Chemoselective Transfer Hydrogenation Of Flavoring Unsaturated Carbonyl Compounds Over Zr And Hf-Based Metal-Organic Frameworks
BULLETIN OF THE KOREAN CHEMICAL SOCIETY(2021)
摘要
Chemoselective transfer hydrogenation of α,β-unsaturated carbonyl compounds into allylic alcohols over Zr/Hf-based MOF-808. The chemoselective hydrogenation of a carbonyl group in multiunsaturated aldehydes and ketones is a difficult task1 because hydrogenation of CC bonds is favored thermodynamically over CO bonds.2 Allylic alcohols are mostly used in the fine chemical industry for the production of pharmaceuticals, flavors, perfumes, and cosmetics.3-5 Many unsaturated carbonyls, which possess aroma and flavoring properties, can be produced using extraction from plant sources. However, the supply and the quality of traditional natural-flavor and fragrance chemicals are limited.6 Currently, around 90% of newly introduced fragrance ingredients are synthetic aroma chemicals.7 Benzaldehyde, cinnamaldehyde, citral, and carvone are widely used as reference unsaturated aldehydes. Cinnarizine, the cardiovascular drug, is derived from cinnamyl alcohol,8 whereas geraniol and nerol (derived from citral) are useful for the production of perfumes, food flavors, and insecticides.9 Vanillin is the most widely used flavor compound in the world and lignin, a major component of lignocellulosic biomass, could be utilized for the production of vanillin.10 Homogeneous Ru-phosphine-diamine catalyst11 and Rh (I) complexes12 showed excellent performance for chemoselective reduction of these carbonyl compounds using molecular H2. However, the requirement for an extra-base for its activation, difficulties in recovery, and loss in catalytic activity in recycling convinced many researchers to use heterogeneous catalysts. Typically, the reductions of these carbonyls were performed under high-pressure molecular H2 with supported metal nanoparticle catalysts such as Ni, Pt, Pd, Ru, and Rh.13 The process is complex because olefins are often preferentially reduced to form major products (saturated aldehydes or ketones). Therefore, tremendous efforts have been made to overcome this problem over the past decades. The alternative method used for chemoselective hydrogenation of an α, β-unsaturated aldehyde or ketone is catalytic transfer hydrogenation. The reaction occurs through the procedure called Meerwein–Ponndorf–Verley reduction (MPV).14 Typically mild reaction conditions are applied for MPV reduction to minimize the risk of reducing other functional groups or unsaturated CC bonds. In the last few years, zirconium-based catalysts have been attracting interest in this regard.15-18 Hydrous zirconia proved to be a good catalyst for the catalytic transfer hydrogenation (CTH) reaction of cinnamaldehyde and gave a yield of 78% cinnamyl alcohol.15 Moreover, transformation could be achieved under flow conditions.19 Isomorphous substituted “Zr” in the framework of zeolite Beta (Zr-Beta) with only a small amount of Zr, achieved 96% cinnamyl alcohol yield from cinnamaldehyde.20 In recent years, MOFs and MOF-derived materials have been used successfully as heterogeneous catalysts in several chemical transformations.21-24 Consequently, Zr-containing MOFs have recently been tested for CTH of various carbonyl compounds.25-27 Our research group has also contributed to the successful transformation of various biomass-derived carbonyl compounds using series of Zr-based functional and metal node-modified MOFs under mild reaction conditions.28, 29 Acid–base properties originated from metal nodes regarded as active sites for the CTH reaction using Zr-MOFs. Herein, we report the results of our investigation on the effect of metal sites in MOF-808, a highly active catalyst for the CTH reaction. Hf-MOF-808 was tested along with Zr-MOF-808 for the chemoselective reduction of α, β-unsaturated carbonyl compounds. Five different flavoring compounds were selected considering their huge potential in the flavor and aroma industries. The synthesized Zr- and Hf-MOF-808 was initially characterized using powder XRD. All structural peaks in the synthesized MOFs were well matched with their simulated patterns (Figure 1(a)).30 This indicated their highly crystalline nature. Thermal gravimetric measurements were performed to evaluate the thermal stability and degradation patterns in the synthesized Zr- and Hf-MOF-808 catalysts. Figure 1(b) shows that three different weight-loss patterns for both the MOFs can be observed. These correspond to physisorbed water (around 100 °C), removal of formate, μ3-OH, and solvent molecules (DMF) in the region of 250–350 °C, and structural degradation that happened due to the elimination of an organic linker (BTC) in the 400–550 °C region. Porous properties were determined using N2 physisorption isotherms measured at 77 K (Figure 1(c)). Both these MOFs showed multistep N2 adsorption features representing microporous as well as near mesoporous properties. The porous properties of these MOFs, along with the metal composition determined from ICP are summarized in the Supporting Information Table S1. The brunauer-emmett-teller surface area of Zr-MOF-808 is better (1407 m2/g) than that of Hf-MOF-808 (1047 m2/g); however, a similar trend has been observed previously in the literature.31 The pore-size distribution calculated from the density-functional theory method (Figure 1(d)) showed that Zr-MOF-808 possesses bigger pores (inner pore diameter 12.7 Å and 18.6 Å for tetrahedral and adamantine cages, respectively) than those of Hf-MOF-808 (11.8 Å and 15.9 Å). This implies that these MOFs are very porous materials. Acid–base sites in the Zr-MOFs were already proved as an important aspect of the CTH reactions. These sites in Zr-MOFs are generated from the metal node, which possesses ZrOZr bonding and was well discussed in our previous work.28, 29 Therefore, acid and base sites were measured using NH3 and CO2 temperature-programmed desorption (TPD), respectively (Supporting information Figure S1). Furthermore, Corma et al. have also reported the superior Lewis acidity of Hf-MOF-808 relative to that of Zr-MOF-808 using fourier-transform infrared (FTIR) with CD3CN as a probe molecule,32 whereas Farha et al. evaluated the acidity of Zr and Hf-based MOFs using potentiometric acid–base titration.33 Zirconium and hafnium-based MOF-808 catalysts can selectively convert these unsaturated carbonyl compounds into their respective alcohols via transfer hydrogenation that utilizes secondary alcohol (isopropanol) as a hydrogen source (Scheme 1). No subsequent reduction of CC has been observed to produce saturated alcohols confirmed from GC analysis; therefore, the reaction proceeds with high chemoselectivity toward CO groups to produce allylic alcohols. Table 1 summarizes the results obtained from the CTH reaction of five substrate molecules over Zr- and Hf-MOF-808 with different time intervals at 82 °C to compare the catalytic activity of the MOFs tested here. The CTH reaction is highly dependent on the electronic structure of the substrate molecules, as is evident from the different reaction rates for different molecules. Citral and cinnamaldehyde, which do not have aromatic α-carbon can readily and quantitatively convert into respective alcohols in 2 h of reaction time. On the other hand, both benzaldehyde and vanillin have aromatic α-carbon. Among these two compounds, vanillin took a longer reaction time (5 h) to produce a nearly quantitative yield of vanillyl alcohol due to the presence of electron donating groups on the aromatic ring. The electronic effect of these substituted groups could lead to an increase in electron density around carbonyl carbons and, subsequently, hydride ion attack from the alcohols hindered. This hampered the reaction rate. Moreover, the hydrogenation of ketone (carvone) is slower than that of aldehydes. It could be attributed to a steric hindrance to forming a six-membered cyclic ring involving a carbonyl group, IPA and metal node from MOF (which is a transition state that transfers a hydride ion from IPA to a carbonyl group) on the catalyst surface. Comparing the catalytic activity of both Zr- and Hf-MOF-808 toward all five substrates, it could be determined that Hf-MOf-808 has higher activity than Zr-MOF-808. At the maximum conversion, the two catalysts showed not much difference in conversions or in selectivity; however, their real reaction rate could be judged by comparing them at lower conversion levels. A similar trend was observed in all the CTH reactions carried out here. Furthermore, under the current applied reaction conditions, equilibrium was reached for the carvone after reaching ~70% conversion. Although a slight augmentation in conversion was observed using Hf-MOF-808 by continuing the reaction for 12 h (Supporting Information Figure S2). The CTH reaction proceeds via MPV reduction, which is a reverse equilibrium reaction called Oppenauer oxidation. The differences in catalytic activity of Hf and Zr catalysts observed here may be caused by a greater electron affinity for Hf, causing stronger binding to reactants and translating to a lower apparent activation energy. Koehle et al. studied the MPV reduction reaction of furfural over Sn, Zr, and Hf containing siliceous zeolite Beta.34 They found that activation energy for Hf-Beta (49.6 kJ/mol) was much smaller than that of Sn- and Zr-Beta zeolites (60.7 kJ/mol and 60.4 kJ/mol, respectively). Accordingly, a similar trend was observed for catalytic activity, where Hf-Beta showed better performance over Sn- and Zr-Beta catalysts. Despite the similar ionic radii of Zr and Hf (0.73 Å and 0.72 Å), the better performance of Hf-MOF-808 could be related to the slightly stronger Lewis acidic character of Hf (relative to that of Zr); however, a detailed investigation is essential to support this claim. For this, FTIR analysis using reliable probe molecules would be highly desirable for calculating the exact amount and strength of Lewis acid, and the base sites. In summary, both Zr-MOF-808 and Hf-MOF-808 were found to be active and chemoselective catalysts for the CTH reaction of α, β-unsaturated carbonyl compounds. The selectivity to all allylic alcohols was >97% throughout the reaction. Excellent conversions were always paired with high selectivity. The slightly better performance of Hf-MOF-808 (compared to Zr-MOF-808) could be due to the superior Lewis acidic and basic characteristics of Hf-MOF-808 relative to those of Zr-MOF-808; however, detailed characterizations will be required to confirm this point. This work was supported by Korea Institute of Energy Technology Evaluation and Planning (KETEP) and the Ministry of Trade, Industry and Energy (MOTIE) of the republic of Korea (No. 20202020800330). A.H.V. and Y.K.H. are deeply grateful to the Institutional Research Program of KRICT (SI2111-40) for financial support. The authors would like to thank Dr. U-H. Lee, D.-Y. Hong, and Dr. D. W. Hwang for helpful discussions. Additional supporting information is available in the online version of this article. Appendix S1: Supporting information Please note: The publisher is not responsible for the content or functionality of any supporting information supplied by the authors. Any queries (other than missing content) should be directed to the corresponding author for the article.
更多查看译文
关键词
Metal-organic frameworks, Metal-node modification, Catalytic transfer hydrogenation, Unsaturated carbonyl compounds
AI 理解论文
溯源树
样例
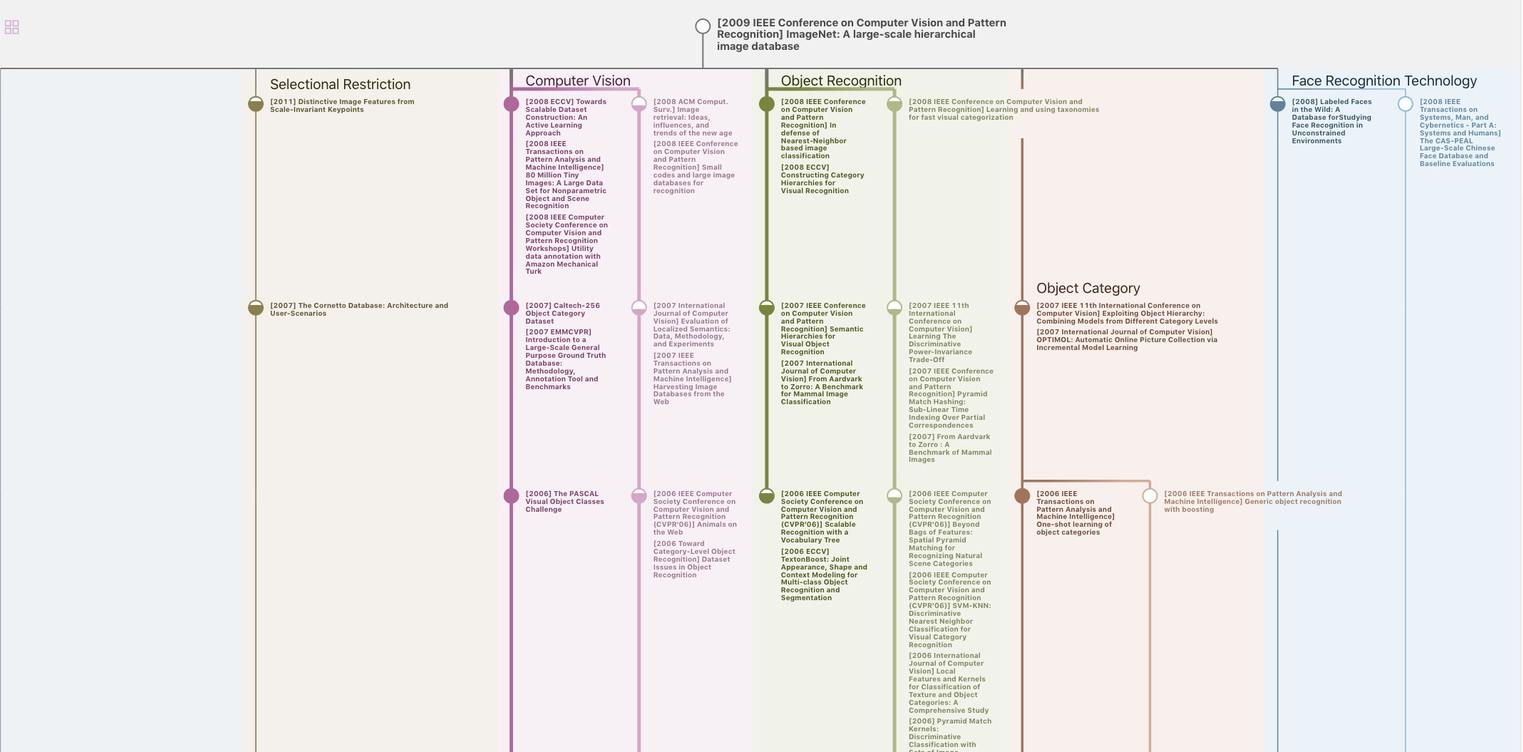
生成溯源树,研究论文发展脉络
Chat Paper
正在生成论文摘要