Redox Behavior of a CuF2/Cu Electrode for an All-Solid-State Fluoride Shuttle Battery
ECS Meeting Abstracts(2020)
摘要
IntroductionThe popularization of electrified vehicles connected to the smart grid community systems with stationary energy storage has attracted much attention as one of the solutions to global environmental problems. The critical technologies for such systems are rechargeable batteries with energy densities higher than that of conventional Li-ion batteries (LIBs). Among various candidates, all-solid-state fluoride-ion batteries (FIBs) operated by fluoride-ion transport [1] are promising owing to an ultra-high theoretical energy density over 5000 Wh/L [2].The FIBs currently we refer to as Fluoride Shuttle Batteries (FSBs) [3] were previously reported as a primary battery [4]. For the development of rechargeable FSBs, both electrolytes with high fluoride-ion conductivity and active materials that undergo reversible fluorination/de-fluorination reactions are crucial. Fluorite- and tysonite-type fluorides have been investigated as potential solid electrolytes since around the 1970s [5, 6]. Recently, our group reported a series of PbxSn2-xF4 (x = 0.96~1.52) compounds [7] with a unique structure consisting of two alternating layers; a double Pb layer and a triple-layer, each flanked by a single Sn layer. The highest conductivity of 1.1 × 10-1 S/cm at 140 °C was achieved for fluorite-type Pb1.2Sn0.8F4 (x = 1.2). As for tysonite-type fluoride, Reddy et al. reported a possible rechargeable FIB using a La0.9Ba0.1F2.9 solid electrolyte [1] in combination with the various metal fluoride active materials such as CuF2, BiF3, and KBiF4. Among many metal fluorides, CuF2/Cu is a fascinating redox couple as the cathode active material owing to its large theoretical capacity (843 mAh/g) and high electro-motive-force (3.5 V vs. Li/Li+) [8]. However, full utilization of their theoretical capacity has not yet been demonstrated. In this study, we investigated the possible rate-determining steps in the CuF2/Cu cathode reaction using Pb1.2Sn0.8F4 (x = 1.2) with a high fluoride-ion conductivity.ExperimentalThe Pb1.2Sn0.8F4 electrolyte was prepared by solid-state reaction using a mixture of PbF2 and SnF2 powders [7]. The fluoride-ion conductivity was measured by electrochemical impedance spectroscopy. The electrochemical cells were prepared by pressing into a 10 mm diameter pellet. Here, the cathode material was assembled using Cu, Pb1.2Sn0.8F4, and C in a 25:70:5 wt% ratio to facilitate both electronic and ionic conduction. Charge/discharge measurements were performed at 140 °C. Structural and electrochemical characterizations were conducted using TEM/SAED and operando XAFS.Results and DiscussionThe initial charge/discharge curves at 140 °C for the Cu cathode versus the PbF2 anode are shown in Fig. 1. The Cu cathode with Pb1.2Sn0.8F4 electrolyte exhibited a large charge (discharge) capacity of 522 (342) mAh/g. This capacity corresponds to 62 (41) % of the theoretical specific capacity even though the ionic conductivity of Pb1.2Sn0.8F4 was approximately 1000 times higher than that of La0.9Ba0.1F2.9 [1]. The Cu K-edge XAFS measurements during the charging process revealed the oxidation of Cu from Cu0 to Cu2+, and vice versa during the discharging process. The isosbestic points observed for all the spectra indicated that the conversion reaction involved the two phases, in agreement with the results of our DFT calculations [9]. In the TEM/SAED patterns of the cathode after charge, the distinct diffraction spots related to the CuF2 phase were observed on the surface of the metallic Cu particle. The fluoride-ion conductivity of CuF2 was 10-11 S/cm at 140 °C, which was very low compared to Pb1.2Sn0.8F4 and La0.9Ba0.1F2.9. These results suggest that the solid electrolyte may not be a dominant factor in the reaction on the cathode side, and alternatively smooth fluoride-ion diffusion inside the Cu active material plays a key role for redox behavior. As a next step, it may be effective to make the Cu active material a nano-composite with other fluorides, which is currently underway.AcknowledgmentsThis work was supported by the New Energy and Industrial Technology Development Organization (NEDO) of Japan under the RISING and RISING2 projects.References[1] M. A. Reddy et al., J. Mater. Chem. 21, 17059 (2011).[2] D. T. Thieu et al., Adv. Funct. Mater. 27, 1701051 (2017).[3] K. Okazaki et al., ACS Energy Lett. 2, 1460 (2017).[4] J. Schoonman et al., J. Electrochem. Soc. 123, 1772 (1976).[5] J. M. Reau et al., Mat. Res. Bull.13, 877 (1978)[6] T. Takahashi et al., J. Electrochem. Soc. 124, 280 (1977).[7] M. Murakami et al., Chem. Mater. 31, 7704 (2019).[8] C. X. Zu et al., Energy Environ. Sci. 4, 2614 (2011).[9] J. Haruyama et al., (in revision)Figure 1
更多查看译文
AI 理解论文
溯源树
样例
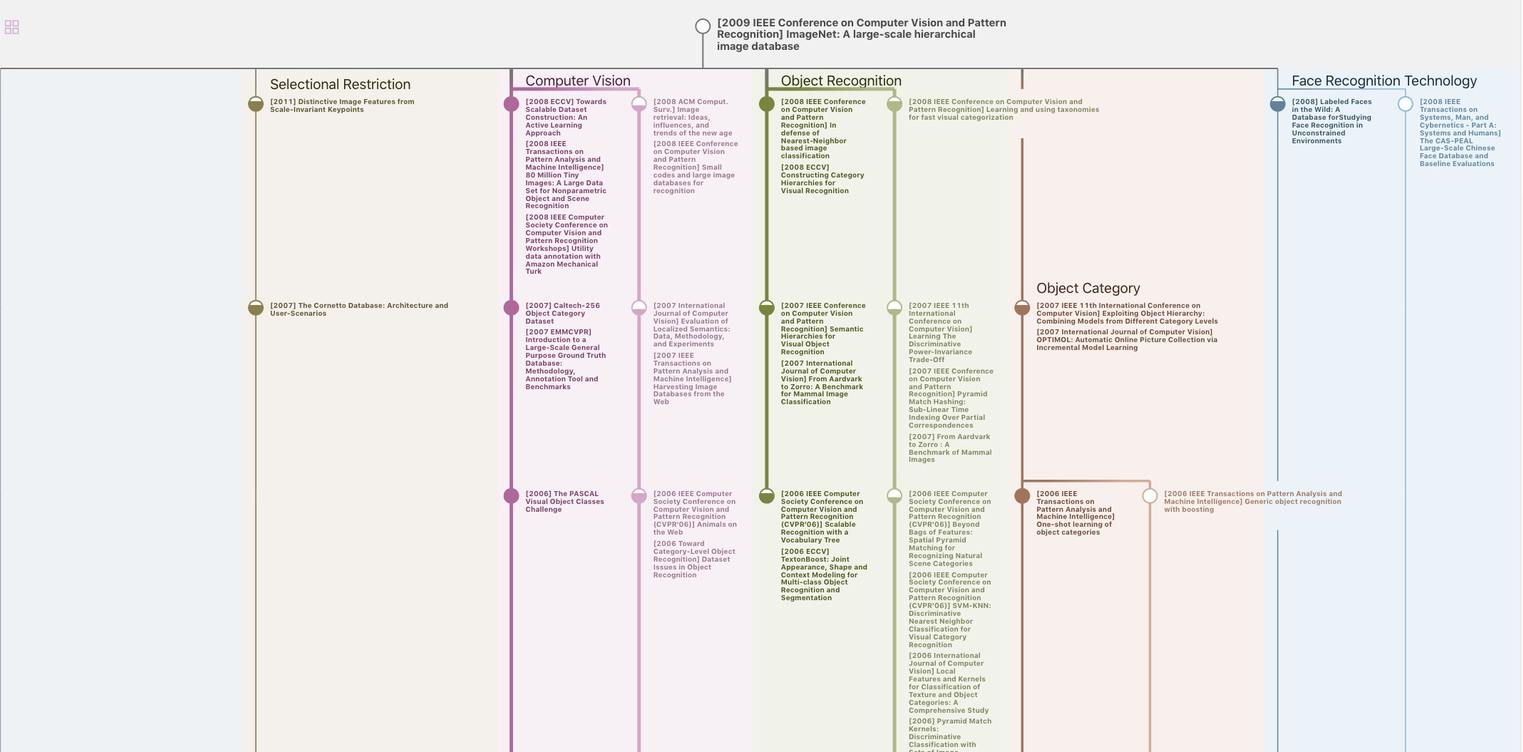
生成溯源树,研究论文发展脉络
Chat Paper
正在生成论文摘要