Room Temperature CW ! " # $ % & $ Single Mode Lasing of InAs Quantum Dot Micro-disk Lasers Grown on ( 001 ) Si
semanticscholar(2017)
摘要
Heteroepitaxially grown InAs quantum dot micro-disk lasers were demonstrated on planar Si (001) substrates. Room-temperature continuous-wave lasing !"#$%'(#with a threshold pump power of )*+#',#was achieved for a 4 μm disk. OCIS codes: (140.3410) Laser resonators; (140.3948) Microcavity devices Integration of direct band-gap III–V materials with the state-of-art complementary metal oxide-semiconductor (CMOS) technology is promising to relieve data exchange bottlenecks encountered both at intra-chip and inter-chip scales [1]. Heteroepitaxial growth of III-V on Si offers a monolithic solution with scalability up to large wafers, but is complicated by crystal defects originated from material incompatibility [2]. Exploiting the capability of quantum dots (QDs) to withstand non-radiative defects [3], we demonstrate room-temperature lasing in InAs QD micro-disk lasers on planar (001) Si substrates at a wavelength of $%'(% The pump power threshold was )*+#',#-./#!#0#'(# disk under continuous-wave optical pumping. Temperature dependence of the lasing characteristics including threshold and lasing wavelength are analyzed. Compared with recent reports of Si based quantum dot lasers [4, 5], this simple direct heteroepitaxy scheme with no absorptive germanium buffer or offcut substrates provides improved compatibility with the mainstream CMOS manufacturing processes and silicon on insulator (SOI) technologies. A 1-μm GaAs-on-silicon template was prepared using the two-step growth method by MOVPE on a (001) Si substrate [6]. The active region contains five layers of self-assembled InAs QDs grown by molecular beam epitaxy (MBE) [7]. A schematic of the whole structure is shown in Fig. 1(a). Micro-disk lasers (MDLs) were fabricated in the 500 nm thick active region supported by the 600-nm Al0.7Ga0.3As post. Fig. 1 (a) Schematic illustration of the epitaxial structure. (b) SEM image of the fabricated device in a 70° tilted view. (c) Room temperature emission spectra under increasing pump intensities. (d) Room temperature emission spectrum at 247.5 1,%#234#symbols represent measured data. The blue line is the fitting sum of using bi-Lorentzian curves. The green line is a fit to cavity emission. (e) Room temperature integrated photoluminescence intensity of the lasing mode as a function of pump power. The dashed line is a guide for eyes. Colloidal lithography and inductively coupled plasma (ICP) etching were performed to define the circular 0#'(# disk mesa. This was followed by partially wet etching underneath to leave a disk supported by a small post at the center. Fig. 1(b) presents a 70° tilted view of a scanning electron micrograph (SEM) of the optically isolated, undercut MDL. The clean surface and smooth sidewall promise excellent optical efficiency in the regions 978-1-943580-11-8/16/$31.00 ©2016 Optical Society of America SM1G.3.pdf CLEO:2016 © OSA 2016 overlapping with the whispering gallery modes. The devices were optically pumped at normal incidence with a 532 nm diode laser under continuous-wave pumping. The laser beam was focused to around 4 '(# 56#75!(4"4/% Light output was analyzed by a monochrometer with InGaAs charge-coupled device array. The device temperature was controlled from 10 K to room temperature. Room temperature emission spectra of a 4 μm diameter disk under progressively higher pump power are shown in Fig. 1(c). 849.:#$;*#',<#=>.6"!64.?=#emission from the QDs was measured with a broad background modulated with small peaks. The peak centered at 1307 nm increases sharply in intensity when the pump power increased to beyond 247.5 ',<#signifying the onset of lasing. By fitting this emission peak to a Lorentzian function shown in Fig. 1(d), the linewidth was extracted to be 0.6 nm, and cold cavity quality factor Q was calculated to be 2178. Fig. 1(e) presents the spectrally integrated emission intensity as a function of the pump intensity, the lasing threshold of @)*+#',#:!=#74"4/(5647#"3/.?A3#!#9564!/#-5"#".#"34#35A34/-power region of the data. The device was then mounted in a Helium gas flow cryostat to study the temperature evolution behavior. The spectra in Fig. 2 (a) show the normalized emission taken at 2.6 times the threshold from room temperature to 10 K. At 10 K, simultaneous lasing on two well-separated modes was observed. The mode at longer wavelength (1208 nm) vanishes when temperature increases above 200 K due to mode competition, bringing in single mode operation. The output intensity vs. input pump power curve (L–L curve) for the dominant line at the three temperatures exhibit pronounced threshold, shown in Fig. 2(b). The threshold power together with central wavelength as a function of temperature are plotted in Fig. 2(c). Temperature variation of the lasing line is governed by both the temperature dependence of a cavity effective refractive index, and temperature shrinkage of an active region bandgap. Blue shift at a rate of 0.4-0.5 nm/K was observed for the lasing mode with the decrease of temperature. Threshold decreases accordingly due to enhanced carrier confinement in the QDs and restrained non-radiative recombination at lower temperatures. Characteristic temperature is extracted to be ~560 K in the temperature range of 10 K to 300 K. Fig. 1 (a) Lasing spectra at 2.6 times the threshold from room temperature to 10 K. (b) L–L curve for the dominant line in the three temperatures. (c) Temperature dependence of threshold and central wavelength In conclusion, through a simple direct heteroepitaxial scheme, we demonstrate room temperature lasing of InAs QDs micro-disks with high luminescence efficiency at $%'(% The results suggest great opportunities for realizing a monolithic platform in III-V/Si integration compatible with conventional CMOS and Si photonics on SOI.
更多查看译文
AI 理解论文
溯源树
样例
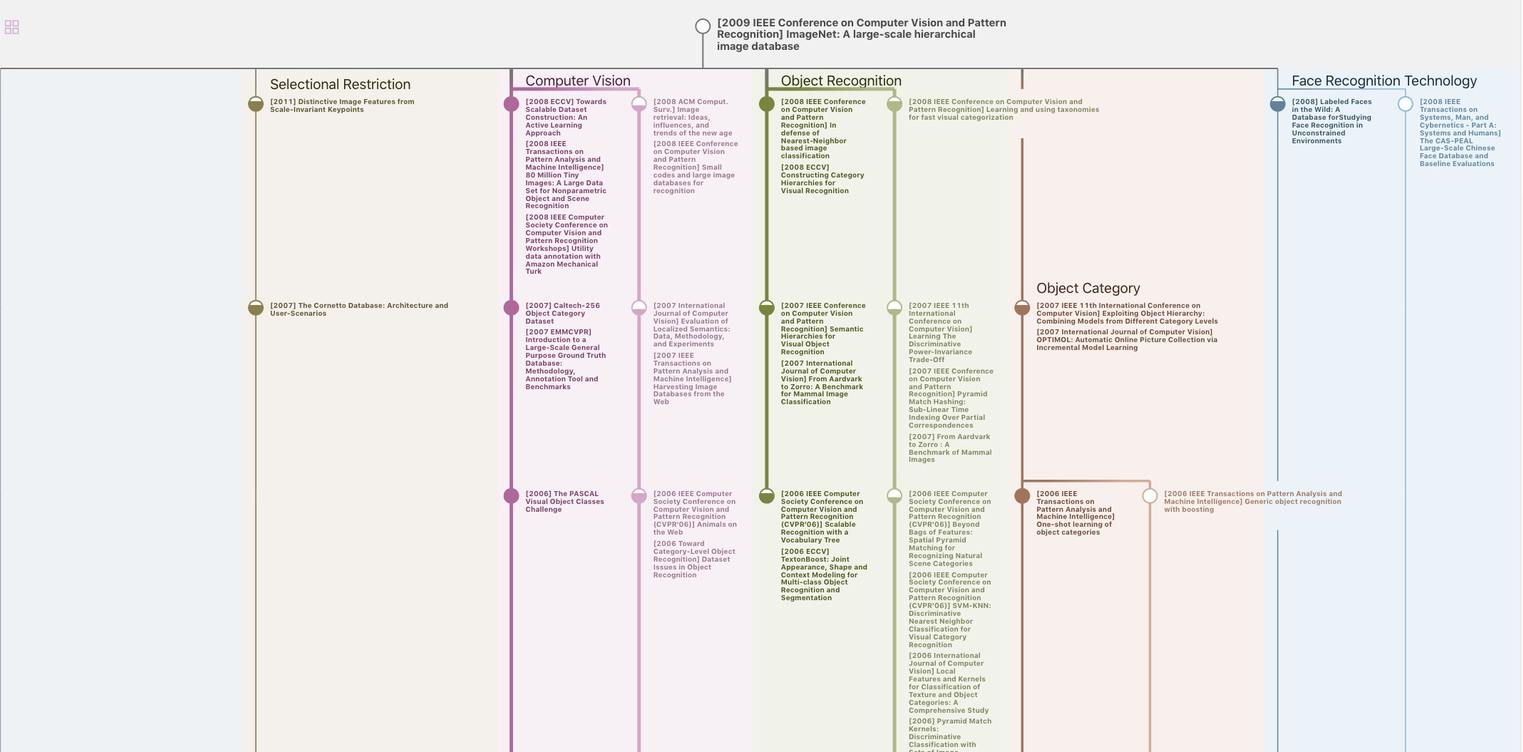
生成溯源树,研究论文发展脉络
Chat Paper
正在生成论文摘要