BASIC INVESTIGATION Vascular Endothelial Growth Factor Accelerates Establishment of a Model of Hepatic Metastasis in Walker-256 Tumor-Bearing Rats
semanticscholar
摘要
Background: Animal models of secondary liver cancer are limited by the time required for the development of hepatic metastases. The authors administered vascular endothelial growth factor (VEGF) to stimulate tumor growth in a model of hepatic metastasis. Methods: A 0.5 to 1.0 mm3 Walker-256 carcinosarcoma tumor tissue was implanted into the livers of 45 Sprague-Dawley rats, randomly assigned to 3 equal groups to receive daily injections (0.1 mL), for 1 week, of either normal saline (control group), 20 mg/L VEGF (VEGF-20 group) or 40 mg/L VEGF (VEGF-40 group). Tumor growth was assessed by magnetic resonance imaging after 3, 7 and 14 days, and overall survival was recorded. Results: Three days after implantation, no tumors were detected by magnetic resonance imaging in the control group. In contrast, tumors were observed in 50% of rats in the VEGF-20 group and 66.7% of rats in the VEGF-40 group (P , 0.05). By day 7, tumors were detected in 92.8% of rats in the VEGF-20 group, 86.7% of rats in the VEGF-40 group, but only 21.4% of rats in the control group (P , 0.05). Tumor size increased progressively, reaching 1.81 6 0.08, 2.51 6 0.12 and 2.67 6 0.10 cm3 in the control, VEGF-20 and VEGF-40 groups, respectively, 14 days after implantation of tumor tissue. Median survival times were significantly shorter in the VEGF40 group (15 days) than in the control and VEGF-20 groups (27 and 25, respectively) (both P , 0.05). Conclusions: Daily VEGF injection (20 mg/L, 1 week) accelerates tumorigenesis without compromising survival, potentially extending the period in which experiments can be conducted in this model. Key Indexing Terms: Vascular endothelial growth factor; Carcinoma 256; Walker; Liver neoplasms; Experimental; Carcinoma; Hepatocellular. [Am J Med Sci 2015;349(3):234–239.] T he liver is considered to be the most frequent site for bloodborne tumor metastases, with metastatic liver tumors approximately 20 times more common than primary hepatic tumors in Western countries. It is generally thought that about 25% to 50% of all primary tumors metastasize to the liver, with 60% to 80% of gastrointestinal tumors developing hepatic metastasis. The primary tumor in half of all cases originates from the gastrointestinal tract, with other common sites including the breast, ovaries, bronchus and kidney. Hepatic metastasis is an important factor underlying the failure of treatment for gastrointestinal cancer; indeed, patients with uncontrolled hepatic metastasis have a median survival time of only 6 to 30 months. Thus, there is a genuine need for novel treatment strategies, and the mechanisms of hepatic metastasis have been investigated with a view to develop effective measures for the prevention and treatment of secondary liver cancer. Animal models of metastatic liver cancer have played an important role in improving our understanding of how secondary liver cancer develops and progresses and facilitated assessment of novel therapies. An ideal animal model for studying liver metastases would mimic all characteristics of metastasis development in human patients, be practical to use and predictable in its performance, and take into account ethical considerations. Unfortunately, an ideal preclinical model has yet to be developed. Nonetheless, various approaches have been used and have proven useful in expanding our understanding of tumorigenesis. Many studies have implanted human colorectal, gastric or pancreatic cancer cells into the livers of severe combined immune deficiency (SCID) mice or implanted colorectal cancer cells into the liver of rats, allowing the growth and biochemistry of orthotopic tumors to be studied in vivo. Rat breast cancer–derived Walker-256 cells are commonly used for establishing rat models of hepatic metastases, as these cells may be easily obtained and highly controllable. A major limitation of this method is that about 1 month is normally required for the establishment of hepatic tumors, 18 and over the past decade, no significant progress has been made to accelerate tumorigenesis in this model. We sought to define a method, which accelerates tumorigenesis, to extend the time period in which experiments can be carried out. Vascular endothelial growth factor (VEGF) specifically interacts with vascular endothelial cells to induce blood vessel formation and is thought to play an important role in the regulation of angiogenesis. However, during tumorigenesis, VEGF can promote tumor cell proliferation. Hence, the role of VEGF in tumor progression, recurrence and metastasis has attracted increasing attention in recent years, with numerous studies investigating the potential of anti-VEGF therapies in animal models of cancer. VEGF has previously been administered in animal models of tumorigenesis, enhancing tumor angiogenesis and accelerating growth. VEGF overexpression has been demonstrated to stimulate proliferation of human melanoma intracerebral xenografts and to stimulate angiogenesis and cyst formation in human ovarian cancer xenografts. We evaluated the feasibility of injection of VEGF in Sprague-Dawley (SD) rats to accelerate the formation of Walker-256 cell tumors in the liver. We found that the injection of VEGF substantially reduced the time required for development of hepatic metastasis. Thus, the approach described in our study provides an improved animal model for the study of mechanisms underlying hepatic metastasis and the efficacy of anti-VEGF therapy for the prevention and treatment of secondary liver cancer. MATERIALS AND METHODS Animals Two-week-old male Wistar rats (body weight, 60–80 g; n 5 2) were used for intraperitoneal passaging; 3-week-old male From the Department of Interventional Radiology, The First Affiliated Hospital, Sun Yat-sen University, Guangzhou, China. Submitted May 18, 2014; accepted in revised form August 8, 2014. This work has received honoraria from the Science and Technology Planning Project of Guangdong Province, China (2012B031800076). The authors have no financial or other conflicts of interest to disclose. Correspondence: Yonghui Huang, Department of Interventional Radiology, The First Affiliated Hospital, Sun Yat-sen University, 58 Zhongshan Road II , Guangzhou, 510080, China (E-mail: 13710397492@163.com). 234 The American Journal of the Medical Sciences Volume 349, Number 3, March 2015 Wistar rats (body weight, 80–100 g; n 5 2) were used for injection of malignant ascites at a site on the inner thigh; and 6to 8-week-old male SD rats (body weight, 200–250 g; n5 45) were used for intrahepatic tumor inoculation (the orthotopic xenograft model). All rats were maintained under specific pathogen-free conditions and were obtained from the Experimental Animal Center, Sun Yat-sen University, Guangzhou, Guangdong, China. This study was approved by the Animal Ethics Committee of Sun Yat-sen University. Cell Culture The rat Walker-256 carcinosarcoma cell line was obtained from the Cell Bank of the Experimental Animal Center of Sun Yat-sen University. Harvesting of Walker-256 Tumor Cells Walker-256 tumor cells were harvested as described previously. A 1-mL volume of Walker-256 cells, corresponding to approximately 1 3 107 cells, was injected intraperitoneally into 2-week-old male Wistar rats (n 5 2). At 7 to 14 days after carcinosarcoma cell inoculation, when the rats showed abdominal distension and puncture-revealed bloody ascites fluid, 5 mL of malignant ascites was extracted and centrifuged for 5 minutes at a speed of 1,200 rpm. The pellet of tumor cells was resuspended, and a 1-mL volume of the cell suspension (corresponding to 2 3 107 cells) was inoculated into 3-weekold male Wistar rats (n 5 2), at a site on the inner thigh. Seven to 10 days after tumor cell injection, subcutaneous tumors of approximately 1 cm in diameter could be seen at the injection site. Establishment of a Model of Breast Carcinoma Metastasis in the Liver An orthotopic liver cancer model was established by methods described previously. Tumor tissue (0.5–1.0 mm3) was collected from the subcutaneous layer of the thigh of each Wistar rat inoculated with Walker-256 cells. Harvested tumor tissue was implanted in the liver of 45 SD rats. The liver of each SD rat was exposed, and a liver lobe pierced carefully with ophthalmic scissors. Tumor tissue was then inserted into the lobe at a depth of one third of the lobe thickness, using ophthalmic tweezers. Subsequently, a 5 3 5 mm piece of Gelfoam (Jinling Pharmaceutical, Nanjing, China) was used to cover the wound, the liver lobe was returned, and the abdomen was closed after the cessation of bleeding. All rats were subsequently returned to their cages to recover from surgery and returned to normal activity. The rats were divided into 3 groups (n 5 15 each): control, VEGF-20 and VEGF-40. Recombinant rat VEGF165 (20 mg per tube; PeproTech, Rocky Hill, NJ) was centrifuged for 30 seconds at 1,000 rpm, dissolved in 100 mL of deionized water and diluted with normal saline solution to the desired concentration. Rats in the control, VEGF-20 and VEGF-40 groups received a daily injection (0.1 mL) of normal saline, 20 mg/L VEGF and 40 mg/L VEGF, respectively, for 1 week. Imaging and Pathological Investigations Magnetic resonance imaging (MRI) and pathological investigations were used to evaluate the development of TABLE 1. Success rate of modeling in each experimental group Group Day 3 Day 7 Day 14 Control 0/14 (0.0) 3/14 (21.4) 12/14 (85.7) VEGF-20 7/14 (50.0)* 13/14 (92.8)** 13/14 (92.8) VEGF-40 10/15 (66.7)* 13/15 (86.7)* 10/12 (83.3) Data are expressed as n (%), relative to the total number of surviving rats in the experimental group at that time point. One rat in each of the control and VEGF-20 groups died 1 day after establishment of the orthotopic cancer model; 3 rats in the VEGF-40 group died between 7 and 14 days. *P , 0.05, **P , 0.01 vs. the control group (x test). VEGF, vascular endothelial growth factor. TABLE 2. Changes in tumor volume measured by MR
更多查看译文
AI 理解论文
溯源树
样例
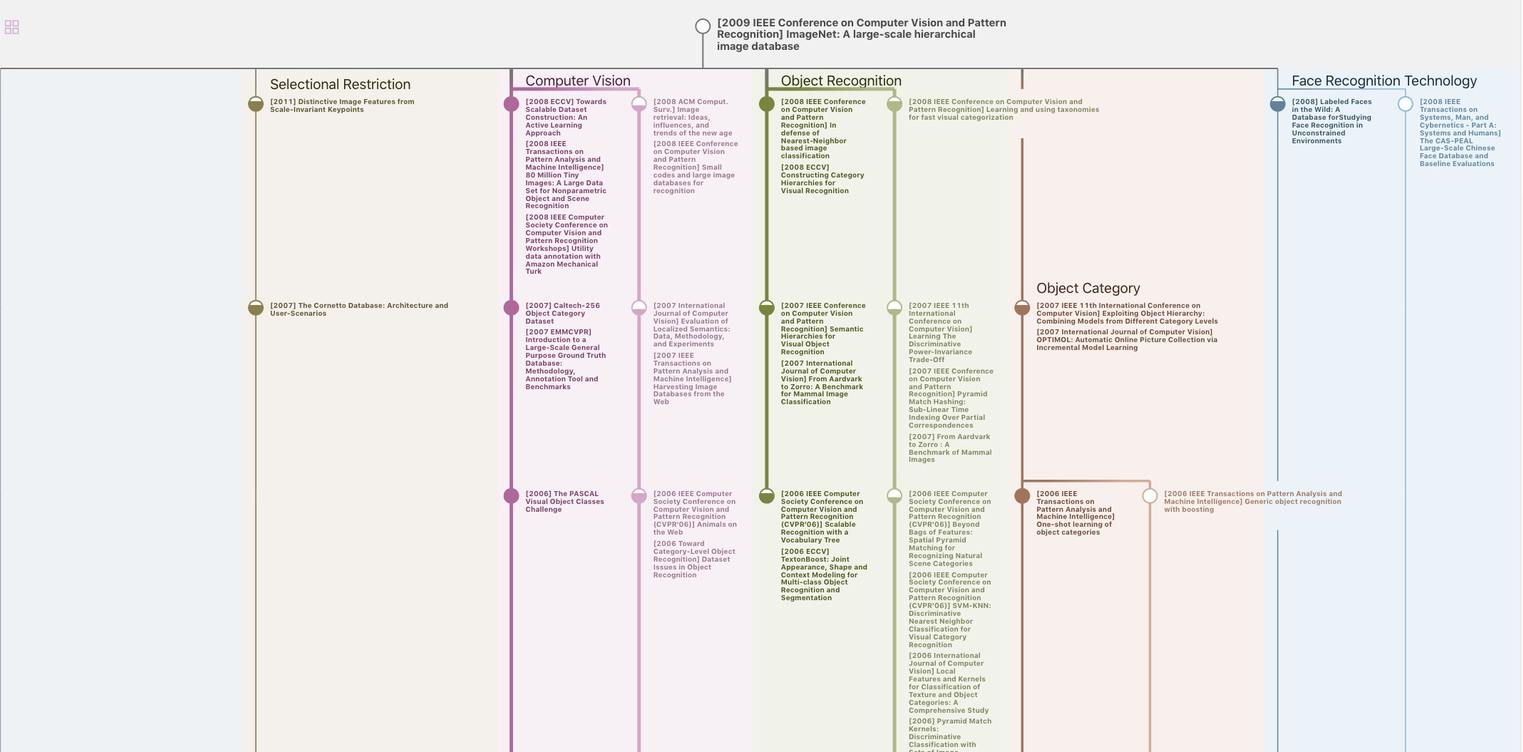
生成溯源树,研究论文发展脉络
Chat Paper
正在生成论文摘要