Metagenome Analysis of Contaminated Sediments at the DOE Hanford Site
semanticscholar(2005)
摘要
s received after January 24, 2005 Metagenome Analysis of Contaminated Sediments at the DOE Hanford Site Natalia Maltsev1, Tanuja Bompada1, Banu Gopalan2 (agor@ornl.gov), Shu-mei Li3, Weiwen Zhang3, J. Chris Detter4, Paul Richardson4, Margie Romine3, and Fred Brockman3 1Bioinformatics Group, Argonne National Laboratory; 2Computational Biology and Bioinformatics, Pacific Northwest National Laboratory, Richland, WA; 3Microbiology Group, Pacific Northwest National Laboratory, Richland, WA; 4Genomics Division, Lawrence Berkeley National Laboratory, Berkeley, CA Technologies are needed to make improved inferences of microbial community function from metagenome sequence. Most microbes have evolved multiple mechanisms (programs) to capture energy. The cells’ immediate biochemical and geochemical environment determines the regulatory networks that are engaged to run the best program to capture energy. Bulk extraction of RNA and analysis on microarrays largely destroys the spatial and functional linkages that are the key to understanding how communities interact. Therefore, a critical need for understanding the details of how multi-species microbial communities interact at the cellular level to generate a functional output, is the ability to interrogate the microscopic spatial organization of gene expression in these multi-species microbial communities. The most likely methods to accomplish this objective are (1) robotic single (prokaryotic) cell “picking” by laser catapulting microscopy followed by RNA extraction, whole transcriptome amplification (if required), and probing and/or sequencing; or (2) mRNA-targeted non-PCR based fluorescence in situ hybridization (mRNA-FISH). A second critical technology is the use of high-end computing to utilize massive amounts of metagenome sequence to design optimal phylogeneticallyconstrained function-oriented oligonucleotide probes for both of these approaches. The goals of this newly funded project are to: • Develop mRNA-targeted non-PCR based fluorescence in situ hybridization (mRNA-FISH) using soluble and nanoparticle near-infrared (low noise) probes coupled to advanced microscopy able to detect a very small number of photons • Use grid-based computational tools to analyze community metagenome data to develop hypotheses regarding the functional processes and linkages occurring in the multi-species community, and for design of a suite of phylogenetically-constrained metabolic function “signature” probes. The results presented in this poster relate to the second goal. As an initial exercise, we are analyzing metagenome sequence produced in a previous Microbial Genome Project. That project pooled enrichments from contaminated sediments beneath a leaking high-level radioactive waste tank at the DOE Hanford Site. Because biomass levels were very low (~104 cells per gram), a variety of enrichments were pooled in order to have adequate DNA to construct a clone library for sequencing. Most of the enrichments produced so little biomass that DNA concentrations were inadequate (in 2002) for constructing clone libraries. In 2003, a clone library was made from an enrichment pool that had the highest amount of DNA (only 750 nanograms). Community DNA’s were also extracted in 2002 from pools of enrichments derived from more contaminated sediments, and in light of recent technological advancements clone libraries are now being constructed from those DNA samples (5 50 nanogram amounts) by Lucigen Corporation (Madison, WI). Here we report on the preliminary metagenome analysis of the clone library constructed in 2003. Although the initial analysis reported here is a very small amount of sequence, we plan a minimum of 100-fold higher amounts of metagenome sequence from each library currently under construction. A total of 2,887 bacterial clones were sequenced and yielded a total of 7,071 hits representing 489 EC classes and 113 KEGG maps. At least one gene was present for synthesis of 18 of the 20 amino acids. Pathways in which ten or more genes in the pathway were present include metabolism of purines, pyrimidines, aminoacyl-tRNA, glycolysis/gluconeogenesis, pyruvate, starch, glycerolipid, porphyrin, glycine/serine/threonine, arginine/proline; and phenylalanine/tyrosine/tryptophan. Protein hits were largely consistent with amplified and sequenced 16S rDNA phylogenies from both pooled enrichments and sediments. The 16S data from pooled enrichments showed 10 genera from the Micrococcineae, Propionibacterineae, and Steptomycineae suborders within the Actinobacteria (high GC Gram positive) phylum; and one genera (Pseudomonas) within the gamma class of the Proteobacteria phylum). The protein hits were 68% Proteobacteria, 30% Actinobacteria, and 3% to the Bacilli and Clostridia classes of the Firmicutes (low GC Gram positive) phylum. The Pseudomonas species detected in the DNA and protein hits are nitrate-reducers and are rare or absent in pristine, deep subsurface sediments at the Hanford Site; however, their presence is consistent with nitrate being the predominant inorganic contaminant in the sediments. A web site has been constructed displaying taxonomic analysis of the metagenome; views of each contig including CDS information, potential functions, and relevant metabolic pathways; metagenome metabolic reconstruction; metabolic pathways indexed by similarity to organisms; and list of KEGG map identifications linked to organisms. Visualizations of the data using PNNL-developed Biological Data Fusion and OmniViz software will be shown. Interesting findings include (1) quite low and amino acid identities (and e-scores) for hits to the Actinobacteria and Firmicutes, suggesting a relatively novel component of this metagenome in comparison to current microbial and metagenome sequence from these phyla, and (2) a strong over-representation of transmembrane transport protein hits in the metagenome sequence. The relevance of these findings is being analyzed in detail. Future work includes metagenome sequence analysis of the very highly contaminated Hanford sediments and identification of phylogenetically-constrained metabolic function “signature” probes. SAXS/WAXS Studies of σ54-Dependent AAA+ ATPases: Insights about Signal ransduction and Motor Function. T B. Tracy Nixon (btn1@psu.edu), Baoyu Chen, Michaeleen Doucleff, David E. emmer, Timothy R. Hoover, and Elena Kondrashkina. W 1) Biochemistry and Molecular Biology, Penn State University, University Park, PA 16802 USA; 2) Chemistry, University of California, Berkeley, CA USA; 3) Lawrence Berkeley National Lab, Berkeley, CA USA; 4) Microbiology, University of Georgia, Athens, GA USA; 5) BioCAT at APS/Argonne National Lab, Illinois Institute of Technology, 9700 South Cass Ave, Argonne, IL 60439, USA. AAA+ ATPases are molecular motors that provide important biological functions in all kingdoms of life. We are still learning how their actions are controlled and how they perform mechanical work. The most prevalent information processing system in bacteria, two-component signal transduction, is sometimes used to regulate the assembly AAA+ ATPase machines that regulate transcription by the σ54-form of RNA polymerase. Powerful and complementary microscope technologies are being developed under the GTL project ‘Microscopes of Molecular Machines (M3): Structural Dynamics of Gene Regulations in Bacteria’ (Carlos Bustamante, PI) to study the structure and changes that occur when multi-protein molecular machines such as AAA+ ATPases are formed. The technologies (cryo-electron microscopy, atomic force microscopy, optical tweezers, and single-molecule fluorescence microscopy) look at molecular machines from different but complementary perspectives. Cryo-electron microscopy can, for example, form visual images of an entire molecular complex, while single-molecule fluorescence can show real time formation of complexes as a result of fluorescence signals that can be seen as specific proteins come in contact with each other. Although static light scattering from molecules in solution occurs from molecules in all possible orientations, shape information is available to about 5 Angstroms resolution. The BioCAT beamline 18ID of the Advance Photon Source in Chicago is well suited for collecting such data, especially for large molecules for which very low Q data are desired. The research presented in this poster demonstrates how solution structures derived ab initio from smalland wide-angle X-ray scattering (SAXS/WAXS) data complement the microscopy approaches (comparable cryo-electron microscopy data will be presented separately by Sacha de arlo and Eva Nogales). C We have collected scattering data for several proteins or protein fragments of DctD, NtrC, NtrC1 and PspF proteins, four such AAA+ ATPases. Solution structures determined from the scattering data give us insight into regulation and function of these molecular motors. In one case, a regulatory domain adopts two homo-dimeric forms, alternately repressing or derepressing motor assembly by adjacent ATPase domains; in another case, regulatory and ATPase domains cooperate to stabilize the assembled motor. Structures of ATPase in the presence of nucleotide analogs promise to reveal subdomain reorientations that are coupled with conformational changes in the ‘second region of homology’ and pore region of the ring shaped motors to mediate interaction with the target protein, σ54. Scattering data also yield preliminary models to explain how σ54 binds tightly to the activator in the transition state for ATP hydrolysis. Use of the Advanced Photon Source was supported by the Department of Energy under contract W-31-109-ENG-38. BioCAT is a Research Center supported by National Institutes of Health, grant RR08630. Cell-Free Protein Synthesis for High-Through-Put Proteomics MacConnell Research Corporporation Evan Dushman, Randal Sivila, and Jennifer Holmes and William P. MacConnell The production of proteins from cloned DNA sequence
更多查看译文
AI 理解论文
溯源树
样例
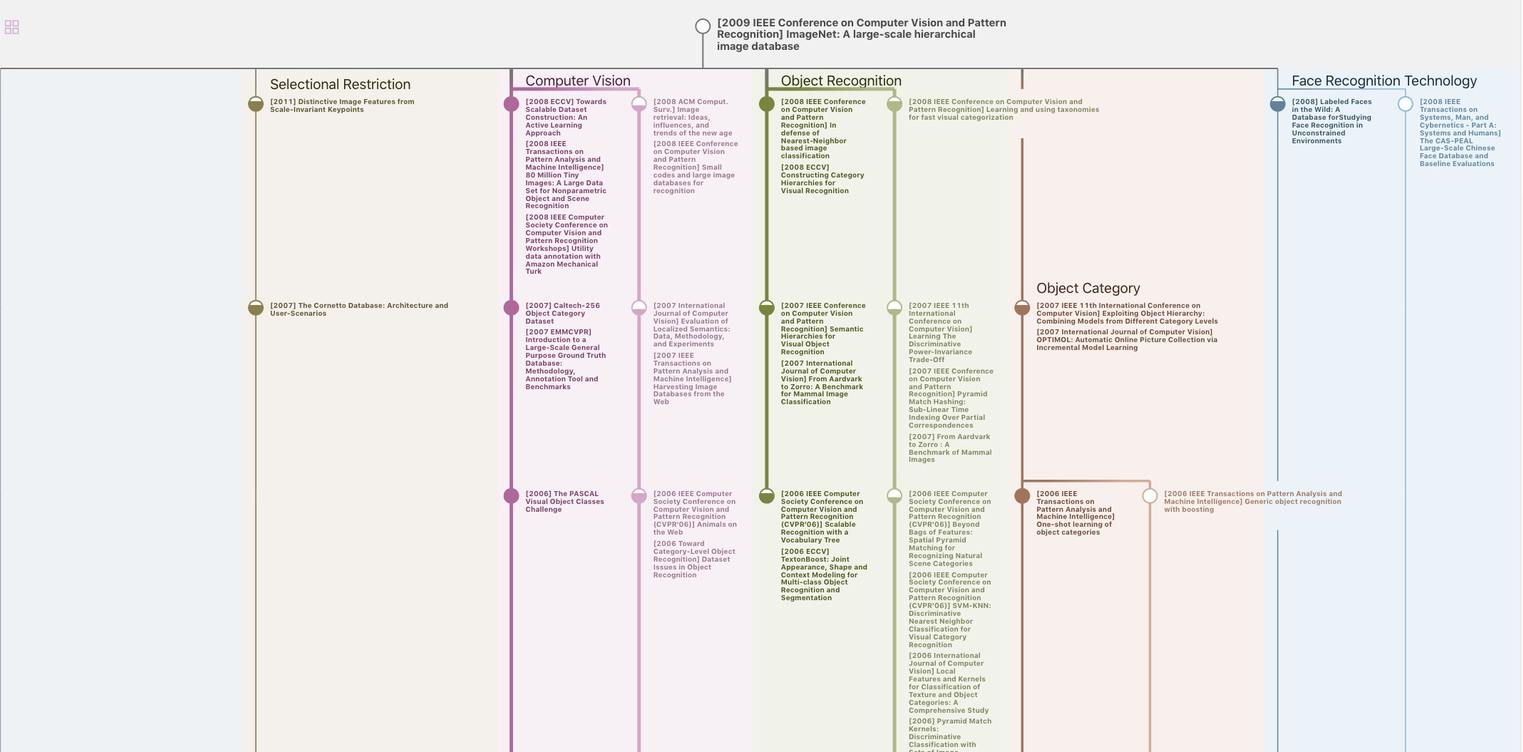
生成溯源树,研究论文发展脉络
Chat Paper
正在生成论文摘要