Research Spotlight
Regenerative Medicine(2011)
摘要
Regenerative MedicineVol. 6, No. 6s Research SpotlightFree AccessResearch SpotlightNissim Benvenisty, Stephen Dalton, Lorraine Young, Mark Sussman & M William LenschNissim BenvenistyProfessor of Genetics, The Herbert Cohn Chair in Cancer Research; Director, Stem Cell Unit, Institute of Life Sciences, The Hebrew University of Jerusalem, Jerusalem, IsraelSearch for more papers by this author, Stephen DaltonProfessor, Biochemistry and Molecular Biology, University of Georgia, GA, USASearch for more papers by this author, Lorraine YoungWolfson Centre for Stem cells, Tissue Engineering and Modelling (STEM), University of Nottingham, Nottingham, UKSearch for more papers by this author, Mark SussmanDistinguished Professor of Biology, SDSU Heart Institute, San Diego State University, San Diego, CA , USASearch for more papers by this author & M William LenschInstructor in Pediatrics, Harvard Medical School, Boston, MA USASearch for more papers by this authorPublished Online:14 Oct 2011https://doi.org/10.2217/rme.11.88AboutSectionsPDF/EPUB ToolsAdd to favoritesDownload CitationsTrack CitationsPermissionsReprints ShareShare onFacebookTwitterLinkedInRedditEmail The ability to reprogram somatic cells into induced pluripotent stem (iPS) cells is changing stem cell research, and facilitating the potential use of stem cells in regenerative medicine. These iPS cells are very similar to embryonic stem cells (ESCs). However, the past 2 years have revealed mounting evidence for potential differences between the two cell types in their gene-expression profile, differentiation ability, tumorigenic capacity, immunogenicity, regulation of imprinted genes and disease modeling. Both mouse and human iPS cells were suggested to carry an epigenetic memory from their donor cells, and this memory may affect their differentiation abilities. In addition to the potential epigenetic differences between ESCs and iPS cells, the two cell types seem to carry genomic differences. It has been suggested that the reprogramming step may involve genomic changes identified at the levels of chromosomes, copy number variations and even point mutations. Such differences may further affect the differentiation and tumorigenic characteristics of the two cell types. Although ESCs are considered the ‘gold standard’ for pluripotent stem cells, the differences between ESCs and iPS cells should not limit the use of iPS cells, and for some aspects of disease modeling and differentiation capacity, such differences may be proven beneficial.The biggest development in the past year is the realization that cells can be transdifferentiated from one state to another without going back to a pluripotent state. While this principle was previously supported by a few isolated examples, it has only been over the last year that this idea has received widespread acceptance. Numerous reports have described transdifferentiation of skin fibroblasts to neural progenitors, cardiomyocytes, hepatocytes and multilineage blood progenitors. If made more efficient, transdifferentiation could be a viable alternative to human ESC- and iPS cell-generated cell lineages.Human pluripotent stem cells are gradually providing more and more clinically relevant cell types for regenerative medicine research and development. Major progress was made by Oldershaw et al. from the University of Manchester (UK) this year, who have achieved the first efficient generation of human pluripotent stem cell-derived chondrogenic cells that are of interest because of their ability to promote cartilage repair [1]. By applying an understanding of the signaling molecules and extracellular matrix components involved in generating chondrocytes from pluripotent stem cells in the developing embryo, high-efficiency production of immature chondrocytes has been achieved. The Manchester team, led by Professor Sue Kimber, have focused on chemically defined media and a scalable monolayer-based differentiation protocol that may be particularly amenable to high-throughput strategies for discovering drugs that could enable, ultimately, cartilage repair in vivo.Bibliography1 Oldershaw RA, Baxter MA, Bates N et al. Directed differentiation of human embryonic stem cells toward chondrocytes. Nat. Biotech.28,1187–1194 (2011).Crossref, Google ScholarIdentification of lung stem cells from human tissue samples capable of regenerating the highly complex and specialized structures of the mature lung is a breakthrough in the field of lung biology and regenerative medicine. Results presented by the Anversa group in the New England Journal of Medicine demonstrate that human lung stem cells can be expanded in vitro and also retain the capacity to integrate into adult tissue upon introduction into mice [1]. This study has opened up an entirely new field of possibilities for lung regeneration and potential therapeutic application for many conditions where treatment options are either very limited or nonexistent. Despite the inevitable debate whenever a paradigm shift occurs, the findings of this study are simply too profound and the implications too important and, as such, deserve to be recognized as one of the most important contributions to the field of regenerative medicine to date.Bibliography1 Kajstura J, Rota M, Hall SR et al. Evidence for human lung stem cells. N. Engl. J. Med.364(19),1795–1806 (2011).Crossref, Medline, CAS, Google ScholarThe central goal of biomedical research is the improvement of clinical practice via the creation of new approaches to the management of human disease, as well as the refinement of existing therapies. Considering the latter, one of the areas that I have been most excited by in recent years is work seeking to functionally expand the fractional percentage of hematopoietic stem cells (HSCs) in a given cellular inoculum.The major limitation in the use of blood stem cell transplants, whether from marrow, mobilized peripheral blood or the infusion of cord blood, is donor/recipient histocompatibility. A second limitation relates to the clinical scale of a given cellular graft, that is, are there enough cells available to do the job? While the blood stem cell is defined, in part, by its ability to recapitulate the entirety of the hematopoietic system from a single input cell, the ability of a single HSC to ‘rescue’ a myeloablated patient is impossible in the clinical setting. In order to restore blood-forming activity in a conditioned patient, a great many input HSCs, progenitors and mature cells, such as red blood cells and platelets, are required if the patient is to survive. When considering the use of cord blood as a source of HSCs, clinical scale has been a major stumbling block, as while a given unit of cord blood contains enough HSCs to transplant a child, a single unit does not typically contain sufficient cells to successfully transplant an adult, although multicord transplants do offer a greater probability of engraftment.Work by groups including Leonard Zon’s laboratory seeks to define conditions wherein HSCs may be expanded in a given unit of cord blood. Here, the Zon laboratory first showed in 2007 that a single drug, prostaglandin E2 (PGE2), could functionally increase long-term repopulating HSCs on the order of about four times [1]. While four times is not a degree of amplification bound to raise many eyebrows for individuals used to seeing amplification effects of 100 times in their experiments, four times more cells means that a given unit can be used to transplant an individual significantly larger than a child – in other words, an adult. This is a huge breakthrough. The laboratory has additionally worked to refine understanding of the molecular mechanisms of this expansion [2]. Recently, Trista North (now working independently) published compelling data on the effective use of PGE2 in a preclinical, nonhuman primate model system [3]. This is very good news indeed for the future of clinical HSC transplantation.Financial & competing interests disclosureThe authors have no relevant affiliations or financial involvement with any organization or entity with a financial interest in or financial conflict with the subject matter or materials discussed in the manuscript. This includes employment, consultancies, honoraria, stock ownership or options, expert testimony, grants or patents received or pending, or royalties. No writing assistance was utilized in the production of this manuscript.Bibliography1 North TE, Goessling W, Walkley CR et al. Prostaglandin E2 regulates vertebrate haematopoietic stem cell homeostasis. Nature447(7147),1007–1011 (2007).Crossref, Medline, CAS, Google Scholar2 Goessling W, North TE, Loewer S et al. Genetic interaction of PGE2 and Wnt signaling regulates developmental specification of stem cells and regeneration. Cell136(6),1136–1147 (2009).Crossref, Medline, CAS, Google Scholar3 Goessling W, Allen RS, Guan X et al. Prostaglandin E2 enhances human cord blood stem cell xenotransplants and shows long-term safety in preclinical nonhuman primate transplant models. Cell Stem Cell8(4),445–458 (2011).Crossref, Medline, CAS, Google ScholarFiguresReferencesRelatedDetails Vol. 6, No. 6s Follow us on social media for the latest updates Metrics Downloaded 348 times History Published online 14 October 2011 Published in print November 2011 Information© Future Medicine LtdPDF download
更多查看译文
关键词
research,spotlight
AI 理解论文
溯源树
样例
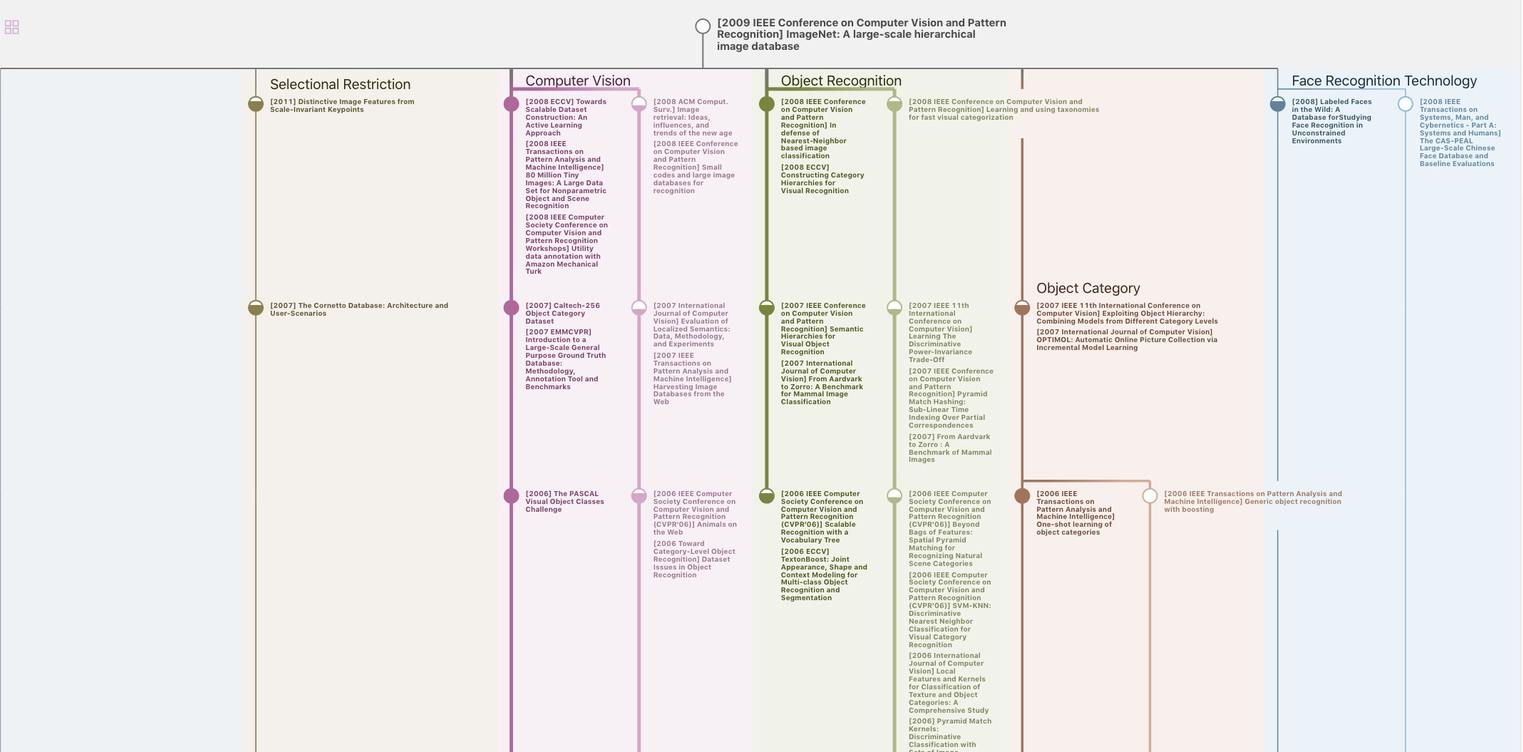
生成溯源树,研究论文发展脉络
Chat Paper
正在生成论文摘要