Cycling Cardiomyocytes Scarce But Important In Recovery From Heart Infarction?
Circulation research(2021)
摘要
HomeCirculation ResearchVol. 128, No. 2Cycling Cardiomyocytes Free AccessEditorialPDF/EPUBAboutView PDFView EPUBSections ToolsAdd to favoritesDownload citationsTrack citationsPermissions ShareShare onFacebookTwitterLinked InMendeleyRedditDiggEmail Jump toFree AccessEditorialPDF/EPUBCycling CardiomyocytesScarce but Important in Recovery From Heart Infarction? Wouter Derks, Olaf Bergmann Wouter DerksWouter Derks https://orcid.org/0000-0002-6508-0078 Center for Regenerative Therapies Dresden, TU Dresden, Dresden, Germany (W.D., O.B.). Search for more papers by this author and Olaf BergmannOlaf Bergmann Correspondence to: Olaf Bergmann, Karolinska Institutet, Cell and Molecular Biology, SE-17177 Stockholm, Sweden. Email E-mail Address: [email protected] https://orcid.org/0000-0003-1065-4107 Center for Regenerative Therapies Dresden, TU Dresden, Dresden, Germany (W.D., O.B.). Karolinska Institutet, Cell and Molecular Biology, Stockholm, Sweden (O.B.). Search for more papers by this author Originally published21 Jan 2021https://doi.org/10.1161/CIRCRESAHA.120.318574Circulation Research. 2021;128:169–171This article is a commentary on the followingLoss of Endogenously Cycling Adult Cardiomyocytes Worsens Myocardial FunctionThe adult mammalian heart has a very limited capacity to replace lost contractile cells with newly formed cardiomyocytes. Although cell cycle activity has been described in cardiomyocytes even in old age, in homeostasis, and in an injured heart,1,2 it is largely unknown to what extent cell cycle activity leads to the formation of new cardiomyocytes and whether these cells have a beneficial effect in heart regeneration. Moreover, it has been notoriously difficult to distinguish between dividing cardiomyocytes and myocytes undergoing endoreplication, which become either polyploid or multinucleated.3 The functional impact of both polyploidy and cardiomyocyte proliferation on homeostasis and heart disease has been heavily discussed but is still unclear.Article, see p 155Previous attempts to label cycling and proliferating cardiomyocytes in different stages of development and disease have included classical strategies using nucleotide analogs,2 genetic labeling strategies to visualize cell cycle- and cytokinesis-related proteins,4–6 fate-mapping strategies based on inducible CreER (Ki67iresCreERT2) mice, transgenic MADM (mosaic analysis with double markers) and clonal analysis (Brainbow/Confetti) to label the progeny of dividing cardiomyocytes.7–9 However, all these reporter systems have their own limitations, such as unknown genomic integration sites, unspecific labeling of noncardiomyocytes, and cardiotoxicity induced by continuous tamoxifen infusion.10In this issue of Circulation Research, Bradley et al11 describe a new transgenic approach using a fate-mapping strategy for tracking cycling cardiomyocytes to identify proliferation and polyploidy events. The authors generated a transgenic mouse using the promoter of the Ki67 gene, which is expressed only in cycling cells and is a widely accepted marker of cell cycle activity.12 After combining both the Dre and Cre recombinase systems (α-DKRC mouse [αMHC-MerDreMer-Ki67p-RoxedCre::Rox-Lox-tdTomato-eGFP, denoted as α-DKRC::RLTG]), a single series of tamoxifen injections activates Dre recombinase, leading to tdTomato expression in all cardiomyocytes, and steady Cre recombinase expression in cycling cardiomyocytes (Ki67+), thereby labeling all cycling cardiomyocytes and their progeny with eGFP (enhanced green fluorescent protein) (Figure).Download figureDownload PowerPointFigure. Description of α-DKRC (αMHC-MerDreMer-Ki67p-RoxedCre::Rox-Lox-tdTomato-eGFP, denoted as α-DKRC::RLTG) mice used to label and ablate cycling cardiomyocytes. The α-DKRC mice created by Bradley et al11 make use of a tamoxifen-inducible, cardiomyocyte-specific Dre recombinase, which in turn activates Cre recombinase under control of the Ki67 promoter. Cre recombinase expression is, therefore, limited to cycling cardiomyocytes, and by combining this system with RLTG (αMHC-MerDreMer-Ki67p-RoxedCre) and DTA mice, it is possible to specifically label or ablate these cells. Labeling strategy: In α-DKRC::RLTG mice, Dre recombinase induces tdTomato labeling of cardiomyocytes, and Cre recombinase induces eGFP (enhanced green fluorescent protein) labeling. The authors induced ischemia/reperfusion (I/R) myocardial infarction (MI) injury after labeling and found increased numbers of cycling cardiomyocytes, mostly located in the border zone of the infarction. Paired eGFP+ cardiomyocytes indicate proliferating cells, while single eGFP+ cardiomyocytes are likely to be endoreplicated cells. Ablation strategy: By combining their α-DKRC mouse model with a Cre-inducible diphtheria toxin ablation system (DTA mice), the authors ablated all cycling cardiomyocytes after a series of tamoxifen injections. Cycling adult cardiomyocytes showed a decrease in cardiac function and an increased scar size after induced myocardial injury.Using this labeling strategy, the authors found increased numbers of eGFP-labeled cardiomyocytes in mice subjected to ischemia/reperfusion myocardial infarction injury, which suggested increased cell cycle activity (Figure). Most of these labeled cardiomyocytes were located in the border zone of the infarction, as has also been shown in previous studies using nucleotide analogs.2 However, this type of analysis quantifies all types of cell cycle activity in cardiomyocytes, including ploidy and multinucleation events. To distinguish cardiomyocyte duplication from endoreplication, the authors reasoned that there must have been a high likelihood (>99%) for eGFP-labeled cardiomyocytes localized next to each other to be a product of cell duplication, whereas single eGFP-positive cardiomyocytes were most likely a result of endoreplication. The vast majority of eGFP-positive cardiomyocytes were found to be single, and only ≈10% were found next to another eGFP-labeled cardiomyocyte. This provides evidence that the cell cycle activity observed in the injured heart only modestly adds to the pool of existing cardiomyocytes, while mostly leading to increased ploidy and multinucleation, in line with previous studies suggesting a ratio of proliferation to endoreplication of 14%2. As the recombination efficiency of α-DKRC mice was not reported, it is difficult to compare the reported numbers of cycling and proliferating cells with those from previous reports on cycling cardiomyocytes. Interestingly, a slightly larger fraction of paired eGFP-labeled cells (proliferating cardiomyocytes) was found in the border zone of the infarction than in the remote zone, indicating that the microenvironment in the border zone increases the likelihood of successful cytokinesis, possibly involving factors that are secreted into the extracellular matrix and can promote successful cytokinesis.13Although a number of studies have documented increased cell cycle activity after cardiac injury, the functional significance of these cells has not been elucidated. Therefore, the authors aimed to kill off all cycling cardiomyocytes to investigate the effect of their loss on functional recovery after cardiac infarction. The authors combined their α-DKRC mouse model with a Cre-inducible diphtheria toxin ablation system, which allows selective ablation of cycling cardiomyocytes from the time of tamoxifen injection (Figure). Mice with ablated cycling cardiomyocytes showed decreased cardiac function, compared with that in the control group, over a period of up to 12 weeks after myocardial injury. As any entry into the cell cycle inevitably leads to induced cell death in recombined cardiomyocytes, the total number of ablated cardiomyocytes increases with time after infarction. A study using [15N]thymidine to identify cycling cardiomyocytes after infarction even found >20% cycling cardiomyocytes in the border zone within 8 weeks after infarction2. Although this proportion might reflect the upper threshold of cycling cells, it is reasonable to assume that the loss of cardiomyocytes, irrespective of their cell cycle stage, would have also compromised heart function. Therefore, it remains unknown whether the partial loss of myocardial function could be attributed to inherent properties of cycling cardiomyocytes or, rather, to a random loss of functional cardiomyocytes throughout the heart.More refined mouse models, able to halt cell cycle progression, such as described with the Cdkn1aSUPER mouse,14 would be necessary to assess to what extent myocardial recovery is attributable to the unique properties of cycling cardiomyocytes. Likewise, blocking cytokinesis in cycling cells, for example, by knocking out Ect-2, as has previously been done in zebrafish,15 could further help understand the contribution of cardiomyocyte proliferation versus merely cell cycle activity to heart recovery. Furthermore, in this study, discrimination between endoreplication and proliferation was only indirectly inferred from the spatial location of eGFP-positive cardiomyocytes, a strategy that could also be applied using traditional labeled nucleotide analogs. Thus, the quest for the ultimate methodology to label the rare population of duplicating cardiomyocytes is still open.Nonetheless, this α-DKRC mouse model can be a useful tool to study cardiomyocyte cycle activity and proliferation and is very valuable for specifically manipulating gene expression in cycling cardiomyocytes to increase the rate of successful cytokinesis and thereby generate new cardiomyocytes. Moreover, this study provided evidence that even a small loss of cardiomyocytes compromised heart function after cardiac infarction. In this light, strategies to augment adult cardiomyocyte proliferation seem to be an attractive avenue for recovering heart function and regenerating the heart.Sources of FundingO. Bergmann was supported by the Center for Regenerative Therapies Dresden, the Karolinska Institutet, the Swedish Research Council, the Ragnar Söderberg Foundation, and the Åke Wiberg Foundation.Disclosures None.FootnotesThe opinions expressed in this article are not necessarily those of the editors or of the American Heart Association.For Sources of Funding and Disclosures, see page 171.Correspondence to: Olaf Bergmann, Karolinska Institutet, Cell and Molecular Biology, SE-17177 Stockholm, Sweden. Email olaf.[email protected]seReferences1. Alkass K, Panula J, Westman M, Wu TD, Guerquin-Kern JL, Bergmann O. No evidence for cardiomyocyte number expansion in preadolescent mice.Cell. 2015; 163:1026–1036. doi: 10.1016/j.cell.2015.10.035CrossrefMedlineGoogle Scholar2. Senyo SE, Steinhauser ML, Pizzimenti CL, Yang VK, Cai L, Wang M, Wu TD, Guerquin-Kern JL, Lechene CP, Lee RT. Mammalian heart renewal by pre-existing cardiomyocytes.Nature. 2013; 493:433–436. doi: 10.1038/nature11682CrossrefMedlineGoogle Scholar3. Leone M, Magadum A, Engel FB. Cardiomyocyte proliferation in cardiac development and regeneration: a guide to methodologies and interpretations.Am J Physiol Heart Circ Physiol. 2015; 309:H1237–H1250. doi: 10.1152/ajpheart.00559.2015CrossrefMedlineGoogle Scholar4. Hesse M, Doengi M, Becker A, Kimura K, Voeltz N, Stein V, Fleischmann BK. Midbody positioning and distance between daughter nuclei enable unequivocal identification of cardiomyocyte cell division in mice.Circ Res. 2018; 123:1039–1052. doi: 10.1161/CIRCRESAHA.118.312792LinkGoogle Scholar5. Raulf A, Horder H, Tarnawski L, Geisen C, Ottersbach A, Röll W, Jovinge S, Fleischmann BK, Hesse M. Transgenic systems for unequivocal identification of cardiac myocyte nuclei and analysis of cardiomyocyte cell cycle status.Basic Res Cardiol. 2015; 110:33. doi: 10.1007/s00395-015-0489-2CrossrefMedlineGoogle Scholar6. Alvarez R, Wang BJ, Quijada PJ, Avitabile D, Ho T, Shaitrit M, Chavarria M, Firouzi F, Ebeid D, Monsanto MM, et al.. Cardiomyocyte cell cycle dynamics and proliferation revealed through cardiac-specific transgenesis of fluorescent ubiquitinated cell cycle indicator (FUCCI).J Mol Cell Cardiol. 2019; 127:154–164. doi: 10.1016/j.yjmcc.2018.12.007CrossrefMedlineGoogle Scholar7. Kretzschmar K, Post Y, Bannier-Hélaouët M, Mattiotti A, Drost J, Basak O, Li VSW, van den Born M, Gunst QD, Versteeg D, et al.. Profiling proliferative cells and their progeny in damaged murine hearts.Proc Natl Acad Sci U S A. 2018; 115:E12245–E12254. doi: 10.1073/pnas.1805829115CrossrefMedlineGoogle Scholar8. Ali SR, Hippenmeyer S, Saadat LV, Luo L, Weissman IL, Ardehali R. Existing cardiomyocytes generate cardiomyocytes at a low rate after birth in mice.Proc Natl Acad Sci U S A. 2014; 111:8850–8855. doi: 10.1073/pnas.1408233111CrossrefMedlineGoogle Scholar9. Sereti KI, Nguyen NB, Kamran P, Zhao P, Ranjbarvaziri S, Park S, Sabri S, Engel JL, Sung K, Kulkarni RP, et al.. Analysis of cardiomyocyte clonal expansion during mouse heart development and injury.Nat Commun. 2018; 9:754. doi: 10.1038/s41467-018-02891-zCrossrefMedlineGoogle Scholar10. Hall ME, Smith G, Hall JE, Stec DE. Systolic dysfunction in cardiac-specific ligand-inducible MerCreMer transgenic mice.Am J Physiol Heart Circ Physiol. 2011; 301:H253–H260. doi: 10.1152/ajpheart.00786.2010CrossrefMedlineGoogle Scholar11. Bradley LA, Young A, Li H, Billcheck HO, Wolf MJ. Loss of endogenously cycling adult cardiomyocytes worsens myocardial function.Circ Res. 2021; 128:155–168. doi: 10.1161/CIRCRESAHA.120.318277LinkGoogle Scholar12. Whitfield ML, George LK, Grant GD, Perou CM. Common markers of proliferation.Nat Rev Cancer. 2006; 6:99–106. doi: 10.1038/nrc1802CrossrefMedlineGoogle Scholar13. Wu CC, Jeratsch S, Graumann J, Stainier DYR. Modulation of mammalian cardiomyocyte cytokinesis by the extracellular matrix.Circ Res. 2020; 127:896–907. doi: 10.1161/CIRCRESAHA.119.316303LinkGoogle Scholar14. Torgovnick A, Heger JM, Liaki V, Isensee J, Schmitt A, Knittel G, Riabinska A, Beleggia F, Laurien L, Leeser U, et al.. The Cdkn1aSUPER mouse as a tool to study p53-mediated tumor suppression.Cell Rep. 2018; 25:1027–1039.e6. doi: 10.1016/j.celrep.2018.09.079CrossrefMedlineGoogle Scholar15. González-Rosa JM, Sharpe M, Field D, Soonpaa MH, Field LJ, Burns CE, Burns CG. Myocardial polyploidization creates a barrier to heart regeneration in zebrafish.Dev Cell. 2018; 44:433–446.e7.CrossrefMedlineGoogle Scholar Previous Back to top Next FiguresReferencesRelatedDetailsRelated articlesLoss of Endogenously Cycling Adult Cardiomyocytes Worsens Myocardial FunctionLeigh A. Bradley, et al. Circulation Research. 2021;128:155-168 January 22, 2021Vol 128, Issue 2Article InformationMetrics Download: 392 © 2020 American Heart Association, Inc.https://doi.org/10.1161/CIRCRESAHA.120.318574PMID: 33476201 Originally publishedJanuary 21, 2021 KeywordsregenerationEditorialscell cyclehomeostasisheart diseasesmyocardial infarctionPDF download
更多查看译文
关键词
Editorials, cell cycle, heart diseases, homeostasis, myocardial infarction, regeneration
AI 理解论文
溯源树
样例
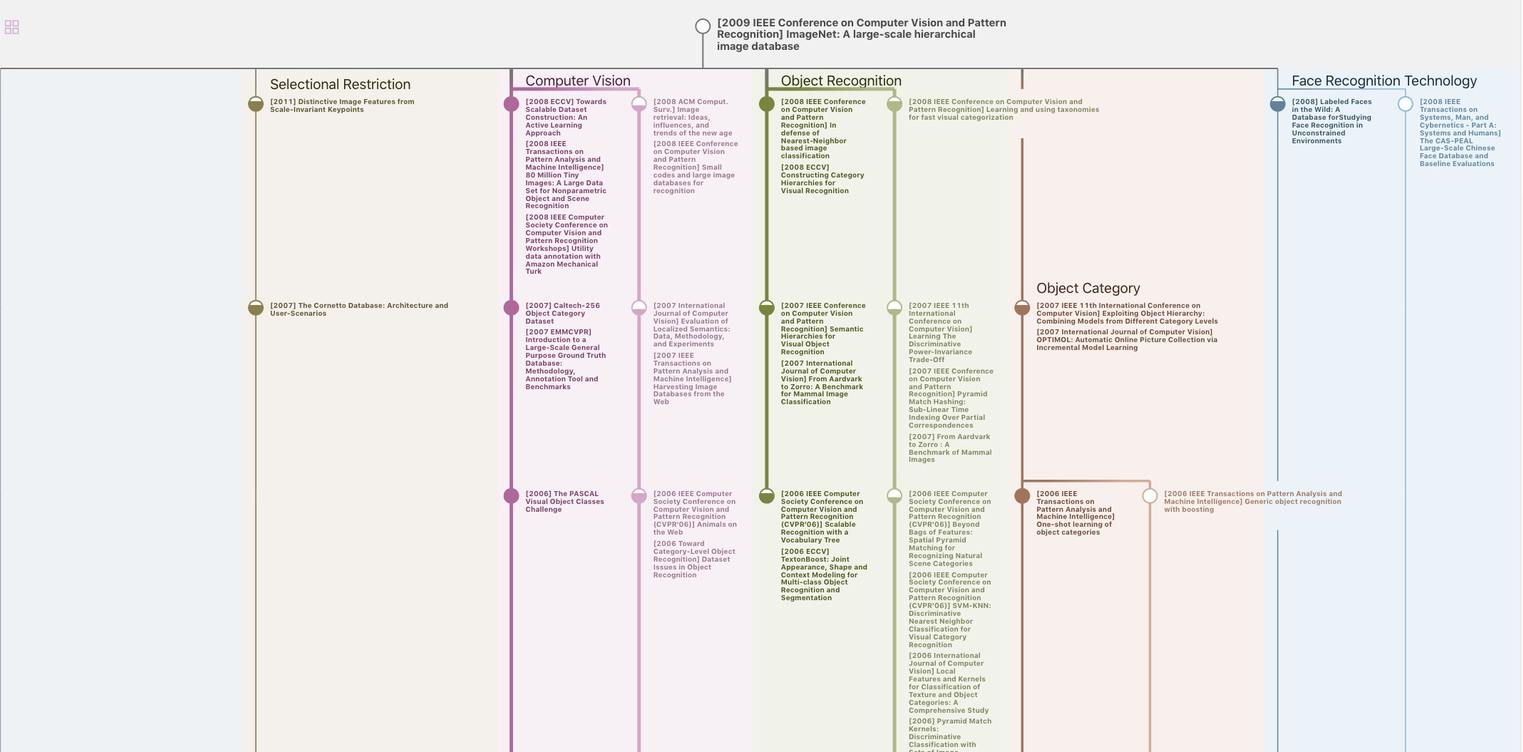
生成溯源树,研究论文发展脉络
Chat Paper
正在生成论文摘要