Excessive Erythrocytosis Is Not Associated With Altered Iron Homeostasis in Men From the World's Highest City.
HemaSphere(2023)
摘要
Iron is required for hemoglobin synthesis and red blood cell (RBC) production. When erythropoiesis is stimulated, erythroferrone, a hormone produced by erythroblasts in response to erythropoietin (EPO), downregulates hepcidin transcription in the liver. Lower hepcidin levels facilitate ferroportin-mediated iron absorption in the duodenum and iron export from reticuloendothelial cells that process old/damaged RBCs,1 thereby ensuring iron availability to the erythropoietic compartment. During acute high-altitude hypoxia, increased erythropoiesis results in iron mobilization, leading to decreased iron stores.2,3 By contrast, healthy permanent residents of altitudes of 3700–4380 m, chronically exposed to hypoxia, keep iron stores within the normal range, despite continuously high erythropoietic activity and iron demand.4,5 Iron stores are also preserved in individuals living at 3825–4380 m who are affected by chronic mountain sickness (CMS),6,7 a clinical syndrome characterized by symptomatic excessive erythrocytosis and severe hypoxemia.8 However, the mechanisms enabling iron stores to be maintained in high-altitude residents have not been described. The aim of this study was to explore how iron homeostasis adapts to chronic exposure to hypoxia in polycythemic residents from the world’s highest city, La Rinconada, located at 5100 m,9 both with and without CMS. The study was approved by the Peruvian ethics committee of Universidad Nacional Mayor de San Marcos in Lima (CIEI-2019-002), and written and oral consent was obtained from each individual before participation. One hundred male participants were included in this study presenting original data on iron parameters. Of these, 69 were included in our previous study on total RBC volume (RBCV)9 (Suppl. Figure S1). Of the 100 participants, 57 were permanent residents in La Rinconada (5100 m) and categorized into no CMS, mild CMS or moderate-to-severe CMS; 26 were permanent residents in Puno (3800 m), also categorized into no CMS, mild CMS or moderate-to-severe CMS; and 17 were healthy residents from Lima (sea level). Participants’ ancestry is reported in Suppl. Table S1. Serum samples were used for biochemical determinations. Total hemoglobin mass (Hbmass) and RBCV were assessed by carbon-monoxide rebreathing. Details on participant recruitment, CMS scoring, methods, and statistics are available in the Suppl. Methods. In line with our previous report,9 all hematologic parameters, in particular Hbmass and RBCV, progressively increased with altitude, reaching extremely high values in residents from La Rinconada (Table 1). In those individuals, excessive erythrocytosis was accompanied by increased EPO9 and soluble transferrin receptor (sTfR) levels (Table 1), confirming chronically high erythropoietic activity. However, despite high iron requirement in the bone marrow, healthy residents—particularly those at 5100 m—did not modify iron metabolism, as both erythroferrone and hepcidin concentrations remained similar to those in sea-level residents. Accordingly, healthy high-altitude residents did not develop iron deficiency (indicated by unaltered transferrin saturation and ferritin), although calculated body iron stores tended to decrease at the highest altitude (Figure 1). The absence of iron deficiency at 5100 m was associated with an increase in ceruloplasmin, a ferroxidase that permits ferroportin-to-transferrin iron transfer1 (Table 1). Table 1 - Characteristics, Hematological Profiles, Total Hemoglobin Mass, and Intravascular Volumes in CMS Patients and Non-CMS Residents at 3800 and 5100 m Sea Level (Lima) 3800 m (Puno) 5100 m (La Rinconada) Altitude (No CMS) CMS 3800 m CMS 5100 m No CMS No CMS Mild CMS Mod-Sev CMS No CMS Mild CMS Mod-Sev CMS P Participant characteristics N 17 18 5 3 17 24 16 Age, y 28 (23–35) 31 (23–43) 49 (49–50) 53 (53–54)a 38 (35–47)b 41 (36–48) 45 (43–49) 0.007 0.002 0.261 Body weight, kg 69 (64–75) 67 (63–74) 70 (65–80) 74 (66–82) 70 (65–74) 70 (65–74) 68 (63–76) 0.844 0.751 0.886 Height, m 1.69(1.66–1.71) 1.68(1.63–1.70) 1.65(1.60–1.65) 1.63(1.59–1.65) 1.65(1.62–1.72) 1.66(1.61–1.69) 1.64(1.61–1.66) 0.467 0.095 0.428 BMI, kg.m-2 24.1(23.2–25.0) 25.6(22.3–27.0) 25.7(25.4–29.4) 26.9(25.5–30.4) 25.0(24.0–26.3) 25.1(24.5–26.7) 24.9(23.5–28.1) 0.595 0.425 0.896 Duration of stay, y _ 25 (23–37) 50 (49–51) 52 (51–53) 10 (10–20) 10 (6–15) 15 (12–21) <0.001 0.020 0.159 [Hb], g·dL-1 13.6(13.4–14.4) 18.4(18.0–19.4)b 20.9(18.3–21.7) 24.0(22.4–24.2) 21.6(20.0–23.4)b , c 22.7(22.3–23.8) 23.9(22.8–25.1)a <0.001 0.062 0.010 Hematocrit, % 43.8(43.0–46.3) 55.4(54.6–56.2)b 65.0(60.3–66.0) 69.5(67.3–72.0)a 68.8(61.0–73.0)b , c 71.3(66.5–74.3) 74.9(73.0–78.2)a <0.001 0.025 0.019 SpO2, % 98 (98–98) 94 (92–94)b 87 (83–92) 84 (82–85) 81 (80–85)b , c 81 (78–85) 79 (77–81) <0.001 0.036 0.111 CMS score with [Hb] 0 (0–2) 1 (0–2) 8 (7–8)a 11 (11–13)a 4 (3–5)b , c 8 (6–9)a 12 (11–13)a , d <0.001 <0.001 <0.001 CMS score without [Hb] 0 (0–2) 1 (0–2) 5 (5–7)a 8 (8–10)a 2 (1–2) 5 (3–6)a 9 (8–10)a , d 0.089 <0.001 <0.001 Hematological characteristics EPO, mIU·mL−1 8.41 (6.22–10.46) 13.63 (8.03–17.47) 13.93 (10.74–17.22) (n = 4) 15.75 (12.32–23.24) 17.58 (11.74–25.03)b 28.11 (19.11–43.14) 26.33 (20.28–46.91) 0.001 0.704 0.138 IL-6, pg·mL−1 0.64 (0.31–0.96) 0.64 (0.38–0.94) 1.07 (0.38–3.58)(n = 4) 0.88 (0.66–1.23) 0.58 (0.41–0.85) 0.55 (0.41–1.07) 1.07 (0.69–1.70)a , d 0.873 0.726 0.011 CRP > 4 mg·L−1,% (N) 0 (0) 6 (1) 0 (0) 0 (0) 0 (0) 0 (0) 25 (4) 0.390 0.790 0.005 sTfR, mg·L−1 0.55(0.50–0.60) 1.06(0.90–1.40)b 2.04(1.66–2.22) 1.66(1.65–2.91) 1.84(1.21–2.37)b 2.18(1.60–2.61) 3.24(1.72–4.70)a <0.001 0.046 0.036 Ceruloplasmin, mU·mL−1 20,136(14,863–24,585) 22,495(17,781–40,676) 36,695(23,373–39,407) 25,017(19,705–27,002) 28,810(24,666–32,584)b 31,682(22,569–35,613) 25,350(21,673–29,541) 0.027 0.782 0.407 Total hemoglobin mass and intravascular volumes Hbmass, g 745(636–847) 872(811–1045) 1343(1020–1348) 1331(1319–1701) 1367(1169–1808)b , c 1577(1318–1944) 1887(1555–1981) <0.001 0.024 0.097 Hbmass, g·kg−1 10.8(9.4–11.3) 12.7(11.6–15.1)b 16.8(14.0–19.6) 22.5 (20.3–22.8)a 19.0 (18.3–24.4)b , c 21.9 (19.1–28.1) 26.7 (20.3–31.3) <0.001 0.014 0.099 RBCV, mL 2409(2035–2773) 2614(2385–3046) 4187(3199–4447) 4159(3948–5295) 4176 (3483–5791)b , c 4625(3903–5930) 5685(4667–6325) <0.001 0.012 0.103 RBCV, mL·kg−1 34.0(29.5–36.6) 38.4(34.4–43.6) 52.3(46.3–61.5) 64.4(60.3–67.9)a 59.0(55.7–78.3)b , c 65.4(57.1–83.9) 81.4(61.4–104.7) <0.001 0.006 0.145 PV, mL 2797(2517–3439) 2075(1858–2371)b 2401(2255–2907) 2201(1921–2220) 2144(1799–2442)b 2072(1847–2268) 1842(1517–2243) <0.001 0.315 0.278 PV, mL·kg−1 40.7(35.8–49.6) 28.8(27.0–33.1) 30.6(28.9–36.9) 28.3(26.4–29.3) 29.8(25.7–34.8)b , c 29.3(25.8-32.2) 27.0(20.9-32.8) <0.001 0.373 0.460 BV, mL 4866(4435–6184) 4677(4392–5462) 6442(5165–7381) 6398(5888–7515) 6355(5678–7718)b , c 6893(5901–8124) 7466(6348–8421) 0.001 0.043 0.392 BV, mL·kg−1 77.2(68.5–80.1) 68.8(62.7–78.1) 80.5(79.5–90.5) 92.7(89.6–94.3) 91.4(81.7–104.0)b , c 100.0(82.0–119.5) 110.2(93.7–127.9) <0.001 0.016 0.422 Participant characteristics of male residents at sea level, 3800 m, and 5100 m. Individuals were categorized with the CMS Qinghai score including the [Hb] criterion (CMS score ≤5 = no CMS; score of 6 to 10 = mild CMS; score > 10 = moderate-to-severe CMS).8 A modified CMS score was calculated by excluding the [Hb] criterion from the regular score and only summing the points from the seven symptoms.9 The effect of altitude was assessed with a Kruskal–Wallis test including participants without CMS. At each altitude (3800 and 5100 m), the effect of CMS severity was assessed with a Kruskal–Wallis test. In the case of significant main effect, post-hoc pairwise comparisons were performed using a Dunn’s test with Bonferroni correction. Hbmass and intravascular volumes are reported in absolute values (g and mL, respectively) and relative to body mass (g·kg−1 and mL·kg−1, respectively).aP < 0.05 vs no CMS, at a given altitude.bP < 0.05 vs sea level, among no CMS participants.cP < 0.05 vs 3800 m, among no CMS residents.dP < 0.05 vs mild CMS, at a given altitude.Values are presented as median and 25th–75th percentiles. The effects of altitude (in participants without CMS) and CMS severity (at each altitude) on systemic inflammation, that is, CRP concentration > 4 mg·L−1, were assessed using Chi-square tests.BMI = body mass index; BV = blood volume; CMS = chronic mountain sickness; CRP = C-reactive protein EPO = IL-6 and sTfR, serum concentrations of erythropoietin, interleukin 6 and soluble transferrin receptor, respectively; [Hb] = hemoglobin concentration; Hbmass = total hemoglobin mass, measured by carbon-monoxide rebreathing; PV = plasma volume; RBCV = total red blood cell volume; SpO2 = pulse oxygen saturation. Figure 1.: Excessive erythrocytosis does not alter iron homeostasis in men at 5100 m. Individual serum concentrations of iron (A), ferritin (C), hepcidin (E) and erythroferrone (F), as well as transferrin saturation (B) and calculated body iron (D), determined in sea-level residents (n = 17) and high-altitude residents at 3800 m (Puno, n = 26) and 5100 m (La Rinconada, n = 57). Lines indicate medians and 25th–75th percentiles. High-altitude residents were categorized into no CMS, mild CMS, or moderate-to-severe CMS (see Table 1 indicating the number of participants in each subgroup). The effect of altitude was assessed with a Kruskal–Wallis test including participants without CMS. At each altitude (3800 m and 5100 m), the effect of CMS was assessed with a Kruskal–Wallis test. Pairwise comparisons were performed using a Dunn’s test with Bonferroni correction and visualized with brackets above the graphs. n = 23 for ferritin and calculated body iron in the mild CMS group at 5100 m. The formula for calculating body iron is available in the Suppl. Methods. CMS = chronic mountain sickness.Also consistent with our previous report,9 similar high levels of Hbmass, RBCV and EPO were found in CMS patients and healthy individuals at 5100 m, although moderate-to-severe CMS patients displayed trends toward even higher levels (Table 1), suggesting a stronger erythropoietic response, substantiated by higher sTfR levels (Table 1). In this subpopulation, excessive erythrocytosis was accompanied by erythroferrone induction and hepcidin inhibition (not observed at lower altitude)10; however, there was no reduction in iron stores (Figure 1). The mild inflammatory status, evidenced by slightly elevated interleukin 6 (an hepcidin inducer)1 in patients with moderate-to-severe CMS at 5100 m (Table 1), seemed insufficient to counteract the decrease in hepcidin triggered by erythroferrone. To explore the effect of age on iron parameters across altitude levels and CMS status, multivariable analysis was conducted. No independent association was found between age and iron parameters (Suppl. Table S2). In healthy people ascending to high altitude, increased erythropoiesis is accompanied by massive mobilization of iron stores.2,3,7 Similarly, in erythrocytic mice constitutively overexpressing EPO systemically,11 or with gain-of-function mutation of EPO receptor,12 low hepcidin levels are associated with reduced transferrin saturation and low iron stores. Conversely, normal ferritin and transferrin levels are found in Puno residents6; similarly, Ethiopian highlanders do not show decreased body iron stores despite high hemoglobin concentration.5 These findings are consistent with an evolutionary adaptation against iron deficiency, as also suggested by the lower prevalence of iron deficiency in Sherpas than in lowlanders upon ascent to 5050 m.3 Our finding that iron stores were normal in healthy residents and CMS patients of the world’s highest city is consistent with previous data obtained at lower altitudes. The confirmation that iron stores are maintained even in the presence of excessive erythrocytosis identified via RBCV determination highlights a strong protective mechanism but also raises questions about the mechanisms that permit massive erythropoiesis without incurring iron deficiency. The EPO/erythroferrone/hepcidin/ferroportin axis, known to secure higher iron export by spleen and liver reticuloendothelial cells after RBC recycling, as well as increased iron absorption by duodenal enterocytes, was minimally affected by altitude and therefore does not appear to be involved. In fact, we showed that erythroferrone induction and hepcidin suppression were detectable only in moderate-to-severe CMS patients, who experience an even higher erythroid drive and iron demand. The unexpected lack of erythroferrone induction and hepcidin repression in healthy residents at very high altitude, despite chronically high EPO levels, may reflect very effective erythropoiesis, which is evidenced by massive RBCV expansion (Table 1). Erythroferrone primarily responds to acute erythropoietic stimulation and an expanded erythroblast population during ineffective erythropoiesis.1 Moreover, the absence of iron deficiency among high-altitude residents, including CMS patients, suggests that hepcidin downregulation may not be promoted by a loss of iron stores, as previously suggested during short-term hypoxia.4 The absence of significant losses of stored iron suggests that high-altitude residents provide iron to the bone marrow mainly by increasing absorption and/or efficiently recycling iron derived by the higher number of processed RBCs. Hypoxia may improve iron absorption in a hepcidin-independent manner by stabilizing the alpha subunit of HIF-2 and subsequently, the HIF-2 heterodimer increases expression of intestinal iron transporters, as demonstrated in healthy lowlanders acutely exposed to hypoxia.2 Similarly, SLC40A1, the hypoxia-responsive gene that encodes ferroportin,1 could be transcriptionally upregulated in macrophages, thus maintaining elevated iron export despite unaltered hepcidin levels. Ceruloplasmin, which is induced by hypoxia,13 is increased at 5100 m, suggesting an additional mechanism to achieve efficient iron mobilization, uptake, and transfer to the erythropoietic compartment. Finally, a possible mechanism uncoupling iron uptake from increasing hemoglobin levels might be that the oxygen-sensing pathway in duodenal enterocytes acts independently of the hemoglobin concentrations.14 In chronic hypoxia, these mechanisms may provide sufficient iron for steady—although very high—RBC production, reflecting an equilibrium between increased iron availability and increased iron consumption. In conclusion, male residents from the world’s highest city experience excessive erythrocytosis and massive RBCV expansion without concomitant iron deficiency, presumably since iron regulation reaches equilibrium at a different level of erythropoiesis, through optimization of iron transport mechanisms not involving the erythroferrone/hepcidin axis. Only the additional erythropoietic stress present in moderate-to-severe CMS patients induces erythroferrone and blunts hepcidin to face the even higher iron requirement for RBC production. At a functional level, very-high-altitude residents display normal iron stores in association with low prevalence of hypoxic pulmonary hypertension,15 a clinical sign of CMS.8 Since higher HIF signaling is the putative mechanism for pulmonary hypertension, and iron depletion decreases the activity of prolyl hydroxylase—the major negative regulator of HIFs—thereby exacerbating hypoxic pulmonary hypertension,7 we suggest that maintaining iron stores in those residents may help to protect their pulmonary vasculature from hypertension. ACKNOWLEDGMENTS The authors thank the study volunteers for their commitment and time invested in this study. Furthermore, thanks are extended to the Peruvian medical students who assisted in the participant recruitment and screening for this study. The authors also thank Severine Lambing and Vincent Rocher from Radiometer France for their excellent technical assistance. Finally, we wish to thank Prof. Philippe Connes for his expert contribution, and Violette Viard for helping in the manuscript’s preparation. The manuscript has been revised for the English by an independent scientific language editing service (Angloscribe). AUTHOR CONTRIBUTIONS GC, BC, SV, and PR designed the study; BC, IH, LO, ES, AP, JVB, SD, and SV performed the study; MC, EG, SR, DG, AC, A-KML, CC, DG, CL, SV, and PR contributed vital new reagents or analytical tools; BC and PR analyzed data; GC and PR wrote the paper; BC, MC, EG, SR, DG, AC, CC, DG, LO, CL, AP, JVB, SD, and SV edited and revised the manuscript; all authors approved the final version of the manuscript. DISCLOSURES The authors have no conflicts of interest to disclose. SOURCES OF FUNDING This study was supported by the Grenoble Alpes University foundation, the “Fonds de dotation AGIR pour les maladies chroniques,” the French National Research Agency (ANR-12-TECS-0010) as part of the “Investissements d’avenir” program (ANR-15-IDEX-02), and the University of Milan, project Linea 2.
更多查看译文
关键词
excessive erythrocytosis,altered iron homeostasis
AI 理解论文
溯源树
样例
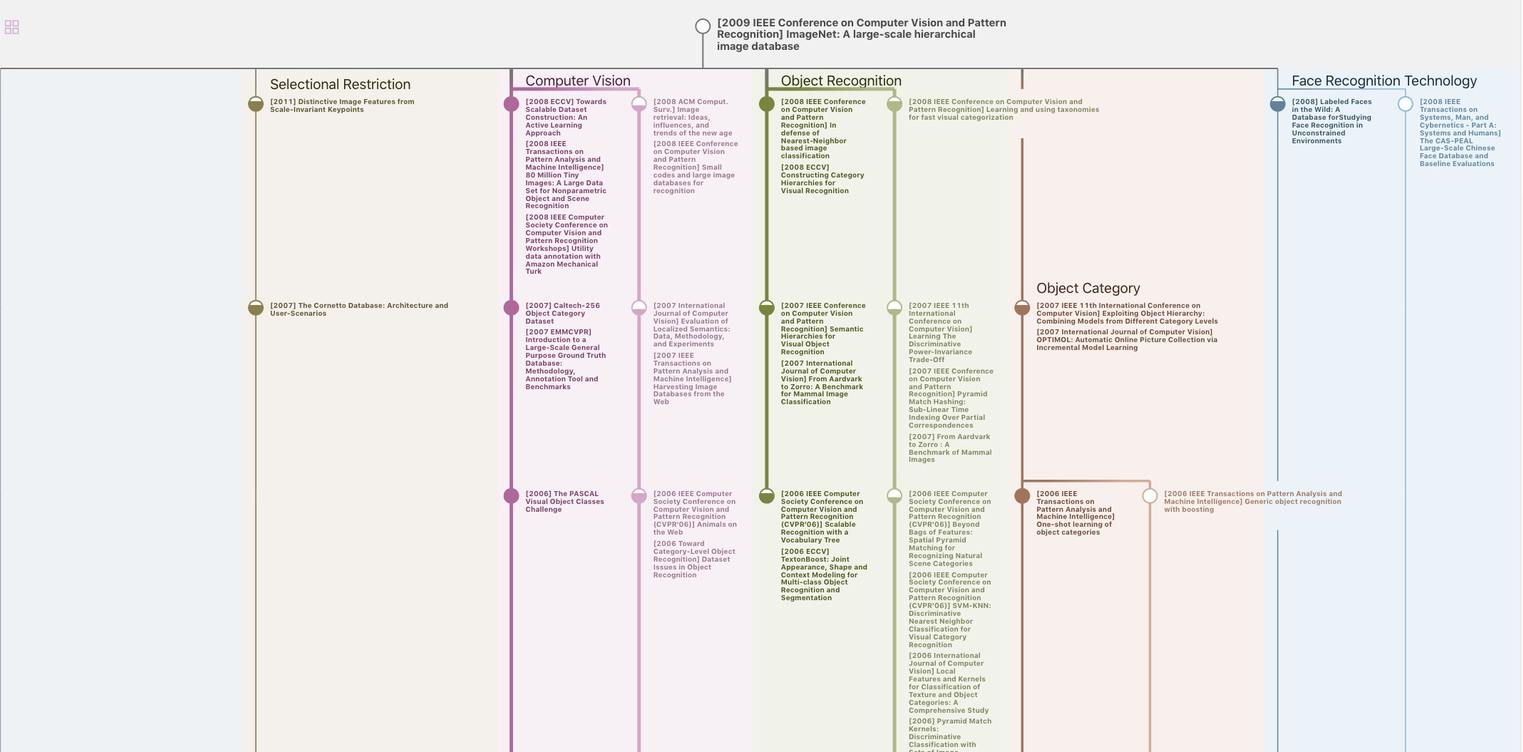
生成溯源树,研究论文发展脉络
Chat Paper
正在生成论文摘要