Abstract NG02: Polyclonal and heterogeneous resistance to targeted therapy in leukemia
Cancer Research(2015)
摘要
Genomic studies in solid tumors have revealed significant branching intratumoral clonal genetic heterogeneity. Such complexity is not surprising in solid tumors, where sequencing studies have revealed thousands of mutations per tumor genome. However, in leukemia, the genetic landscape is considerably less complex. Chronic myeloid leukemia (CML) is the human malignancy most definitively linked to a single genetic lesion, the BCR-ABL gene fusion. Genome wide sequencing of acute myeloid leukemia (AML) has revealed that AML is the most genetically straightforward of all extensively sequenced adult cancers to date, with an average of 13 coding mutations and 3 or less clones identified per tumor. In CML, tyrosine kinase inhibitors (TKIs) of BCR-ABL have resulted in high rates of remission. However, despite excellent initial response rates with TKI monotherapy, patients still relapse, including virtually all patients with Philadelphia-positive acute lymphoblastic leukemia and blast crisis CML. Studies of clinical resistance highlight BCR-ABL as the sole genetic driver in CML as secondary kinase domain (KD) mutations that prevent drug binding are the predominant mechanism of relapse on BCR-ABL TKIs. In AML, a more diverse panel of disease-defining genetic mutations has been uncovered. However, in individual patients, a single oncogene can still drive disease. This is the case in FLT3 mutant AML, in which the investigational FLT3 TKI quizartinib achieved an initial response rate of ∼50% in relapsed/refractory AML patients with activating FLT3 internal tandem duplication (ITD) mutations, though most patients eventually relapsed. Confirming the importance of FLT3 in disease maintenance, we showed that 8 of 8 patients who relapsed on quizartinib did so due to acquired drug-resistant FLT3 KD mutations. Studies in CML have revealed that sequential TKI therapy is associated with additional complexity where multiple mutations can coexist separately in an individual patient (“polyclonality”) or in tandem on a single allele (“compound mutations”). In AML, we observed polyclonal FLT3-ITD KD mutations in 2 of 8 patients examined in our initial study of quizartinib resistance. In light of the polyclonal KD mutations observed in CML and AML at the time of TKI relapse, we undertook next generation sequencing studies to determine the true genetic complexity in CML and AML patients at the time of relapse on targeted therapy. We used Pacific Biosciences RS Single Molecule Real Time (SMRT) third generation sequencing technology to sequence the entire ABL KD or the entire FLT3 juxtamembrane and KD on a single strand of DNA. Using this method, we assessed a total of 103 samples from 79 CML patients on ABL TKI therapy and 36 paired pre-treatment and relapse samples from 18 FLT3-ITD+ AML patients who responded to investigational FLT3 TKI therapy. In CML, using SMRT sequencing, we detected all mutations previously detected by direct sequencing. Of samples in which multiple mutations were detectable by direct sequencing, 85% had compound mutant alleles detectable in a variety of combinations. Compound mutant alleles were comprised of both dominant and minor mutations, some which were not detectable by direct sequencing. In the most complex case, 12 individual mutant alleles comprised of 7 different mutations were identified in a single sample. For 12 CML patients, we interrogated longitudinal samples (2-4 time points per patient) and observed complex clonal relationships with highly dynamic shifts in mutant allele populations over time. We detected compound mutations arising from ancestral single mutant clones as well as parallel evolution of de novo polyclonal and compound mutations largely in keeping with what would be expected to cause resistance to the second generation TKI therapy received by that patient. We used a phospho-flow cytometric technique to assesses the phosphorylation status of the BCR-ABL substrate CRKL in as a method to test the ex vivo biochemical responsiveness of individual mutant cell populations to TKI therapy and assess functional cellular heterogeneity in a given patient at a given timepoint. Using this technique, we observed co-existing cell populations with differential ex vivo response to TKI in 2 cases with detectable polyclonal mutations. In a third case, we identified co-existence of an MLL-AF9 containing cell population that retained the ability to modulate p-CRKL in response to BCR-ABL TKIs along with a BCR-ABL containing only population that showed biochemical resistance to all TKIs, suggesting the co-existence of BCR-ABL independent and dependent resistance in a single patient. In AML, using SMRT sequencing, we identified acquired quizartinib resistant KD mutations on the FLT3-ITD (ITD+) allele of 9 of 9 patients who relapsed after response to quizartinib and 4 of 9 patients who relapsed after response to the investigational FLT3 inhibitor, PLX3397. In 4 cases of quizartinib resistance and 3 cases of PLX3397 resistance, polyclonal mutations were observed, including 7 different KD mutations in one patient with PLX3397 resistance. In 7 quizartinib-resistant cases and 3 PLX3397-resistant cases, mutations occurred at the activation loop residue D835. When we examined non-ITD containing (ITD-) alleles, we surprisingly uncovered concurrent drug-resistant FLT3 KD mutations on ITD- alleles in 7 patients who developed quizartinib resistance and 4 patients with PLX3397 resistance. One additional PLX3397-resistant patient developed a D835Y mutation only in ITD- alleles at the time of resistance, suggesting selection for a non-ITD containing clone. All of the individual substitutions found on ITD- alleles were the same substitutions identified on ITD+ alleles for each individual patient. Given that the same individual mutations found on ITD- alleles were also found on ITD+ alleles, we sought to determine whether these mutations were found in the same cell or were indicative of polyclonal blast populations in each patient. To answer this question, we performed single cell sorting of viably frozen blasts from 3 quizartinib-resistant patients with D835 mutations identified at the time of relapse and genotyped single cells for the presence or absence of ITD and D835 mutations. This analysis revealed striking genetic heterogeneity. In 2/3 cases, polyclonal D835 mutations were found in both ITD+ and ITD- cells. In all cases, FLT3-ITD and D835 mutations were found in both heterozygous and homozygous combinations. Most surprisingly, in all 3 patients, approximately 30-40% of FLT3-ITD+ cells had no identified quizartinib resistance-causing FLT3 KD mutation to account for resistance, suggesting the presence of non-FLT3 dependent resistance in all patients. To determine that ITD+ cells lacking FLT3 KD mutations observed in patients relapsed on quizartinib are indeed consistent with leukemic blasts functionally resistant to quizartinib and do not instead represent a population of differentiated or non-proliferating cells, we utilized relapse blasts from another patient who initially achieved clearance of bone marrow blasts on quizartinib and developed a D835Y mutation at relapse. We performed a colony assay in the presence of 20nM quizartinib. As expected, this dose of quizartinib was unable to suppress the colony-forming ability of blasts from this relapsed patient when compared to DMSO treatment. Genotyping of individual colonies grown from this relapse sample in the presence of 20nM quizartinib again showed remarkable genetic heterogeneity, including ITD+ and ITD- colonies with D835Y mutations in homozygous and heterozygous combinations as well as ITD+ colonies without D835Y mutations, again suggesting the presence of blasts with non-FLT3 dependent resistance. Additionally, 4 colonies with no FLT3 mutations at all were identified in this sample, suggesting the presence of a quizartinib-resistant non-FLT3 mutant blast population. To see if we could identify specific mechanisms of off-target resistance, we performed targeted exome sequencing 33-AML relevant genes from relapse and pre-treatment DNA from all four patients and detected no new mutations in any genes other than FLT3 acquired at the time of disease relapse. Clonal genetic heterogeneity is not surprising in solid tumors, where multiple driver mutations frequently occur, but in CML and FLT3-ITD+ AML, where disease has been shown to be exquisitely dependent on oncogenic driver mutations, our studies suggest a surprising amount of clonal diversity. Our findings show that clinical TKI resistance in these diseases is amazingly intricate on the single allele level and frequently consists of both polyclonal and compound mutations that give rise to an complicated pool of TKI-resistant alleles that can change dynamically over time. In addition, we demonstrate that cell populations with off-target resistance can co-exist with other TKI-resistant populations, underscoring the emerging complexity of clinical TKI resistance. Such complexity argues strongly that monotherapy strategies in advanced CML and AML may be ultimately doomed to fail due to heterogeneous cell intrinsic resistance mechanisms. Ultimately, combination strategies that can address both on and off target resistance will be required to effect durable therapeutic responses. Citation Format: Catherine C. Smith, Amy Paguirigan, Chen-Shan Chin, Michael Brown, Wendy Parker, Mark J. Levis, Alexander E. Perl, Kevin Travers, Corynn Kasap, Jerald P. Radich, Susan Branford, Neil P. Shah. Polyclonal and heterogeneous resistance to targeted therapy in leukemia. [abstract]. In: Proceedings of the 106th Annual Meeting of the American Association for Cancer Research; 2015 Apr 18-22; Philadelphia, PA. Philadelphia (PA): AACR; Cancer Res 2015;75(15 Suppl):Abstract nr NG02. doi:10.1158/1538-7445.AM2015-NG02
更多查看译文
AI 理解论文
溯源树
样例
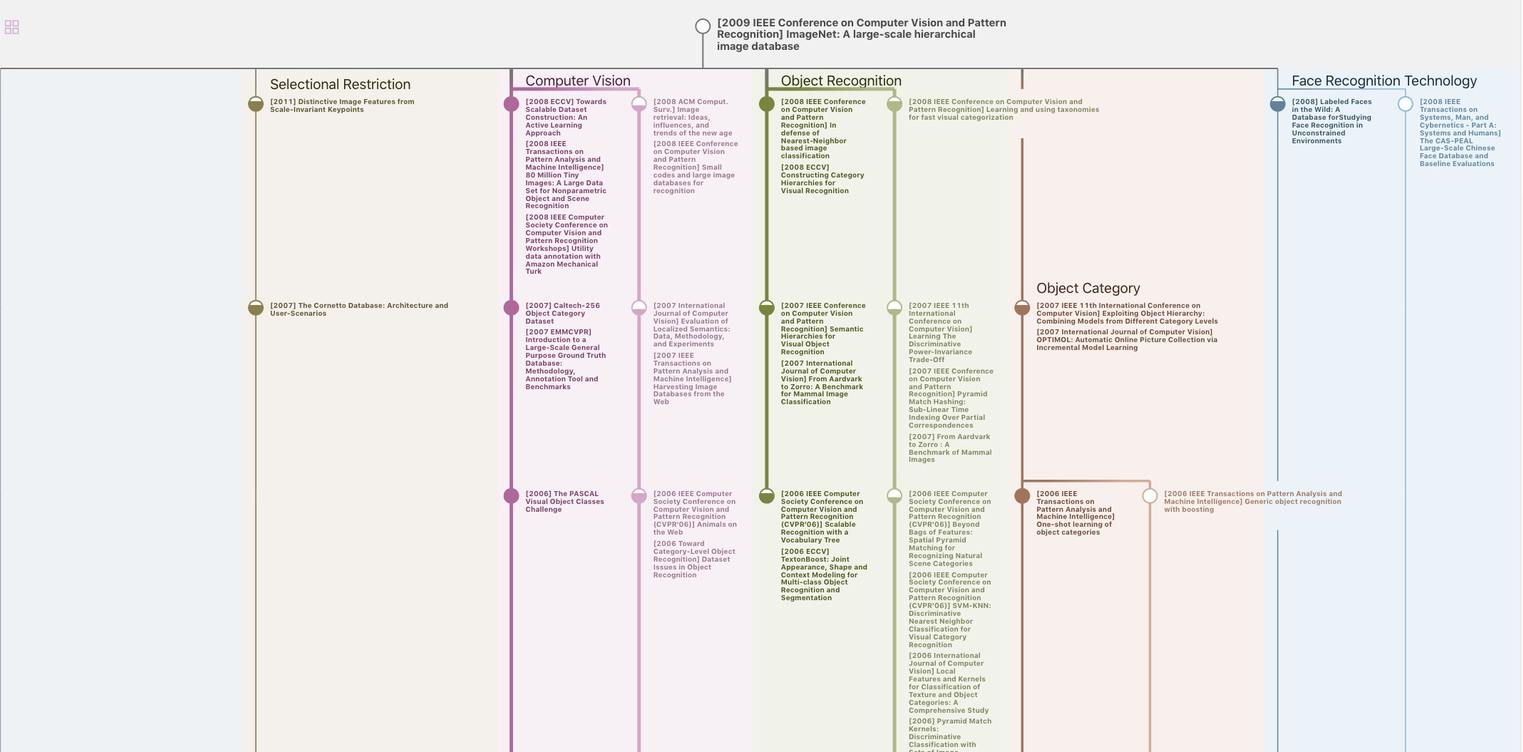
生成溯源树,研究论文发展脉络
Chat Paper
正在生成论文摘要