Mitochondrial Stress Induces an HRI-eIF2α Pathway Protective for Cardiomyopathy.
Circulation(2022)
摘要
HomeCirculationVol. 146, No. 13Mitochondrial Stress Induces an HRI-eIF2α Pathway Protective for Cardiomyopathy Free AccessLetterPDF/EPUBAboutView PDFView EPUBSections ToolsAdd to favoritesDownload citationsTrack citationsPermissions ShareShare onFacebookTwitterLinked InMendeleyReddit Jump toFree AccessLetterPDF/EPUBMitochondrial Stress Induces an HRI-eIF2α Pathway Protective for Cardiomyopathy Siting Zhu, Anh Nguyen, Jing Pang, Jun Zhao, Ze’e Chen, Zhengyu Liang, Yusu Gu, Helen Huynh, Yutong Bao, Sharon Lee, Yuval Kluger, Kunfu Ouyang, Sylvia M. Evans and Xi Fang Siting ZhuSiting Zhu https://orcid.org/0000-0002-6950-9556 Department of Cardiovascular Surgery, Peking University Shenzhen Hospital, School of Chemical Biology and Biotechnology, State Key Laboratory of Chemical Oncogenomics, Peking University Shenzhen Graduate School, Shenzhen, China (S.Z., Z.C., K.O.). Department of Medicine (S.Z., A.N., J.P., Z.C., Z.L., Y.G., H.H., Y.B., S.L., S.M.E., X.F.), University of California San Diego, La Jolla. *S. Zhu, A. Nguyen, and J. Pang contributed equally. Search for more papers by this author , Anh NguyenAnh Nguyen https://orcid.org/0000-0003-1272-6856 Department of Medicine (S.Z., A.N., J.P., Z.C., Z.L., Y.G., H.H., Y.B., S.L., S.M.E., X.F.), University of California San Diego, La Jolla. *S. Zhu, A. Nguyen, and J. Pang contributed equally. Search for more papers by this author , Jing PangJing Pang Department of Medicine (S.Z., A.N., J.P., Z.C., Z.L., Y.G., H.H., Y.B., S.L., S.M.E., X.F.), University of California San Diego, La Jolla. *S. Zhu, A. Nguyen, and J. Pang contributed equally. Search for more papers by this author , Jun ZhaoJun Zhao Department of Pathology, Yale School of Medicine, New Haven, CT (J.Z., Y.K.). Search for more papers by this author , Ze’e ChenZe’e Chen Department of Cardiovascular Surgery, Peking University Shenzhen Hospital, School of Chemical Biology and Biotechnology, State Key Laboratory of Chemical Oncogenomics, Peking University Shenzhen Graduate School, Shenzhen, China (S.Z., Z.C., K.O.). Department of Medicine (S.Z., A.N., J.P., Z.C., Z.L., Y.G., H.H., Y.B., S.L., S.M.E., X.F.), University of California San Diego, La Jolla. Search for more papers by this author , Zhengyu LiangZhengyu Liang https://orcid.org/0000-0002-3307-0959 Department of Medicine (S.Z., A.N., J.P., Z.C., Z.L., Y.G., H.H., Y.B., S.L., S.M.E., X.F.), University of California San Diego, La Jolla. Search for more papers by this author , Yusu GuYusu Gu Department of Medicine (S.Z., A.N., J.P., Z.C., Z.L., Y.G., H.H., Y.B., S.L., S.M.E., X.F.), University of California San Diego, La Jolla. Search for more papers by this author , Helen HuynhHelen Huynh Department of Medicine (S.Z., A.N., J.P., Z.C., Z.L., Y.G., H.H., Y.B., S.L., S.M.E., X.F.), University of California San Diego, La Jolla. Search for more papers by this author , Yutong BaoYutong Bao https://orcid.org/0000-0003-4021-2991 Department of Medicine (S.Z., A.N., J.P., Z.C., Z.L., Y.G., H.H., Y.B., S.L., S.M.E., X.F.), University of California San Diego, La Jolla. Search for more papers by this author , Sharon LeeSharon Lee Department of Medicine (S.Z., A.N., J.P., Z.C., Z.L., Y.G., H.H., Y.B., S.L., S.M.E., X.F.), University of California San Diego, La Jolla. Search for more papers by this author , Yuval KlugerYuval Kluger Department of Pathology, Yale School of Medicine, New Haven, CT (J.Z., Y.K.). Search for more papers by this author , Kunfu OuyangKunfu Ouyang Department of Cardiovascular Surgery, Peking University Shenzhen Hospital, School of Chemical Biology and Biotechnology, State Key Laboratory of Chemical Oncogenomics, Peking University Shenzhen Graduate School, Shenzhen, China (S.Z., Z.C., K.O.). Search for more papers by this author , Sylvia M. EvansSylvia M. Evans https://orcid.org/0000-0001-5035-3697 Department of Medicine (S.Z., A.N., J.P., Z.C., Z.L., Y.G., H.H., Y.B., S.L., S.M.E., X.F.), University of California San Diego, La Jolla. Department of Pharmacology (S.M.E.), University of California San Diego, La Jolla. Skaggs School of Pharmacy and Pharmaceutical Sciences (S.M.E.), University of California San Diego, La Jolla. Search for more papers by this author and Xi FangXi Fang Correspondence to: Xi Fang, PhD, Department of Medicine, University of California San Diego, 9500 Gilman Dr, Mail Code 0613-C, La Jolla, CA 92093. Email E-mail Address: [email protected] https://orcid.org/0000-0001-7816-8821 Department of Medicine (S.Z., A.N., J.P., Z.C., Z.L., Y.G., H.H., Y.B., S.L., S.M.E., X.F.), University of California San Diego, La Jolla. Search for more papers by this author Originally published26 Sep 2022https://doi.org/10.1161/CIRCULATIONAHA.122.059594Circulation. 2022;146:1028–1031Mitochondrial dysfunction elicits a mitochondrial stress response (MSR) through mitochondrial-nuclear communication and activates activating transcription factor 4 (ATF4), a master transcriptional regulator of the cellular stress response.1 Knowledge of consequences of MSR-triggered ATF4 activation in mitochondrial cardiomyopathy is limited yet critical for therapeutic approaches.Mitochondrial phosphatase protein tyrosine phosphatase mitochondrial 1 (Ptpmt1) cKO (PKO) mice2 provide a model to investigate in vivo mechanisms of MSR in cardiomyocytes, displaying key features of fetal mitochondrial cardiomyopathy while evidencing lethality between embryonic day (E) 16.5 and 18.5.2 All mouse protocols were approved by the Institutional Animal Care and Use Committee. Molecular analyses confirmed that loss of PTPMT1 in cardiomyocytes resulted in upregulation of ATF4 and its target genes at E11.5 (Figure [A–D]), similar to other MSR models. The most well-recognized upstream regulator of ATF4 is eukaryotic initiation factor 2α (eIF2α) phosphorylation, which increases ATF4 translation. Atf4 is also a direct target of transcriptional repression by hypoxia-inducible factor-1α (HIF1α) in cardiomyocytes. Hif1α cKO hearts display increased Atf4 mRNA and protein. The mammalian target of rapamycin (mTOR) is another upstream regulator of ATF4. We examined these upstream regulators of ATF4 in PKO hearts and found that phosphorylation of eIF2α was significantly increased in PKO versus control hearts at E11.5, whereas HIF1α and mTOR phosphorylation were not altered (Figure [E]), suggesting that eIF2α phosphorylation induced the expression of ATF4.Download figureDownload PowerPointFigure. HRI-eIF2α pathway benefits mitochondrial cardiomyopathy. A, Volcano plot obtained from DESeq2 analysis of gene expression in Ptpmt1 cardiomyocyte-specific knockout (Ptpmt1f/f; Xml-Cre+; PKO) vs Cre-negative control hearts at embryonic day E 11. 5. Genes with adjusted P<0.05 and log2 (fold change) >1 are considered significantly upregulated or downregulated genes in PKO hearts. Green indicates downregulated genes; red indicates upregulated genes. n=3 per group. RNA-sequencing data were deposited to the GEO database (Accession No. GSE201042). B, Functional clustering analysis using the Reactome database revealed that deletion of PTPMT1 in cardiomyocytes significantly upregulates pathways involved in amino acid (AA) metabolism, which is classically found to be regulated by activating transcription factor 4 (ATF4). C, Motif enrichment analysis at promoter regions (±2 kb from transcription start sites) of the genes that were upregulated in PKO hearts revealed that the top enriched transcription factor binding motifs were ATF4 and its cofactors C/EBP (CCAAT/enhancer-binding protein) or C/EBP homologous protein binding motifs. D, Quantitative reverse transcription–polymerase chain reaction (qRT-PCR) validated the upregulation of classic ATF4 target genes in PKO (red) vs control (Ctrl; blue) hearts at E11.5. n=3 to 4 per group. E, Western blot analysis of ATF4, phosphorylated eukaryotic initiation factor 2α (eIF2α) at serine 51 (Ser51), phosphorylated mammalian target of rapamycin (mTOR) at serine 2448 (Ser2448), and hypoxia-inducible factor-1α (HIF1α) in PKO and Ctrl hearts at E11.5. n=4 per group. F, Model for eIF2α-mediated translational control of ATF4 in response to diverse cellular stress responses. G, Western blot analysis of ATF4 and phosphorylated eIF2α at Ser51 and ATF4 in eIF2αS51A mutant (Ptpmt1f/f; eIF2αm/m; Xml-Cre−; S51A), PKO (Ptpmt1f/f; eIF2α+/+; Xml-Cre+), PKO/eIF2αS51A double-mutant (dMut; Ptpmt1f/f; eIF2αm/m; Xml-Cre+), and control (Ctrl; Ptpmt1f/f; eIF2α+/+; Xml-Cre−) hearts at E11.5. n=4 per group. H, qRT-PCR analysis of ATF4 target genes in S51A, PKO, dMut, and Ctrl hearts at E11.5. n=3 to 4 per group. I, Heat map representation of transcript levels of selected ATF4 target genes in S51A, PKO, dMut, and Ctrl hearts at E11.5. J, Heat map representation of transcript levels of selected endoplasmic reticulum stress–induced genes, including the target genes of ATF4, and XBP1 and ATF6 in PKO and Ctrl hearts at E11.5. K, Heat map representation of transcript levels of selected ATF4 target genes in PKO/GCN2 double-knockout (dKO; Ptpmt1f/f-Eif2ak4−/−; Xml-Cre+) and wild-type (WT) control (Ptpmt1+/+-Eif2ak4+/+; Xml-Cre−) hearts (left) and HRI-null (HKO; Ptpmt1f/f; Eif2ak4−/−; Xml-Cre−), PKO (Ptpmt1f/f; Eif2ak4+/+; Xml-Cre+), PKO/HRI dKO (Ptpmt1f/f; Eif2ak4−/−; Xml-Cre+), and Ctrl (Ptpmt1f/f; Eif2ak4+/+; Xml-Cre−) hearts (right). Note that the genes that encode GCN2 and PTPMT1 are at the same allele, so we could not obtain single-knockout littermates. L, qRT-PCR analysis of ATF4 target genes in PKO/GCN2 dKO and WT hearts at E11.5. n=5 to 7 per group. M, Western blot analysis of ATF4 and phosphorylated eIF2a at Ser51 in PKO/GCN2 dKO and WT hearts. n=4 per group. N, qRT-PCR analysis of ATF4 target genes in HKO, PKO, PKO/HRI dKO, and Ctrl hearts at E11.5. n=3 to 4 per group. O, Western blot analysis of ATF4 and phosphorylated eIF2a at Ser51 in HKO, PKO, PKO/HRI dKO, and Ctrl hearts. n=4 per group. P, Whole embryonic (top) and heart (bottom) morphology of S51A, PKO, dMut, and Ctrl hearts at E13.5. Scale bar, 1 mm. Q, Whole embryonic (top) and heart (bottom) morphology of HKO, PKO, PKO/HRI dKO, and Ctrl hearts at E14.5. Scale bar, 1 mm. R, qRT-PCR analysis of ATF4 target genes in HKO (Tazf/Y; Eif2ak4−/−; Xml-Cre−), Tafazzin cardiomyocyte-specific knockout (TKO; Tazf/Y; Eif2ak4+/+; Xml-Cre+), TKO/HRI dKO (Tazf/Y; Eif2ak4−/−; Xml-Cre+), and Ctrl (Tazf/Y; Eif2ak4+/+; Xml-Cre−) hearts at postnatal day (P) 7. n=3 to 4 per group. S, Western blot analysis of ATF4 and phosphorylated eIF2α at Ser51 in HKO, TKO, TKO/HRI dKO, and Ctrl hearts at P7. n=4 per group. T, Cardiac ventricular weight to body weight ratio for HKO, TKO, TKO/HRI dKO, and Ctrl hearts at P7. n= 5 to 7 per group. U, Whole-mount (top) and hematoxylin and eosin–stained sections (bottom) for HKO, TKO, TKO/HRI dKO, and Ctrl hearts at P8. Scale bar, 1 mm. V, Echocardiographic measurements of left ventricular percentage of fractional shortening (left), end-diastolic left ventricular internal diameter (LVIDd; middle), and end-systolic left ventricular internal diameter (LVIDs; right) for HKO, TKO, TKO/HRI dKO, and Ctrl mice at P7. n=4 to 6 mice per group. GAPDH was used as a loading control for Western blots. qRT-PCR data were normalized to corresponding 18S levels, and levels in mutants are expressed as the fold change vs Ctrl. Data are represented as mean±SEM. *P<0.05, PKO, TKO, or dMut or dKO vs Ctrl or as indicated. #P<0.05, dMut or dKO vs PKO or TKO or as indicated by 2-tailed Student t test (2-group comparison) or 2-way ANOVA (4-group comparison). AA indicates amino acid; DEG, indicates differentially expressed gene; His, histidine; Lys, lysine; Phe, phenylalanine; SLC, solute carrier superfamily; and Trp, tryptophan.Phosphorylation of eIF2α at serine 51 integrates signals from diverse cellular stress responses (Figure [F]).1 We generated a phosphorylation-resistant eIF2α-mutant allele1 in which the serine 51 phosphorylation site was mutated to alanine (eIF2αS51A) and crossed this allele into PKO mice to generate PKO/eIF2αS51A double-mutant (dMut) mice. The absence of eIF2α phosphorylation in eIF2αS51A and dMut hearts was validated (Figure [G]). ATF4 protein and downstream targets were significantly decreased to baseline levels in dMut hearts (Figure [G–I]), confirming that eIF2α phosphorylation was essential for MSR-triggered ATF4 activation in PKO hearts.Four eIF2α kinases are activated by distinct forms of stress (Figure [F]).1 RNA-sequencing analysis from PKOs indicated increased expression of ATF4 targets but no increase in XBP1 or ATF6 (Figure [J]), the latter negating the likelihood that a eukaryotic translation initiation factor 2 alpha kinase 3 (PERK)-mediated endoplasmic reticulum (ER) stress response was involved.1 Next, we generated null alleles for eukaryotic translation initiation factor 2 alpha kinase 4 (GCN2) or heme-regulated eIF-2alpha kinase (HRI) and generated PKO/GCN2 and PKO/HRI double-knockout (dKO) mice. Loss of HRI abolished activation of eIF2α-ATF4 signaling in PKOs, whereas eIF2α-ATF4 remained activated in PKO/GCN2 dKO hearts (Figure [K–O]). Thus, MSR-induced eIF2α-ATF4 signaling was dependent on HRI, and other eIF2α kinases were not involved. Our results are consistent with recent in vitro findings in HeLa cells,3,4 providing the first demonstration of the in vivo relevance of this pathway in mitochondrial cardiomyopathy. It is not clear whether activation of HRI depends on the amount of heme in cardiomyocytes.Whether the eIF2α pathway was adaptive or maladaptive remained to be addressed. Although global suppression of protein synthesis by eIF2α phosphorylation conserves energy and increasing ATF4 translation allows cells to survive periods of stress, persistent ATF4 and suppression of protein synthesis may also be detrimental. Homozygous eIF2αS51A mutants die within 18 hours after birth.1 However, constitutive activation of eIF2α by deleting both eIF2α phosphatases Ppp1r15a and Ppp1r15b results in embryonic lethality.1 Thus far, in vivo physiological consequences of eIF2α phosphorylation in response to stress have not been addressed. We analyzed the morphology and survival of PKO/eIF2αS51A dMut mice. PKO/eIF2αS51A dMut mice died at E13.5, whereas PKO mice survived at this stage with abnormal heart morphology, demonstrating that blocking eIF2α phosphorylation negatively impacted survival (Figure [P]).Although HRI-null mice were viable1 and displayed normal cardiac development and function at baseline, the role of HRI activation in mitochondrial cardiomyopathy remained to be addressed. We found that PKO/HRI dKO mice died between E14.5 and E16.5, whereas PKO littermates survived with abnormal cardiac morphology (Figure [Q]). Thus, although milder than effects of the eIF2α S51A mutant, deletion of HRI was also detrimental to survival. Thus HRI-eIF2α activation was protective for fetal mitochondrial cardiomyopathy.To determine the role of HRI in adult mitochondrial cardiomyopathy, we crossed HRI-knockout mice with Tafazzin cKO (TKO) mice. TKO mice display mitochondrial dysfunction at 2 months and dilated cardiomyopathy at 4 months, but they survive >1 year with impaired cardiac function.5 Western blot and qRT-PCR analysis confirmed that eIF2α-ATF4 signaling was activated in TKO hearts but abolished in TKO/HRI dKO hearts (Figure [R and S]). TKO/HRI dKO mice died between postnatal days 7 and 10 with enlarged hearts, compared with TKO mice that survived >1 year.5 We also observed increased ratios of ventricular weight to body weight in dKO mice (Figure [T and U]). Echocardiographic analysis revealed severe cardiac dysfunction in dKO mice (Figure [V]). Thus, MSR-triggered HRI-eIF2α was also protective for adult mitochondrial cardiomyopathy.Overall, we demonstrated that an HRI-eIF2α pathway mediated mitochondrial-nuclear communication and MSR-triggered ATF4 activation in both embryonic and adult heart. Our results uncovered a protective role for MSR-triggered HRI-eIF2α-ATF4 signaling in both fetal and adult mitochondrial cardiomyopathy. Therefore, intention to inhibit the HRI-eIF2α-ATF4 pathway in mitochondrial cardiomyopathy could be detrimental, rather than beneficial, for cardiac dysfunction.The data, analytical methods, and study materials that support the findings of this study will be available to other researchers from the corresponding authors on reasonable request. RNA-sequencing data were deposited to the GEO database (Accession No. GSE201042).Article InformationSources of FundingDr Fang is supported by National Institutes of Health grants. Dr Evans is supported by National Institutes of Health grants and the Foundation Leducq (16 CVD 03).Nonstandard Abbreviations and AcronymsATF4activating transcription factor 4dKOdouble-knockoutdMutdouble-mutanteIF2αeukaryotic initiation factor 2αHRIheme-regulated inhibitorMSRmitochondrial stress responsePKOphosphatase Ptpmt1 cKO micePTPMT1Protein Tyrosine Phosphatase Mitochondrial 1qRT-PCRQuantitative reverse transcriptase -polymerase chain reactionTKOTafazzin cKODisclosures Dr Zhao is currently employed by Guardant Health. This work is not related to her employment at Guardant Health. The other authors report no conflicts.FootnotesCirculation is available at www.ahajournals.org/journal/circ*S. Zhu, A. Nguyen, and J. Pang contributed equally.For Sources of Funding and Disclosures, see page 1031.Correspondence to: Xi Fang, PhD, Department of Medicine, University of California San Diego, 9500 Gilman Dr, Mail Code 0613-C, La Jolla, CA 92093. Email [email protected]eduReferences1. Costa-Mattioli M, Walter P. The integrated stress response: from mechanism to disease.Science. 2020; 368:eaat5314.CrossrefMedlineGoogle Scholar2. Chen Z, Zhu S, Wang H, Wang L, Zhang J, Gu Y, Tan C, Dhanani M, Wever E, Wang X, et al. PTPMT1 is required for embryonic cardiac cardiolipin biosynthesis to regulate mitochondrial morphogenesis and heart development.Circulation. 2021; 144:403–406. doi: 10.1161/CIRCULATIONAHA.121.054768LinkGoogle Scholar3. Fessler E, Eckl EM, Schmitt S, Mancilla IA, Meyer-Bender MF, Hanf M, Philippou-Massier J, Krebs S, Zischka H, Jae LT. A pathway coordinated by DELE1 relays mitochondrial stress to the cytosol.Nature. 2020; 579:433–437. doi: 10.1038/s41586-020-2076-4CrossrefMedlineGoogle Scholar4. Guo X, Aviles G, Liu Y, Tian R, Unger BA, Lin YT, Wiita AP, Xu K, Correia MA, Kampmann M. Mitochondrial stress is relayed to the cytosol by an OMA1-DELE1-HRI pathway.Nature. 2020; 579:427–432. doi: 10.1038/s41586-020-2078-2CrossrefMedlineGoogle Scholar5. Zhu S, Chen Z, Zhu M, Shen Y, Leon LJ, Chi L, Spinozzi S, Tan C, Gu Y, Nguyen A, et al. Cardiolipin remodeling defects impair mitochondrial architecture and function in a murine model of Barth syndrome cardiomyopathy.Circ Heart Fail. 2021; 14:e008289. doi: 10.1161/CIRCHEARTFAILURE.121.008289LinkGoogle Scholar Previous Back to top Next FiguresReferencesRelatedDetailsCited By Huynh H, Zhu S, Lee S, Bao Y, Pang J, Nguyen A, Gu Y, Chen C, Ouyang K, Evans S and Fang X (2023) DELE1 is protective for mitochondrial cardiomyopathy, Journal of Molecular and Cellular Cardiology, 10.1016/j.yjmcc.2022.12.003, 175, (44-48), Online publication date: 1-Feb-2023. September 27, 2022Vol 146, Issue 13 Advertisement Article InformationMetrics © 2022 American Heart Association, Inc.https://doi.org/10.1161/CIRCULATIONAHA.122.059594PMID: 36154620 Originally publishedSeptember 26, 2022 Keywordsmitochondriaheme-regulated eIF-2alpha kinasecardiomyopathyactivating transcription factor 4PDF download Advertisement SubjectsCardiomyopathyCell Signaling/Signal TransductionPhysiology
更多查看译文
关键词
activating transcription factor 4, cardiomyopathy, mitochondria, heme-regulated eIF-2alpha kinase
AI 理解论文
溯源树
样例
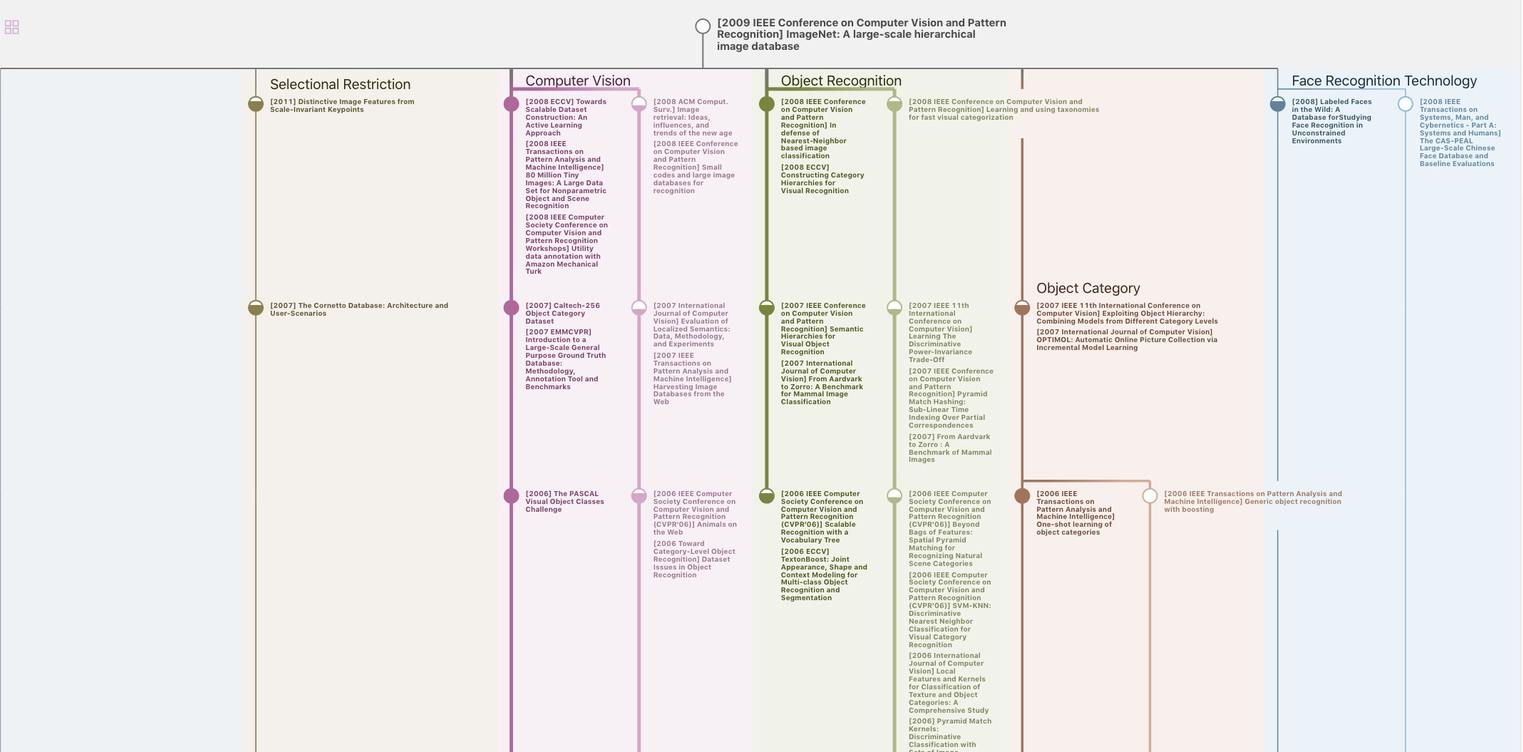
生成溯源树,研究论文发展脉络
Chat Paper
正在生成论文摘要