plasticity mechanisms has shed light on crucial activity-dependent synaptic regulation and the active role of glial cells, providing a more holistic conception of how the brain functions. The miscellaneous plasticity mechanisms of the brain are in fact guided by the almost infinite possibilities to fine-tune the efficacy of information transmission. Spatial and temporal readjustments include mechanisms affecting the different cell types present, stabilization or destabilization processes, release and receptors binding of neurotransmitters and modulation of the electrical signal, among others. Impairments in the formation, remodelling, strengthening and pruning of synapses underlie most neurodevelopmental and neurological disorders. A better comprehension of these mechanisms would be a stepping-stone to develop pharmacological treatments for pathologies that are fatal nowadays, including Alzheimer's disease. The XIth Cajal Conference ‘New Frontiers in Neuron-Glial Plasticity in Health and Disease’, organized by the Spanish Society of Neuroscience (SENC), which took place in Sant Feliu de Guíxols, Spain (18–20 May 2022) (Figure 1), was devoted to discussing this concept of neuronal-glial plasticity, both in physiological and pathological conditions. The conference occurred in an open and informal atmosphere, enabling lively and fruitful discussions between participants, both plenary speakers, and PhD students and early career postdoctoral researchers. Students recorded entertaining video abstracts to present the latest publications of the plenary speakers and the whole attendance enjoyed cultural and recreational activities, such as a guided visit to the local History Museum and kayaking on the Mediterranean coast. This editorial summarizes the main findings discussed at the conference. These included new insights into pre-synaptic plasticity mechanisms, with a focus on the involvement of protein synthesis in long-term potentiation, as well as post-synaptic ones, highlighting the relevance of regulating the membrane fusion of synaptic receptors. The astrocytic control of synaptic transmission and its function in plasticity was also a matter of debate, as well as the role of microglia in sculpting and remodelling synapses. Finally, the impairment of these plasticity mechanisms in neurodegenerative diseases was addressed and the relevant roles of proteases, such as presenilin and a disintegrin and metalloproteinase 10, were discussed. Understanding how neuronal circuits are stabilized throughout time, making some of them more efficient whereas others are weakened, is a major task in neuroscience. This complex process refers to activity-dependent modifications of the strength in synaptic transmission and is regulated by the release of several neurotransmitters from the pre-synaptic to the post-synaptic neuron. Synaptic plasticity enables mammals to adapt to lifetime experiences and this modification of synaptic strength in neurons occurs in two different ways: by modifying the pre-synaptic elements (e.g., by increasing the opening of calcium channels; Scarnati et al., 2020) or by modulating the expression of post-synaptic receptors (Woo et al., 2020; Wu et al., 2020). The relative importance of pre-synaptic and post-synaptic plasticity mechanisms has been a subject of debate throughout the years (Padamsey & Emptage, 2013) and was also the source of thrilling discussions at the Cajal Conference. Pablo E. Castillo (Albert Einstein College of Medicine, USA) gave new insights into how pre-synaptic protein synthesis is involved in LTP. During pre-synaptic LTP, the cyclicAMP/Protein Kinase A signalling cascade is activated (Castillo, 2012) (Figure 2), whereas at the post-synaptic membrane, neurotransmitters bind different post-synaptic receptors, enabling synaptic transmission. The elements taking part in the post-synaptic plasticity are well-defined, whereas the pre-synaptic ones remain unclear (Monday & Castillo, 2017), but both of them contribute in different ways to learning processes (Mizusaki et al., 2022). One form of pre-synaptic plasticity in the mature brain is mediated by retrograde signalling of the endocannabinoid (eCB) system (Castillo et al., 2012). Castillo provided evidence demonstrating the implication of cannabinoid-receptor 1 (CB1) in mediating LTD of inhibitory transmission (Monday et al., 2020). This form of pre-synaptic plasticity involves a protein-synthesis-dependent long-lasting reduction in γ-aminobutyric acid (GABA) release (Younts et al., 2016). The relevance of pre-synaptic protein synthesis was also discussed by Arnaldo Parra-Damas from Carlos A. Saura's group (Institut de Neurociències UAB, Spain). He highlighted that neuronal activity triggers CREB-regulated transcription coactivator-1 (CRTC1) activity, inducing transcription in pre-synaptic neurons in the hippocampus (Parra-Damas et al., 2014; Parra-Damas et al., 2017). Castillo also raised new considerations about the recurrent circuit between hilar mossy cells (MC), the first synapses in which pre-synaptic long-term plasticity was reported (Kobayashi et al., 1996; Zalutsky & Nicoll, 1990), and granule cells (GC). This synaptic connection takes place in the dentate gyrus of the hippocampus, one of the main brain regions responsible for learning and memory processes. LTP at this synapse enhances dentate gyrus output by increasing excitation/inhibition balance (Hashimotodani et al., 2017), and in some diseases such as epilepsy, this balance is altered. Thus, this hippocampal brain circuit might contribute to early stages of epileptogenesis by contributing to seizure generation and propagation (Nasrallah et al., 2022). Castillo also exposed that the synapses between these two excitatory cell types undergo a novel pre-synaptic, N-methyl-d-aspartate receptor (NMDAR)-independent, form of LTP that requires post-synaptic brain-derived neurotrophic factor (BDNF)/TrkB signalling and pre-synaptic cyclic AMP (cAMP)/PKA (Hashimotodani et al., 2017) (Figure 2). This pre-synaptic cAMP is also needed in other processes such as pre-synaptic axonal arbor co-stabilization, as described by Alice Louail from Amanda Sierra's laboratory (Achucarro Basque Center for Neuroscience, Spain). She explained how the reduction of cAMP signalling in a subset of neurons facilitates the elimination of axonal projections from neighbouring neurons (Louail et al., 2020). In the debate between pre- and post-synaptic plasticity mentioned earlier, the role of the post-synaptic neuron was advocated by Sandra Jurado (Instituto de Neurociencias de Alicante, Spain), who discussed the relevance of mechanisms of dendritic exocytosis underlying post-synaptic plasticity. Membrane fusion mechanisms, which require the participation of soluble N-ethylmaleimide sensitive factor attachment protein receptor (SNARE) and Sec1/Munc18 (SM)-like proteins, are involved in vesicle exocytosis from pre-synaptic terminals (Südhof & Rizo, 2011). However, little is known about the mechanisms mediating membrane fusion of synaptic receptors at the post-synaptic level. A classical measure of post-synaptic changes in synaptic strength is the ratio between two glutamatergic receptors: α-amino-3-hydroxy-5-methyl-4-isoxazolepropionic acid (AMPA) receptors (AMPARs) and NMDARs. Because these two receptors have different kinetics of activation and NMDAR enables Ca2+-dependent plasticity (Malenka & Nicoll, 1999), this AMPA/NMDA ratio, which increases in LTP and decreases in LTD, is indicative of what occurs in post-synaptic glutamatergic synapses (Counotte et al., 2014). Jurado's presentation was focused on the machinery that mediates the activity-dependent AMPARs exocytosis during LTP. She reported that the SNARE protein synaptobrevin 2/vesicle-associated membrane protein 2 (VAMP2) is present in both the regulated AMPAR exocytosis during LTP and in constitutive basal AMPAR exocytosis. However, two other SNARE proteins, syntaxin-3 and SNAP-47, are only required for the regulation of the process during LTP (Arendt et al., 2015; Jurado et al., 2013; Madrigal et al., 2019) (Figure 2). This supports the idea of a unique SNARE-mediated fusion machinery needed by the post-synaptic exocytosis of AMPARs during LTP (Jurado, 2014). Thus, specific SNARE complexes regulate different forms of plasticity. In this context, Rocío Rojas, from Núria Casals' laboratory (International University of Catalonia, Spain), suggested that the trafficking of AMPARs to the plasma membrane can be regulated by different nutrients such as saturated and unsaturated fatty acids. In addition to the post-synaptic exocytosis of AMPARs, there are additional exocytic events at dendrites, such as the release of endogenous neuropeptides, that play an important role in modulating synaptic transmission and plasticity. Jurado emphasized the importance of the hypothalamic neuropeptides oxytocin and vasopressin, two neuromodulators whose balance determines an appropriate social behaviour (Rigney et al., 2022; Torres et al., 2018). In order to measure the developmental dynamics of endogenous neuropeptides, her group used the immunolabeling-enabled three-dimensional imaging of solvent-cleared organs (iDISCO+) clearing technique (Renier et al., 2016) together with light-sheet ultraresolution imaging to obtain 3D reconstructions of the entire oxytocinergic and vasopressinergic systems in the central nervous system (CNS). She reported how this multidisciplinary approach enabled advances in the understanding of these neuromodulatory circuits. Strikingly, she described that distinct hypothalamic nuclei show strong differences between the expression of oxytocin and vasopressin depending on the developmental stage (Madrigal & Jurado, 2021). These adaptations can contribute to defining different neuronal circuits affecting functional properties of plasticity and the modulation of social behaviours. Although the first day of the Cajal Conference was focused on the pre- and post-synaptic regulation of plasticity between neurons, these are not the only cells implicated in synapses. Some decades ago, it was described that glial cells also participate in this process. Glial cells (astrocytes, microglia, oligodendrocytes and others) represent a significant population of brain cells and account for at least half of total brain volume (Azevedo et al., 2009). The second day of the Cajal Conference was devoted to the role of glial cells in regulating synapses and brain plasticity. Besides his studies of neurons, Santiago Ramón y Cajal devoted many studies to glial cells (Ramón y Cajal, 1897) and their role in brain function (Navarrete & Araque, 2014). Yet, their role was revealed only a century later. At the Cajal Conference, Alfonso Araque (School of Medicine, University of Minnesota, USA) launched the second day providing a beautiful summary on the astrocytic control of synaptic transmission and plasticity. In 1998, his work ultimately demonstrated the essential role of astrocytes in regulating synaptic transmission and plasticity. It had been known for almost a decade that astrocytes express a large array of neurotransmitter and neuromodulator receptors, such as glutamate (NMDA, AMPA, kainate, mGluR), GABA, acetylcholine (ACh), dopamine (DA). Many of these receptors are coupled to second-messenger signalling that induces the release of Ca2+ from astrocytes (Verkhratsky et al., 1998). Under high-frequency stimulation of glutamtergic axons, glutamate ‘spills over’ from synapses and activates glutamate receptors on astrocytes, causing an elevation of their intracellular Ca2+ levels (Porter & McCarthy, 1996). Astrocytic calcium activity also induces astrocytic release of glutamate in culture and in hippocampal slices (Bezzi et al., 1998; Kimelberg et al., 1990). This astrocytic Ca2+ increase induces neuronal Ca2+ increase in culture (Hassinger et al., 1995; Nedergaard, 1994). All these findings strongly suggest a crucial role for astrocytes in synapses. Araque's work reported that astrocytes are actually able to regulate synaptic activity in opposite manners. On the one hand, they play a role in glutamate-dependent depression, reducing the amplitude of excitatory post-synaptic currents (EPSCs) and inhibitory post-synaptic currents (IPSCs) (Araque, Parpura, et al., 1998). On the other hand, astrocytic NMDAR activation induces intracellular Ca2+ elevation which increases the frequency, but not the amplitude, of EPSC and IPSCs in neighbouring neurons (Araque, Sanzgiri, et al., 1998). In line with previous reports showing that synaptic neurotransmission induces a Ca2+ wave which propagates in adjacent astrocytes in culture (Cornell-Bell et al., 1990), Alfonso Araque demonstrated that this activity-induced Ca2+ wave leads to astrocytic release of glutamate that binds to glutamate receptors in pre-synaptic neurons and inhibits their neurotransmitter release (Araque, Parpura, et al., 1998) (Figure 3). This observation, combined with the observation, using 3D reconstruction, that astrocytic processes envelop the synaptic cleft (Ventura & Harris, 1999), led him to propose a new concept, revolutionizing the perception of synapses: the ‘Tripartite synapse’ (Araque et al., 1999). Since then, the tripartite synapse has been the focus of tremendous work. Astrocytes respond distinctly to synchronous or miniature synaptic events, generating either robust regional Ca2+ elevations, or producing highly confined, fast miniature Ca2+ responses, respectively (Di Castro et al., 2011). Astrocytes can also discriminate between the activity of different synapses belonging to different pathways (Perea & Araque, 2005) or to different patterns and frequency of input activity. Their range of responses varies with distinct amplitude, frequency, kinetics and spatial diffusion (Araque et al., 2014). When post-synaptic activity coincides with astrocytic Ca2+ elevation, astrocytic glutamate release can trigger synaptic potentiation. This transient astrocytic potentiation depends on region-specific activation of pre-synaptic neurotransmitter receptors: metabotropic glutamate receptors (mGluR) in the CA1 region (Navarrete et al., 2012; Navarrete & Araque, 2010; Perea & Araque, 2007) and NMDAR in the dentate gyrus (Jourdain et al., 2007). The coincidence of post-synaptic activity and Ca2+-mediated glutamate release from astrocytes triggers the long-term potentiation (LTP) of synaptic transmission (Perea & Araque, 2007). In contrast, in the cortex, glutamate released from stimulated astrocytes activates pre-synaptic NMDAR and mediates LTD of excitatory transmission by activating pre-synaptic NMDAR (Min & Nevian, 2012). Astrocytes can also potentiate inhibitory transmission by acting on pre-synaptic kainate receptors in the hippocampal CA region (Araque et al., 2014). Other neurotransmitters than glutamate have been shown to induce astrocytic Ca2+ response. DA seems to be able to modulate Ca2+ concentration in opposite directions. In the hippocampus, DA signalling on astrocytic D1 and D2 receptors mobilizes Ca2+ from intracellular stores and leads to a strong Ca2+ increase, whereas DA signalling only on D2 receptors induces Ca2+ decrease (Jennings et al., 2017) (see Figure 3). At the Cajal Conference, Araque presented the latest research of his laboratory. Although most of the research performed on the tripartite synapse has been done in the hippocampus, they focused on another brain region, the nucleus accumbens. They showed that optogenetic stimulation of DA axons activates astrocytic D1 receptors and induces astrocytic Ca2+ elevations. These calcium elevations provoke the release of ATP/adenosine that mediates LTD at the synapse (Corkrum et al., 2020), suggesting they participate in the synaptic regulation of the brain reward system. Endocannabinoids released by hippocampal neurons bind to astrocytic CB1 receptors and induce Ca2+ elevations (Navarrete & Araque, 2010) (see Figure 3). Astrocytic response to chronic exposure to tetrahydrocannabinol (THC) was the subject of Cristina Martín-Monteagudo's presentation from Marta Navarrete's laboratory (Instituto Cajal, CSIC, Spain). Neuronal cholinergic activity on astrocytic muscarinic and mGluR receptors induces astrocytic Ca2+ elevations leading to LTP in hippocampal synapses (Araque et al., 2002; Navarrete et al., 2012). Araque's group is also investigating global network cortical activity. During sensory stimulation, cortical astrocytes increase their Ca2+ activity and interact with neurons to modulate their response. These cortical astrocytes are able to negatively regulate low-frequency (0–4 Hz) delta activity and positively regulate sensory-evoked high-frequency (30–50 Hz) gamma activity (Lines et al., 2020). The role of astrocytes in regulating neuronal network synchronization was also the subject of Claudia Miguel-Quesada's presentation from the laboratory of Juliana M. Rosa and Juan Aguilar (Hospital Nacional de Parapléjicos, Spain). Network desynchronization and synaptic dysfunction are a common feature for a large number of brain pathologies that often involve both neurons and glia. Álvaro Fernández-Blanco from Mara Dierssen's laboratory (Center for Genomic Regulation, Spain) urged for a better understanding on the role of astrocytes dysfunction and impaired astrocyte to neuron communication in memory impairments (Fernández-Blanco & Dierssen, 2020). How astrocytes are able to finely regulate synaptic transmission with such a wide range of responses remains a subject of debate. Another type of glial cells is microglia, first mentioned by Pío del Río Hortega in 1919 (Rio-Hortega, 1919). Traditionally linked to inflammatory responses, it took over 50 years to uncover their many important roles in physiology (Sierra et al., 2019). Microglia are the brain ‘constant gardeners’, continuously scanning the brain parenchyma with their highly dynamic processes (Davalos et al., 2005; Nimmerjahn et al., 2005). Once their physiological function in the brain parenchyma was established, it only took a few years for the role of microglia in synaptic plasticity to be revealed. In the following years, it was demonstrated that microglia express a wide variety of ion channels, connexins, cytokines and chemokine receptors, and a wide range of neurotransmitters, neurohormone and neuromodulator receptors (Kettenmann et al., 2011). Similar to astrocytic receptors, most of the neurotransmitter receptors expressed by microglia trigger intracellular Ca2+ transients, suggesting they are involved in neuronal connectivity. At the Cajal Conference, Rosa Paolicelli (University of Lausanne, Switzerland) gave a comprehensive talk providing an overview of the main roles of microglia in synapse monitoring and remodelling. In both the developing and mature nervous system, microglia transiently contact synaptic elements with their dynamic processes and present phagocytic structures (Ji et al., 2013; Tremblay et al., 2010; Wake et al., 2013; Wu et al., 2015), pointing to a role for microglia in regulating synapse formation and stabilization. Paolicelli's work was pioneer in demonstrating that microglia engulf synaptic material and that this process is necessary for physiological brain and synapse development (Paolicelli et al., 2011). Because surveying microglia are responsible for the phagocytosis of apoptotic neurons (Sierra et al., 2010), it was suggested that microglia engulfment of synapses is mediated by phagocytosis, or, more recently, by ‘trogocytosis’ (Weinhard et al., 2018). The laboratory of Beth Stevens provided the beautiful demonstration that microglia prune synaptic elements in an activity-dependent manner. Synaptic material is engulfed by microglia and colocalizes with lysosomal markers (Schafer et al., 2012). As a consequence, the concept of ‘four-way’ or ‘Quad-partite’ synapse emerged (Paolicelli & Gross, 2011; Schafer et al., 2013). The major tasks known today for microglia at synapses involves structural and functional remodelling, particularly at early developmental stages, in an activity-dependent fashion. Several molecular pathways, including fractalkine signalling, DAP12/triggering receptor expressed on myeloid cells 2 (TREM2) signalling, phosphatidylserine and the complement pathway, have been implicated in the process of synapse removal by microglia (Filipello et al., 2018; Paolicelli et al., 2011; Schafer et al., 2012; Scott-Hewitt et al., 2020). Microglia-mediated remodelling of synapses is an age-dependent and activity-dependent process. In the developing visual system, the peak of microglial phagocytosis coincides with preferential microglia-mediated pruning of connectivity and synapses coming from the ‘weaker’ eye (Schafer et al., 2012). Microglial contacts with synapses and phagocytic structures present in microglial cells are reduced when neuronal activity is altered, suggesting that microglia respond to neuronal activity (Tremblay et al., 2010; Wake et al., 2013). Indeed, microglia can respond to excitatory and inhibitory neurotransmission. Active glutamatergic neurons release ATP which binds to the purinergic receptor P2Y12 and mediates microglial process extension towards the synapse (Eyo et al., 2014; Fontainhas et al., 2011) (see Figure 3). GABA signalling displays opposite effects on microglia and decreases the motility of their processes (Fontainhas et al., 2011). Fractalkine signalling is required for microglia to sense ATP and participate in synaptic plasticity (see Figure 3). Fractalkine-deficient models have been reported to present defective microglial processes migration, impaired LTP induction, reduced ratio of spontaneous/miniature EPSC, decreased learning and memory performances, social and stereotyped behavioural defects (Pagani et al., 2015; Paolicelli et al., 2011; Rogers et al., 2011; Zhan et al., 2014). Most of the research on microglial synaptic pruning has focused on excitatory synapses, and little is known about their role in inhibitory circuits. Microglia are involved in the positioning and connectivity of cortical parvalbumin interneurons (Thion et al., 2019), suggesting they also have a role in inhibitory synapse development. Very recently, it has been shown that specialized microglia, expressing the GABAB1 receptor, prune inhibitory synapses via a complement-dependent mechanism (Favuzzi et al., 2021). This suggests that microglia participate in the development and maintenance of the excitation/inhibition balance in the brain. It is clear today that brain and synapse development need more than only one or two cell types; and plasticity mechanisms involving more than two cell types have already been reported. Microglial stimulation of astrocytes via purinergic signalling leads to astrocytic glutamate release that modulates the spontaneous activity of neighbouring neurons (Pascual et al., 2012). Hippocampal astrocytes can release lactate, which participates in the microglia-dependent induction of synaptic LTD (Zhang, Malik, et al., 2014) (Figure 3). Interestingly, astrocytic lactate release is also required for LTP and memory formation in the hippocampus (Newman et al., 2011; Suzuki et al., 2011). At the Cajal Conference, Paolicelli presented one of her laboratory's latest research projects concerning the role of lactate in microglial metabolism, such as intracellular signalling or lysosomal acidification (Monsorno et al., 2022). Lactate transporters are expressed by many brain cells, including neurons and microglia. Lactate dehydrogenase B, which preferably converts lactate into pyruvate, is one of the most abundant genes expressed by microglia (Pinto et al., 2012). New molecular pathways involved in brain connectivity are regularly identified, and the role of the different cell types remains to be fully understood. The bidirectional communication between neurons and microglia was a topic of compelling discussions between several participants of the Cajal Conference. Several speakers discussed the region-specific aspects of microglial maturation, as it has previously been described in cerebellar microglia (Ayata et al., 2018; Stowell et al., 2018), which could play a part in the region-specific patterns of development in the brain. Another brain cell population, expressing the neural/glial antigen 2 (NG2) proteoglycan, was identified at the end of the XXth century (Stallcup & Beasley, 1987). They were first described as the main source of oligodendrocytes in the mature brain and referred to as oligodendrocytes precursor cells (OPCs) (Dawson et al., 2000). Their morphology resembles the one of mature astrocytes, with highly motile processes like microglia, but they have a distinct gene signature (Haberlandt et al., 2011). NG2 cells express a large array of ion channels, neurotransmitter and neuromodulator receptors (Larson et al., 2016), and they are the only glial cells that receive synapses, both excitatory and inhibitory (see Figure 3). Similar to astrocytes and microglia, activation of neurotransmitter receptors induces Ca2+ elevations in NG2 cells, mainly localized in their processes (Haberlandt et al., 2011). They respond to excitatory synapses with EPSC and modify the LTP of neighbouring neurons (Ge et al., 2006), although they cannot generate action potentials (De Biase et al., 2010). Synapses on the surface of NG2 cells appear as soon as the cells are generated and are transferred to daughter cells when they divide, but they disappear when NG2 cells differentiate to oligodendrocytes, along with a drastic change in their transcriptomic signature (Kukley et al., 2008; Ge et al., 2009; De Biase et al., 2010; Kukley et al., 2010; Zhang, Chen, et al., 2014). The amplitude of synaptic currents in NG2 cells increases during the peak period of myelination of many brain areas (Mangin et al., 2008; Vélez-Fort et al., 2010), suggesting a role for synaptic signalling in the development and differentiation of NG2 cells, therefore playing a role in myelination. Indeed, oligodendrocyte differentiation can be regulated by glutamatergic (Cavaliere et al., 2013; Chen et al., 2018), GABAergic (Serrano-Regal et al., 2020) or cannabinoid signalling (Sánchez-de la Torre et al., 2022). At the conference, Alice Staffa from Isabel Perez-Otaño's laboratory (Neuroscience Institute of Alicante, Spain) presented her work concerning the role of the glutamatergic receptors on the differentiation of NG2 cells into oligodendrocytes. The role of this panel of receptors on these cells remains to be determined. Recently, a new role for NG2 cells in neural circuits development has been uncovered in the visual cortex. Young NG2 cells are able to engulf and prune pre-synaptic terminals in an activity-dependent manner (Auguste et al., 2022), similar to microglia. Finally, in addition to glial cells, the concept of synapse has been further expanded to include the extracellular matrix (ECM) as an essential part of it (Dityatev & Rusakov, 2011; Dityatev & Schachner, 2003; Dityatev & Schachner, 2006). All these elements (pre- and post-synaptic neurons, glial cells and ECM molecules) are important for the maintenance of functional synapses and have a key role in the regulation of synaptic plasticity, thus affecting learning and memory processes (Pelucchi et al., 2022). The third day of the Cajal Conference was focused on the pathological consequences of the impairment of synaptic plasticity. In that regard, the participants tackled this important issue from different angles, including a pre- and post-synaptic perspective, as well as the involvement of specific neurotransmitter circuits and glial cells in neurodegenerative processes. Most of the time was devoted to Alzheimer's disease (AD), the most common neurodegenerative disease worldwide. AD, which affects an estimated 50 million people worldwide, is a fatal disease with no effective treatment that gradually impairs learning and memory. The main histopathological hallmark of AD is th
更多查看译文
关键词
multipartite synapse, neurodegenerative diseases, synaptic plasticity
AI 理解论文
溯源树
样例
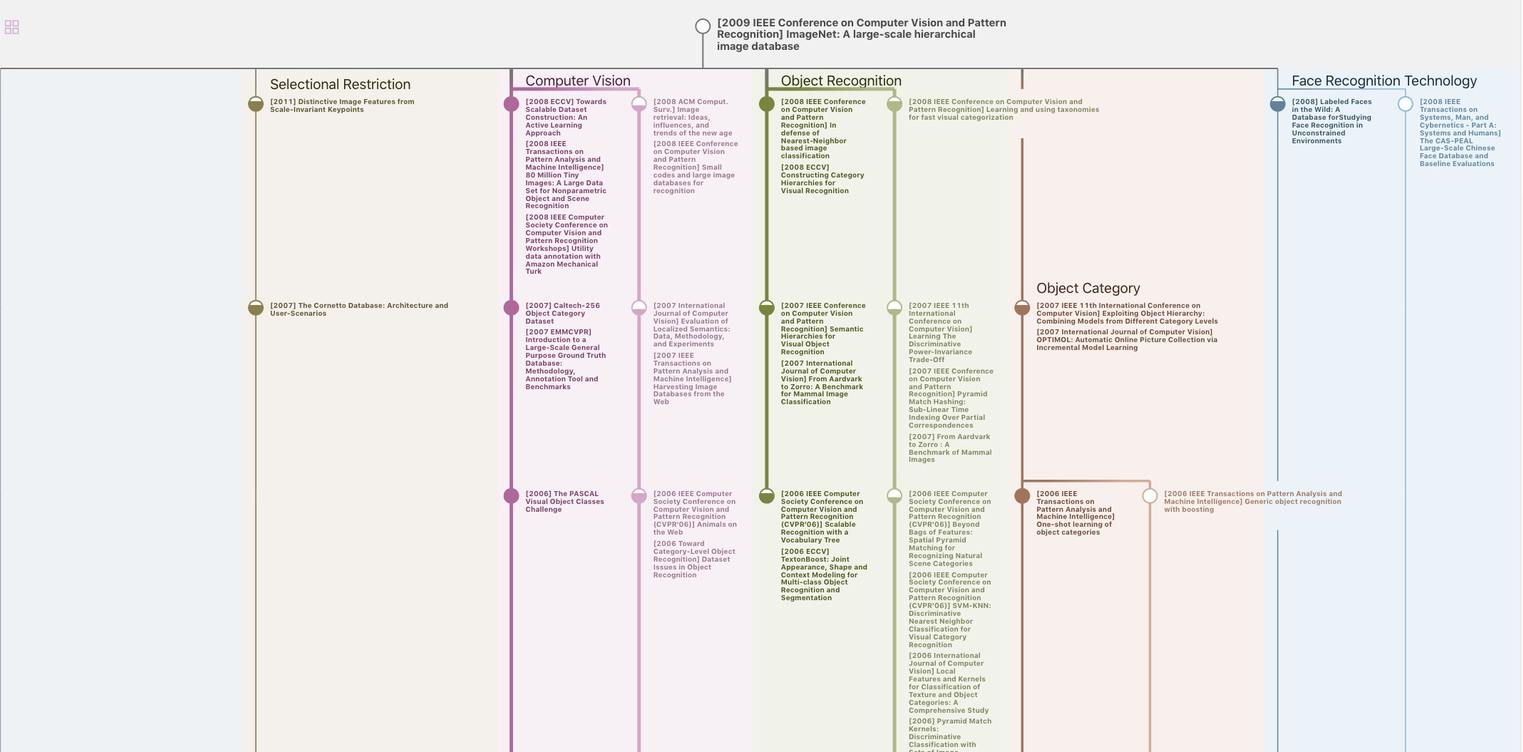
生成溯源树,研究论文发展脉络
Chat Paper
正在生成论文摘要