Detection of viable SARS-CoV-2 in retrospective analysis of aerosol samples collected from hospital rooms of patients with COVID-19
Clinical Microbiology and Infection(2023)
摘要
Managing the COVID-19 pandemic has required the implementation of public health mitigation measures to limit the transmission of SARS-CoV-2. Airborne transmission via particles of different sizes, generally called droplets and aerosols, was recognized very late by public health organizations in part due to limited direct evidence of infectious virus in air samples. SARS-CoV-2 RNA has been detected in indoor air samples in various settings [[1]Borges J.T. Nakada L.Y.K. Maniero M.G. Guimaraes J.R. SARS-CoV-2: a systematic review of indoor air sampling for virus detection.Environ Sci Pollut Res Int. 2021; 28: 40460-40473https://doi.org/10.1007/s11356-021-13001-wCrossref PubMed Scopus (31) Google Scholar], but to this day only a few studies reported infectious virus particles in bioaerosols [2Vass W.B. Lednicky J.A. Shankar S.N. Fan Z.H. Eiguren-Fernandez A. Wu C.Y. Viable SARS-CoV-2 Delta variant detected in aerosols in a residential setting with a self-isolating college student with COVID-19.J Aerosol Sci. 2022; 165106038https://doi.org/10.1016/j.jaerosci.2022.106038Crossref PubMed Scopus (7) Google Scholar, 3Lednicky J.A. Lauzard M. Fan Z.H. Jutla A. Tilly T.B. Gangwar M. et al.Viable SARS-CoV-2 in the air of a hospital room with COVID-19 patients.Int J Infect Dis. 2020; 100: 476-482https://doi.org/10.1016/j.ijid.2020.09.025Abstract Full Text Full Text PDF PubMed Scopus (358) Google Scholar, 4Santarpia J.L. Herrera V.L. Rivera D.N. Ratnesar-Shumate S. Reid S.P. Ackerman D.N. et al.The size and culturability of patient-generated SARS-CoV-2 aerosol.J Expo Sci Environ Epidemiol. 2022; 32: 706-711https://doi.org/10.1038/s41370-021-00376-8Crossref PubMed Scopus (45) Google Scholar, 5Kitagawa H. Nomura T. Kaiki Y. Kakimoto M. Nazmul T. Omori K. et al.Viable SARS-CoV-2 detected in the air of hospital rooms of patients with COVID-19 with an early infection.Int J Infect Dis. 2023; 126: 73-78https://doi.org/10.1016/j.ijid.2022.11.003Abstract Full Text Full Text PDF PubMed Scopus (3) Google Scholar]. Well-defined methods to monitor indoor air remain essential to inform on the risks of acquisition in the community and occupational environments and to evaluate mitigation methods [[1]Borges J.T. Nakada L.Y.K. Maniero M.G. Guimaraes J.R. SARS-CoV-2: a systematic review of indoor air sampling for virus detection.Environ Sci Pollut Res Int. 2021; 28: 40460-40473https://doi.org/10.1007/s11356-021-13001-wCrossref PubMed Scopus (31) Google Scholar,[6]Silva P.G. Branco P. Soares R.R.G. Mesquita J.R. Sousa S.I.V. SARS-CoV-2 air sampling: a systematic review on the methodologies for detection and infectivity.Indoor Air. 2022; 32e13083https://doi.org/10.1111/ina.13083Crossref PubMed Scopus (1) Google Scholar]. The quantity and infectivity of viral particles collected from air is strongly influenced by the samplers, the environmental context, the time of sampling and sample storage before cell culture requiring level 3 containment laboratory. In this study, we sought to assess the possibility of isolating infectious SARS-CoV-2 virus particles in a retrospective analysis of aerosol samples. We collected air samples in individual airborne isolation hospital rooms with negative pressure and >12 air changes/hour occupied by patients with acute COVID-19 in fall 2020 in Quebec, Canada when the Alpha variant was circulating but not yet detected in Quebec and before vaccines were available. Thirty samples were collected in 10 different rooms using two types of samplers selected based on previous reports, namely 37mm closed-face cassettes with 0.8μm polycarbonate filters (SKC, Eighty Four) with a flow rate of 10L air/min or a condensation growth tube (CGT) air sampler (Series 110A Liquid Spot Sampler, Aerosol Devices) with a nominal flow rate of 1.5L air/min, located at 2-3m from the patient's bed with sampling duration of 4.75-20h to cover sporadic events generating viral aerosols (Fig. 1A and B). Samples eluted in Viral Transport Media (VTM) were stored at -80°C. SARS-CoV-2 RNA (ORF1b) was detected by quantitative RT-PCR (RT-qPCR) in 9/22 (40.9%) cassettes and 2/8 (25%) Spot Sampler samples with concentration ranging from 129 to 2056 genomes equivalent/m3 air (Fig. 1B). We interrogated for the presence of replicating virus in four samples (two Spot Sampler and two cassettes) collected from the same patient room among the highest RNA concentrations detected (Fig. 1B). The samples had been stored 14 months before carrying out the cell culture experiments. We first confirmed that our cell culture design clearly differentiated between replicating and non-replicating virus. VERO E6 cells were inoculated with 150 pfu (chosen based on the mean copy number of the air samples analyzed) of replicating or β-propiolactone (BPL)-inactivated SARS-CoV-2/SB2 isolate (obtained from Dr. Samira Mubareka, Sunnybrook Research Institute, Toronto, Canada). Signs of cytopathic effects (CPE) attributable to SARS-CoV-2 replication (Fig. 1C), cellular expression of spike (S) and nucleocapsid (N) proteins (Fig. 1D), and production of de novo virions quantified via the median tissue culture infectious dose (TCID50) were observed at 3 days of infection with the replicating virus (Fig. 1E), while none of these parameters turned positive 2 hours after inoculation, or when using BPL-inactivated SARS-CoV-2. Having confirmed that only actively replicating virus led to positive read-outs, we proceeded with the analysis of air samples. As air samples contained low SARS-CoV-2 RNA levels (Fig. 1B), two successive cycles of infection of VERO E6 cells were carried out to maximize the amplification of the virus and therefore the detection of viable particles (Fig. 1F). One sample (#23), collected using the Spot Sampler, induced detectable CPE, with destruction of the monolayer inferior to that observed after infection with 150 pfu of SARS-CoV-2/SB2 isolate (Fig. 1G), cellular S and N expression (Fig. 1H) and de novo production of infectious virions (Fig. 1I). The titer of virions after 2 cycles of infection was of 6.32 × 107 TCID50/mL, which is 5.67 times lower than the titer observed after infection with 150 pfu of SARS-CoV-2/SB2 isolate (Fig. 1I). TCID50 analysis also allowed the detection of virions (3.57 × 102 TCID50/mL) in the supernatant of cells infected with sample #22 after 2 rounds of infection, while neither CPE nor S and N expression were observed (Fig. 1G–I). Neither of the two samples collected with the cassette produced detectable CPE, viral proteins expression or de novo virions (Fig. 1G–I). We combined measurement of virus-induced CPE, immunoblotting of viral proteins and titration of infectious virions as read-outs to provide clear demonstration of the infectivity of the virus. Immunoblotting against viral proteins confirmed the identity of SARS-CoV-2 in sample #23. RT-qPCR of N performed on the supernatant at day 3 of the second inoculation (not shown), further confirmed the presence of SARS-CoV-2. No SARS-CoV-2 N RNA was detected in sample #22 making it impossible to infer on the presence of SARS-CoV-2 in this sample. The only sample from which we successfully retrieved virus capable of replicating in cell culture was collected using the Spot Sampler. This is consistent with previous reports suggesting that CGT samplers allow collection of airborne SARS-CoV-2 and at least partially preserve virion infectivity [[2]Vass W.B. Lednicky J.A. Shankar S.N. Fan Z.H. Eiguren-Fernandez A. Wu C.Y. Viable SARS-CoV-2 Delta variant detected in aerosols in a residential setting with a self-isolating college student with COVID-19.J Aerosol Sci. 2022; 165106038https://doi.org/10.1016/j.jaerosci.2022.106038Crossref PubMed Scopus (7) Google Scholar,[3]Lednicky J.A. Lauzard M. Fan Z.H. Jutla A. Tilly T.B. Gangwar M. et al.Viable SARS-CoV-2 in the air of a hospital room with COVID-19 patients.Int J Infect Dis. 2020; 100: 476-482https://doi.org/10.1016/j.ijid.2020.09.025Abstract Full Text Full Text PDF PubMed Scopus (358) Google Scholar]. Patient's (45-year-old female known only for diabetes) characteristics that may have influenced aerosolization of SARS-CoV-2 were no immunization against COVID-19, symptomatic for 7 days including severe cough, and requirement of oxygen administration by nasal canula (2L/min) on the day of air sampling. No aerosol generating procedure occurred during air sampling. A nasopharyngeal swab collected 48h prior to the air sampling was positive for SARS-CoV-2 (Fig. 1B). In conclusion, we provide much needed additional evidence for the presence of replicating SARS-CoV-2 virions in bioaerosols [[3]Lednicky J.A. Lauzard M. Fan Z.H. Jutla A. Tilly T.B. Gangwar M. et al.Viable SARS-CoV-2 in the air of a hospital room with COVID-19 patients.Int J Infect Dis. 2020; 100: 476-482https://doi.org/10.1016/j.ijid.2020.09.025Abstract Full Text Full Text PDF PubMed Scopus (358) Google Scholar,[4]Santarpia J.L. Herrera V.L. Rivera D.N. Ratnesar-Shumate S. Reid S.P. Ackerman D.N. et al.The size and culturability of patient-generated SARS-CoV-2 aerosol.J Expo Sci Environ Epidemiol. 2022; 32: 706-711https://doi.org/10.1038/s41370-021-00376-8Crossref PubMed Scopus (45) Google Scholar]. Our results highlight the possibility to recover replicative virus particles in air samples after freezing at -80°C in VTM and storage for several months. Our results are important as they provide rationale for retrospective evaluation of the presence of infectious SARS-CoV-2 in samples collected during the different waves since 2020. All experiments related to SARS-CoV-2 virus culture were conducted in a certified containment level-3 (CL3) facility, using standard operating procedures approved by the Biosafety committee at Centre de Recherche du Centre Hospitalier de l'Université de Montréal, Montreal, Canada. The characteristics of patients occupying the rooms with detectable airborne infectious virus were extracted by reviewing their medical chart. Institutional Review Board of the Institut Universitaire de Cardiologie et de Pneumologie de Québec gave ethical approval for this work (MEO-21-2021-3475). A waiver of individual written informed consent was approved for the air samplings and the retrospective extraction of patient information from medical records. YL declares receiving a research grant from Syneos Health unrelated to the current study. The other authors declare that they have no conflicts of interest. This work was supported by the Fonds de recherche du Québec-Santé COVID initiative (295848 to CD) and the Fondation du Centre Hospitalier de l’Université de Montréal (to NG). The authors thank Dr. Samira Mubareka (Sunnybrook Research Institute, Toronto, Canada) for the SARS-CoV-2/SB2 isolate used as a control in this study and Dr. Arinjay Banerjee (Vaccine and Infectious Disease Organization, University of Saskatchewan, Saskatoon, Canada) for advice for SARS-CoV-2 culture and Magali-Wen St-Germain for Fig. 1A. Fig. 1F has been created with BioRender.com. AF, MV, CD and NG are members of the Réseau de recherche en Santé du Québec (RSR-Q). CD and NG are members of the Réseau Québécois COVID – Pandémie (RQCP). NG is a member of the Coronavirus Variants Rapid Response Network (CoVaRR-Net). CD holds a Tier-1 Canada Research Chair on Bioaerosols.
更多查看译文
AI 理解论文
溯源树
样例
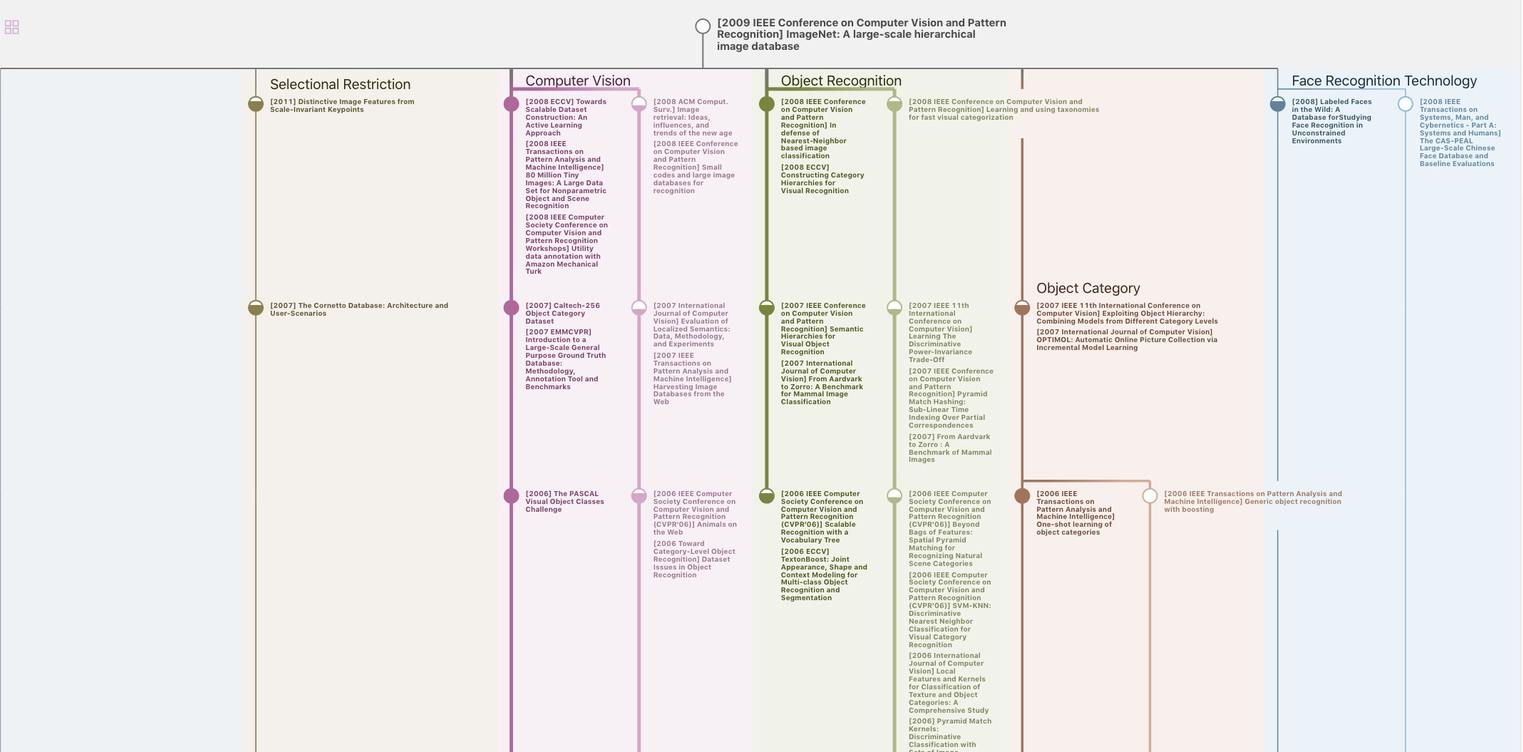
生成溯源树,研究论文发展脉络
Chat Paper
正在生成论文摘要