Mechanistic and computational studies of oxidatively-induced aryl-CF3 bond formation at palladium
semanticscholar(2020)
摘要
This article describes the rational design of 1st generation systems for oxidatively-induced Aryl– CF3 bond-forming reductive elimination from PdII. Treatment of (dtbpy)Pd(Aryl)(CF3) (dtbpy = di-tert-butylbipyridine) with NFTPT (N-fluoro-1,3,5-trimethylpyridium triflate) afforded the isolable PdIV intermediate (dtbpy)Pd(Aryl)(CF3)(F)(OTf). Thermolysis of this complex at 80 °C resulted in Aryl–CF3 bond-formation. Detailed experimental and computational mechanistic studies have been conducted to gain insights into the key reductive elimination step. Reductive elimination from this PdIV species proceeds via pre-equilibrium dissociation of TfO− followed by Aryl–CF3 coupling. DFT calculations reveal that the transition state for Aryl–CF3 bond formation involves the CF3 acting as an electrophile with the Aryl ligand acting as a nucleophilic coupling partner. These mechanistic considerations along with DFT calculations have facilitated the design of a 2nd generation system utilizing the tmeda (N,N,N’,N’-tetramethylethylenediamine) ligand in place of dtbpy. The tmeda complexes undergo oxidative trifluoromethylation at room temperature. Introduction The formation of C–CF3 bonds is an important transformation for the construction of pharmaceuticals and agrochemicals.1 Replacing a methyl group with a trifluoromethyl substituent can have a profound effect on the physical and biological properties of a molecule.2 As a result, there is high demand for versatile synthetic methods for generating carbon–CF3 bonds.3 While there has been significant progress in the construction of sp3carbon–CF3 linkages,3 there are comparatively fewer methods for Aryl–CF3 bondformation.4,5,6,7 Transition metal-catalyzed cross-coupling between Aryl–X and CF3–Y would serve as an attractive method for the synthesis of benzotrifluorides.8,9,10,11 The use of Pd-based catalysts for such transformations is of particular interest because they serve as versatile and widely used catalysts for a variety of other carbon–carbon bond-forming reactions.12 However, developments in this area have been limited by the challenges associated with a key step of the cross-coupling catalytic cycle, namely Aryl–CF3 bond-forming reductive elimination from PdII centers.4,13 The vast majority of known Pd(Aryl)(CF3) complexes are inert to Aryl–CF3 coupling at temperatures as high as 150 °C.24,21 * mssanfor@umich.edu . Supporting Information Avaliable. Experimental and computational details and spectroscopic data for new compounds. This material is available free of charge via the internet at http://pubs.acs.org. NIH Public Access Author Manuscript J Am Chem Soc. Author manuscript; available in PMC 2012 May 18. Published in final edited form as: J Am Chem Soc. 2011 May 18; 133(19): 7577–7584. doi:10.1021/ja201726q. N IH PA Athor M anscript N IH PA Athor M anscript N IH PA Athor M anscript Two strategies have been utilized in the literature to address this challenge. The first has focused on achieving Aryl–CF3 bond-forming reductive elimination from PdII via steric and electronic modification of the ancillary ligands (L) at (L)nPd(Aryl)(CF3). For example, pioneering work by Grushin demonstrated that the Xantphos ligand (Xantphos = 4,5bis(diphenylphosphino)-9,9-dimethylxanthene) facilitates high yielding formation of trifluorotoluene from (Xantphos)Pd(Ph)(CF3) at 80 °C (eq. 1).14 More recently, Buchwald has elegantly demonstrated that the sterically large monodentate phosphine ligand Brettphos (Brettphos = 2-(dicyclohexylphosphino)-3,6-dimethoxy-2′,4′,6′-triisopropyl-1,1′-biphenyl) promotes stoichiometric Aryl–CF3 coupling from (Brettphos)Pd(Aryl)(CF3) at 80 °C (eq. 2).15 Remarkably, this latter system was successfully applied to the catalytic trifluoromethylation of aryl chlorides with Et3SiCF3 at 130–140 °C.15 While this first approach has provided important breakthroughs, PdII-mediated Aryl–CF3 bond-forming reactions remain limited by the requirement for specialized and expensive phosphine ligands16,17 relatively high reaction temperatures (80-140 °C), and the need for expensive Et3SiCF3 in catalytic processes. As a result, several groups have focused on a second, complementary strategy for achieving Aryl–CF3 bond-forming reductive elimination from Pd.19,20,21 This approach hinges on changing the oxidation state, rather than the ancillary ligand environment, at the metal center. As shown in eq. 3, it was expected that palladium(IV) complexes of general structure (L)2(X)2Pd(Aryl)(CF3) (A) would be highly kinetically and thermodynamically reactive towards Aryl–CF3 coupling. This hypothesis is predicated on literature reports showing that PdIV complexes participate in numerous carbon-heteroatom bond-forming reductive elimination reactions that remain challenging at PdII centers.22,23 A key advantage of this approach would be that PdIV intermediate A could potentially be accessed using nucleophilic (CF3), electrophilic (CF3), or free-radical (CF3·) based trifluoromethylating reagents. Two preliminary examples have shown the viability of this approach. First, Yu and coworkers have elegantly demonstrated the PdII/IV-catalyzed ligand-directed C–H trifluoromethylation with electrophilic trifluoromethylating reagents (CF3 reagents).19 Subsequent stoichiometric studies implicated a mechanism involving: (a) C–H activation to form a cyclometalated PdII–Aryl intermediate, (b) oxidation with CF3 to generate Ball et al. Page 2 J Am Chem Soc. Author manuscript; available in PMC 2012 May 18. N IH PA Athor M anscript N IH PA Athor M anscript N IH PA Athor M anscript Pd(Aryl)(CF3), and (c) Aryl–CF3 bond-forming reductive elimination from PdIV to release the product.20 In a second example, our group has shown the viability of stoichiometric Aryl–CF3 bondforming reductive elimination from PdIV centers bearing σ-aryl ligands that do not contain chelate directing groups.21 In this system, the key PdIV intermediate is generated via oxidation of a pre-assembled Pd(Aryl)(CF3) species with an N-fluoropyridinium reagent. We report herein a detailed mechanistic study of Aryl–CF3 bond-forming reductive elimination from PdIV in this latter system. This work provides valuable insights into the influence of ancillary ligands, the role of each coupling partner, and the nature of the transition state for this transformation. These mechanistic studies have also allowed us to rationally design the first examples of room temperature Aryl–CF3 bond-formation from palladium. Results and Discussion Development and Scope of Aryl–CF3 Coupling at PdIV. Our studies began with the synthesis of a series of Pd–CF3 complexes of general structure (dtbpy)Pd(Aryl)(CF3) (1a-i, dtbpy = 4,4′-di-tert-butyl-2,2′-bipyridine). These compounds were prepared by the treatment of (dtbpy)PdII(Aryl)(I) with CsF followed by TMSCF3 in THF at 23 °C (Table 1). The products were isolated as yellow solids in 32-70% yield.21 Xray quality crystals of 1a were obtained by vapor diffusion of pentanes into a dichloromethane solution of 1a, and the X-ray crystal structure of this complex is shown in Figure 1. The PdII complexes (1a-i) are inert towards direct Aryl–CF3 bond-forming reductive elimination. For example, heating 1a at 130 °C for 72 h produced <5% of 4fluorobenzotrifluoride (2a), and the PdII starting material could be recovered in >80% yield (eq. 4). Importantly, similarly low reactivity has been reported in the literature for other (L~L)Pd(Aryl)(CF3) complexes (L~L = diphenylphosphinoethane, diphenylphosphinopropane, and diphenylphosphinobenzene).24a,b As discussed above, the only demonstrated examples of Aryl–CF3 bond-forming reductive elimination from PdII require relatively high temperatures (80 °C) and specialized phosphine ligands.14,15a We reasoned that the 2e− oxidation of (dtbpy)Pd(Aryl)(CF3) would yield a highly reactive PdIV adduct that might undergo more facile Aryl–CF3 bond-forming reductive elimination (eq. 5, i). Thus, we examined the reaction of 1a with N-bromosuccinimide (NBS), Nchlorosuccinimide (NCS), and PhI(OAc)2, which are all well-known to promote the oxidation of PdII to PdIV.22 As shown in Table 2, all three oxidants reacted rapidly with 1a in nitrobenzene-d5 at 80 °C. However, the desired trifluoromethylated product was not obtained; instead, the major organic product contained a nucleophile derived from the oxidant (Br, Cl, or OAc, respectively). These results are consistent with the formation of a PdIV intermediate, from which Aryl–X (X = OAc, Br, and Cl) bond-forming reductive elimination is significantly faster than Aryl–CF3 coupling (eq. 5, ii).21 Ball et al. Page 3 J Am Chem Soc. Author manuscript; available in PMC 2012 May 18. N IH PA Athor M anscript N IH PA Athor M anscript N IH PA Athor M anscript In an effort to avoid competing reductive elimination processes, we next examined the use of electrophilic fluorinating reagents (F+ sources). These reagents were selected based on the hypothesis that fluoride (the X-type ligand introduced to PdIV by F+ sources) might undergo slower reductive elimination than CF3 .22d,27,28 Gratifyingly, a variety of different F+ reagents reacted with 1a to afford modest to excellent yields of the trifluoromethylated product 2a after 3 h at 80 °C (Table 2, entries 4-10). The optimal electrophillic fluorinating reagent was N-fluoro-1,3,5-trimethylpyridium triflate (NFTPT), which provided 2a in 70% yield as determined by 19F NMR spectroscopy.29 Importantly, <5% of products derived from Aryl–F or Aryl–OTf coupling were observed under these conditions. This transformation was next applied to complexes 1b-i, which contain sterically and electronically diverse aryl groups. As shown in Table 3, the yield of trifluoromethylated product was relatively insensitive to the electronic properties of the arene, and the reaction proceeded with good results in systems containing both electron withdrawing [e.g., CN, C(O)Ph] and electron donating (e.g., CH3, OCH3) paraand meta-substituents.30 At
更多查看译文
AI 理解论文
溯源树
样例
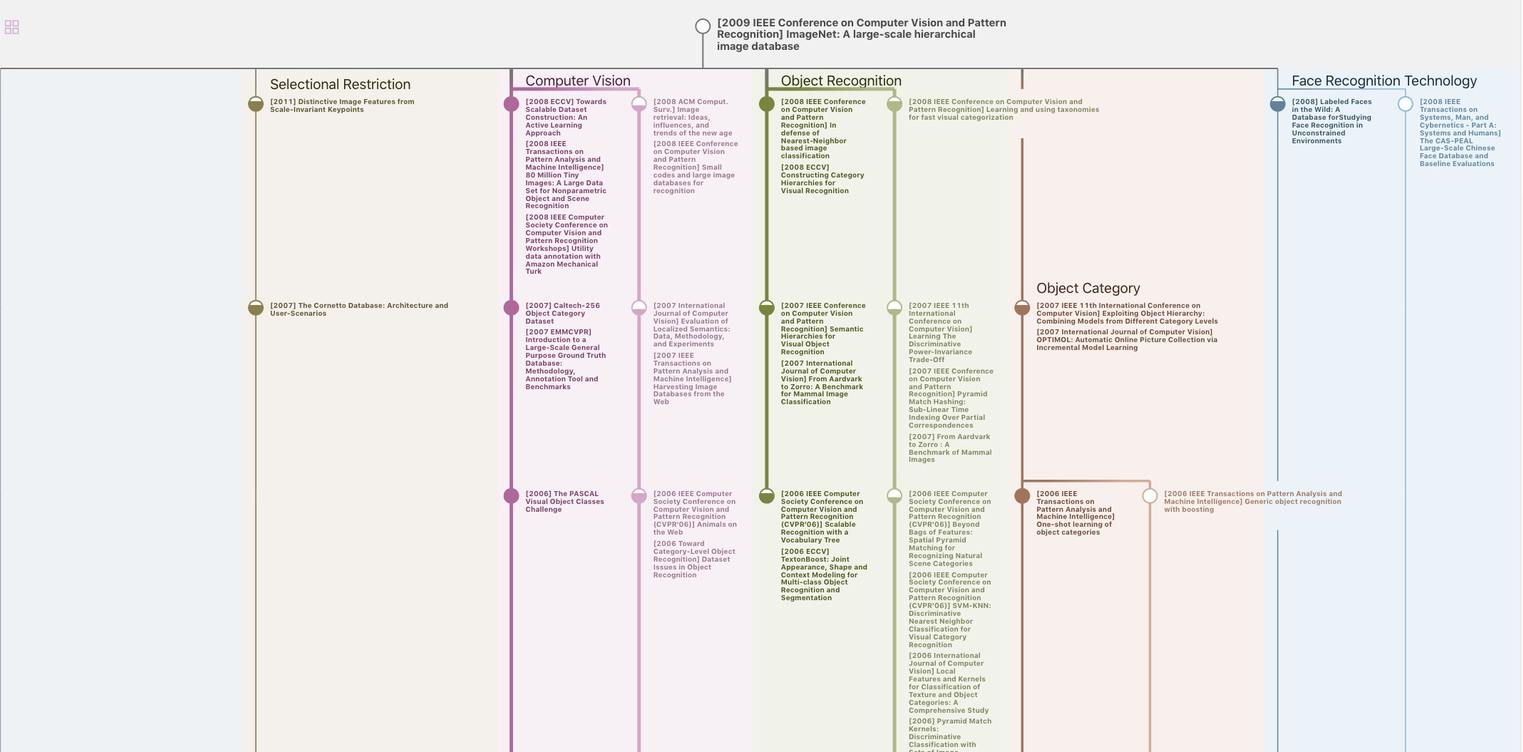
生成溯源树,研究论文发展脉络
Chat Paper
正在生成论文摘要