2019- Abstract book NSABS24_CC003
semanticscholar(2019)
摘要
Microfluidic devices offer a well-controlled environment for crystal nucleation and growth. Important parameters are mixing and the associated shear. Incomplete mixing results in a wide crystal size distribution and affect the morphology of the product. Bulk acoustic waves offer the possibility to quickly mix two liquid streams and to manipulate particles in the microfluidic channel. In this contribution, fast mixing of two liquid streams by acoustic streaming is demonstrated. Miconazole nitrate crystals were grown in the presence of the acoustic field, and focused on the pressure node. Growth and focusing of amyloid fibrils in the microfluidic channel has also been demonstrated. INTRODUCTION Mounting evidence suggests that shear flows influence the aggregation behavior of proteins [1]. Shear influences both the nucleation and crystal growth [2,3]. Acoustic streaming is a great methodology to create lateral convection in otherwise laminar flow operated channels. It offers a good strategy to reduce mass transfer resistance dramatically [4]. It could be expected that the shear patterns associated with the vortex flows of acoustic streaming will affect the crystallization/nucleation process greatly. Small particles or crystals are only affected by the Stokes drag forces and will flow along with the vortices. Larger crystals on the other hand will experience a radiation force and will rapidly focus at either the node or anti-node of the standing pressure wave in the channel [5]. In the present contribution a proof of concept has been obtained for the crystal formation and growth in presence of the acoustic field. Fast nucleation of a small pharmaceutical molecule (miconazole nitrate) was performed in the presence of acoustic streaming. The formed crystal nuclei were allowed to grow under the same conditions. Once a desired size was reached, the crystals were focused on the pressure node and separated from the main flow. Within a broader study on the influence of controlled flow on protein aggregation, we were able to visualize and focus clusters of amyloid fibrils from lysozyme. This is of great relevance since the formation of amyloid fibrils underlies a wide range of human disorders, including neurodegenerative and cardiovascular diseases like Alzheimer and cardiac amyloidosis. EXPERIMENTAL Rectangular microfluidic channels were etched in a silicon wafer. A width and thickness of respectively 375 μm and 310 μm were fabricated. Two inlets and outlets were provided and connected to syringe pumps by means of capillaries. A standing pressure wave was generated inside the channel with a piezo-ceramic element (PZT) having a resonance frequency at 2.0 MHz (Figure 1). Coupling of Comm. Appl. Biol. Sci, Ghent University, 84/1, 2019 75 the microfluidic chip to the PZT was done by a thin glycerol layer. An in-house built PMMA holder ensured a good connection between the chip, PZT and Nanoports. The applied voltage on the PZT was generated by a frequency generator and amplified by a RF power amplifier. Visualization of all the experiments was done using an inverted fluorescent microscope in combination with a CCD camera. First mixing experiments were performed to quickly mix the solvent with the antisolvent. Water containing a fluorescent salt and non-fluorescent DI water were brought into contact in the microfluidic chip. Mixing was initiated by actuating the PZT at a frequency of 1.93 MHz. The recorded movies were analyzed with ImageJ software. The intensity of each pixel was related to the fluorescence intensity and eventually to the efficiency of the mixing (η). For the crystallization experiments miconazole nitrate was dissolved in dimethylsulfoxide (DMSO) (5 w/w%) and brought in the microfluidic channel at a flowrate of 2 μl/min. Water was used as an antisolvent and pumped. Figure 1: Schematic representation of microfluidic channel: The microfluidic channel is connected to a piezo ceramic element vibrating at 1.93 MHz. Actuation is done with a frequency generator. The vibrations are transferred to the channel wall, creating a standing pressure wave (thicknesses are not to scale). The generated vortex flow from the acoustic streaming is shown in the enlarged picture. Through the channel at a flowrate of 8 μl/min. Crystal formation was monitored in absence and presence of the acoustic field. Crystals reaching a cut-off size were focused in the center of the microfluidic channel. In the broader context of protein aggregates, amyloid fibrils from lysozymes were grown overnight in the microfluidic channel and without the presence of an acoustic field. Once the fibrils were formed, a flowrate of 4 μl/min was set and the acoustic field was activated. RESULTS AND DISCUSSION Mixing of two water streams due to the presence of an acoustic field was visualized by a camera. Figure 2a shows snapshots at different times at a fixed observation position (16 mm downstream the contact area) during the mixing process, overlaid with the concentration profile. In this case the flow rate was set to 5 mm/s and the actuation voltage to 15 Vp-p. At t = 0.60 s the channel already had a mixing efficiency of η = 0.94. The mixing efficiency has been measured for different applied voltages and flowrates (Fig. 2b). Actuation at voltages lower than 10 Vp-p does not induce any visible mixing. For a linear velocity of 5 mm/s a mixing of already η = 0.65 is reached at 10 Vp-p actuation. Complete mixing (η = 0.94) is reached at an actuation voltage of 15 Vp-p for both flowrates of 5 mm/s and 10 mm/s. To our knowledge such fast mixing in a microfluidic channel with bulk acoustic waves has not been demonstrated yet.
更多查看译文
AI 理解论文
溯源树
样例
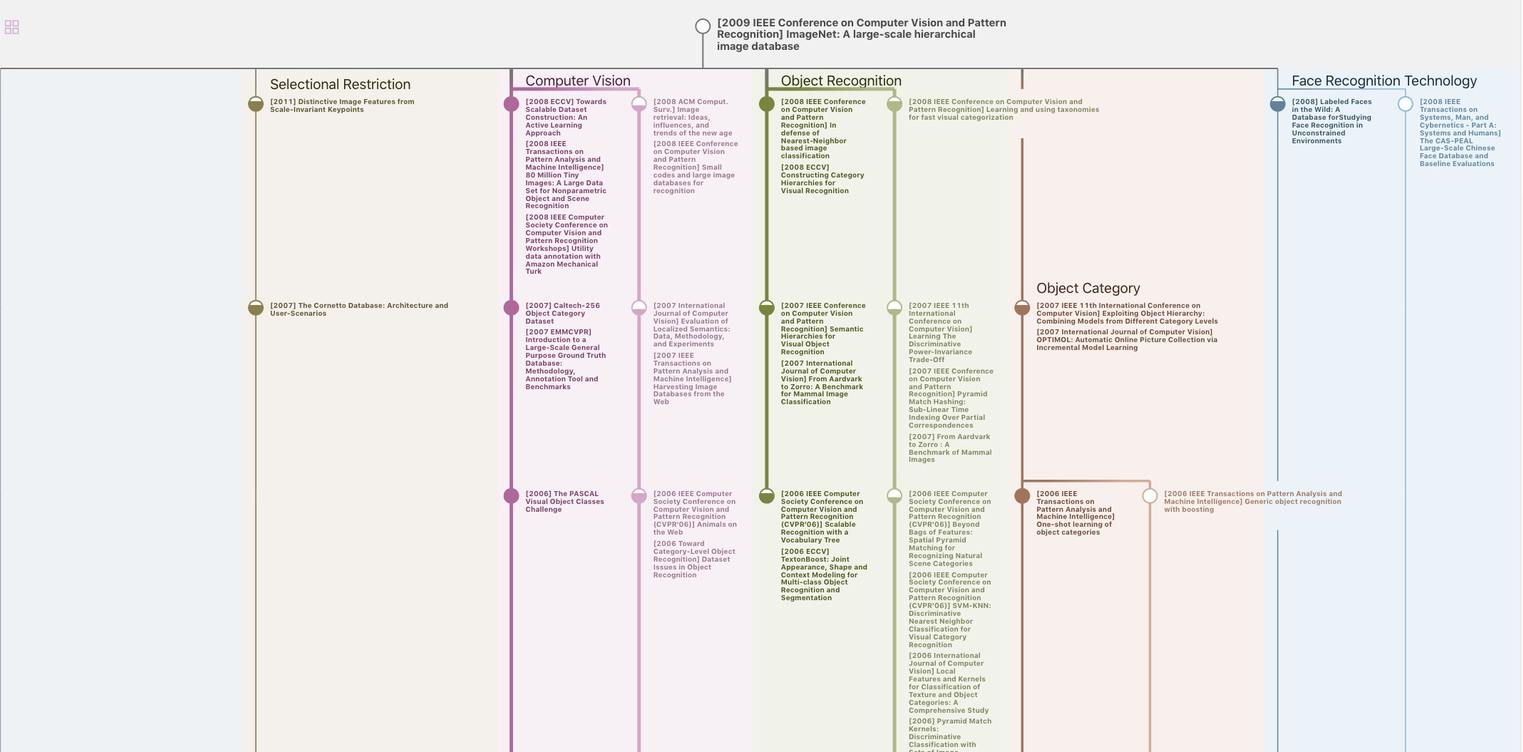
生成溯源树,研究论文发展脉络
Chat Paper
正在生成论文摘要