High-Throughput Molecular Cancer Cell Line Characterization Using Digital Multiplex Ligation-Dependent Probe Amplification for Improved Standardization of in Vitro Research
The Journal of Molecular Diagnostics(2020)
摘要
Tumor cell lines are widely used for cancer research, but challenges regarding quality control of cell line identity, cross contamination, and tumor somatic molecular stability remain, demanding novel approaches beyond conventional short tandem repeat profiling. A total of 21 commonly used multiple myeloma cell lines obtained from public repositories were analyzed by digital multiplex ligation-dependent probe amplification (digitalMLPA) to characterize germline single-nucleotide polymorphisms, insertions/deletions, and somatic copy number aberrations (CNAs). Using generated profiles and an in-house developed analytical pipeline, blinded experiments were performed to determine capability of digitalMLPA to predict cell line identity and potential spike-in DNA contamination in 41 anonymized cell line samples. The dominant cell line was correctly identified in all cases, and cross contamination was correctly detected in 33 of 37 samples with spike-in DNA; there were no false-positive predictions. The four samples in which spike in was not detected all carried low levels of contamination (1%), whereas levels of contamination ≥5% were correctly identified in all cases. Unsupervised clustering of CNA profiles identified shared commonalities that correlated with initiating Ig heavy locus translocation events. Longitudinal CNA assessment of nine cell lines revealed changes under standard culturing conditions not detected by insertion/deletion profiling alone. Results suggest that digitalMLPA can be utilized as a high-throughput tool for advanced quality assurance for in vitro cancer research. Tumor cell lines are widely used for cancer research, but challenges regarding quality control of cell line identity, cross contamination, and tumor somatic molecular stability remain, demanding novel approaches beyond conventional short tandem repeat profiling. A total of 21 commonly used multiple myeloma cell lines obtained from public repositories were analyzed by digital multiplex ligation-dependent probe amplification (digitalMLPA) to characterize germline single-nucleotide polymorphisms, insertions/deletions, and somatic copy number aberrations (CNAs). Using generated profiles and an in-house developed analytical pipeline, blinded experiments were performed to determine capability of digitalMLPA to predict cell line identity and potential spike-in DNA contamination in 41 anonymized cell line samples. The dominant cell line was correctly identified in all cases, and cross contamination was correctly detected in 33 of 37 samples with spike-in DNA; there were no false-positive predictions. The four samples in which spike in was not detected all carried low levels of contamination (1%), whereas levels of contamination ≥5% were correctly identified in all cases. Unsupervised clustering of CNA profiles identified shared commonalities that correlated with initiating Ig heavy locus translocation events. Longitudinal CNA assessment of nine cell lines revealed changes under standard culturing conditions not detected by insertion/deletion profiling alone. Results suggest that digitalMLPA can be utilized as a high-throughput tool for advanced quality assurance for in vitro cancer research. Human cancer cell lines are indispensable tools for a wide range of research applications from basic biology to preclinical drug profiling, the outcomes of which frequently feed into decision-making processes of research and development programs that can ultimately impact patient care. Recently, the degree of interlaboratory and intralaboratory variation in key molecular features of widely used cell lines, often established decades ago, has been described, including occurrence of contamination, highlighting the urgent need for improved and applicable quality control tools for cell line identification and molecular characterization.1Vaughan L. Glänzel W. Korch C. Capes-Davis A. Widespread use of misidentified cell line KB (HeLa): incorrect attribution and its impact revealed through mining the scientific literature.Cancer Res. 2017; 77: 2784-2788Crossref PubMed Scopus (34) Google Scholar, 2Lorsch J.R. Collins F.S. Lippincott-Schwartz J. Fixing problems with cell lines.Science. 2014; 346: 1452-1453Crossref PubMed Scopus (128) Google Scholar, 3Almeida J.L. Cole K.D. Plant A.L. Standards for cell line authentication and beyond.PLoS Biol. 2016; 14: e1002476Crossref PubMed Scopus (76) Google Scholar Short tandem repeat profiling is widely used for cell line identification, but has inherent limitations in terms of number of markers assessed and varying sensitivity regarding detection of minor contaminants. Furthermore, short tandem repeat typing does not provide information about key somatic molecular tumor features. This article describes a novel approach, using digital multiplex ligation-dependent probe amplification (digitalMLPA) for combined assessment of insertions/deletions and single-nucleotide polymorphisms (SNPs) for cell line identification and tumor-specific copy number aberration (CNA) profiling for molecular characterization of cell lines, using human multiple myeloma (MM) cell lines as a representative example. High sensitivity for detection of cross contamination is demonstrated in blinded experiments (K.M., L.A., and M.S.), identification of cell line–specific CNAs, and their longitudinal changes in long-term cultures, highlighting digitalMLPA's potential for application in high-throughput cell line quality control and management, in particular for laboratories working with different cell lines. MM cell line DNA samples used in this study were purchased directly from Leibnitz Institute German Collection of Microorganisms and Cell Cultures (Braunschweig, Germany) for 18 cell lines (AMO-1, ARH-77, COLO-677, EJM, IM-9, JJN-3, KMS-12-BM, KMS-12-PE, L-363, LOPRA-1, LP-1, MOLP-2, MOLP-8, NCI-H929, OPM-2, RPMI-8226, SK-MM-2, and U-266) by MRC Holland (Amsterdam, the Netherlands). DNA for nine cell lines, some nominally identical with MRC obtained cell lines, were provided by the Myeloma Molecular Therapy Team at the Institute of Cancer Research (ICR; London, UK). These had been obtained as viable cells from the Leibnitz Institute German Collection of Microorganisms and Cell Cultures (JJN-3, KMS-12-BM, L-363, LP-1, NCI-H929, and RPMI-8226), Japanese Collection of Research Bioresources Cell Bank (National Institutes of Biomedical Innovation, Health and Nutrition, Tokyo, Japan; KMS-11), and European Collection of Authenticated Cell Cultures (Public Health England, Salisbury, UK)/American Type Culture Collection (Manassas, VA; JIM-3, MM1.S, MM1.R) repositories, expanded and DNA extracted from early (<10) passages. Two single-blinded batches of samples for the cell line contamination study were prepared, one by MRC Holland (L.A.) and one by ICR (K.M.), containing either DNA from a single cell line or spike-in cell line DNA from two different cell lines with varying proportions (1% to 50%) of spike-in DNA. The external blinded samples 1 to 12 were prepared at ICR (K.M.) and sent to MRC Holland labeled with unique pseudonymized identifiers (numbers 1 to 12) only. Analysis was performed by MRC Holland (M.S. and I.C.), blinded to the cell line information and cell line identity, and spike-in estimates were transferred to ICR using anonymized labels, which were then matched with original cell line/spike in. Blinded samples 13 to 45 were prepared at MRC Holland by a laboratory scientist (L.A.), labeled with unique pseudonymized identifiers, which were used for digitalMLPA analysis. Data were analyzed separately by a bioinformatician using pseudonymized identifiers, and results were passed back to the laboratory scientist for depseudonymization and matching of results. DigitalMLPA is a next-generation sequencing–based multiplex ligation-dependent probe amplification variant that allows analysis of up to 1000 MLPA probes in a single reaction and the amplicon quantification by Illumina (San Diego, CA) sequencers. DigitalMLPA was performed, as described previously.4Benard-Slagter A. Zondervan I. de Groot K. Ghazavi F. Sarhadi V. Van Vlierberghe P. De Moerloose B. Schwab C. Vettenranta K. Harrison C.J. Knuutila S. Schouten J. Lammens T. Savola S. Digital multiplex ligation-dependent probe amplification for detection of key copy number alterations in T- and B-cell lymphoblastic leukemia.J Mol Diagn. 2017; 19: 659-672Abstract Full Text Full Text PDF PubMed Scopus (18) Google Scholar Briefly, DNA sample mixed with sample identification barcode was denatured, followed by overnight hybridization of digitalMLPA probes and ligation of hybridized probes. Ligated probes were further PCR amplified, and the PCR products of all samples were combined and loaded to MiSeq or MiniSeq Illumina sequencer. In the current study, digitalMLPA was used to perform profiling of 254 SNPs, in MM cell line–derived DNA samples by using a dedicated digitalMLPA probemix that contains 45 probes for input DNA and assay quality control alongside 254 SNP-specific probes, and to detect common copy number alterations in MM cell lines with total of 282 target copy number probes included in the newly developed research version of D006-X2 Multiple Myeloma digitalMLPA probemix (MRC Holland bv, Amsterdam, the Netherlands). This D006-X2 probemix also contains one probe specific for BRAF V600E mutation, 96 references, 45 input DNA and assay quality controls, six X and Y chromosome specific, and 39 pairs of SNP probes for sample identification and detection of sample contamination. At least triplicates of 40 ng of human genomic DNA (G1471; Promega, Mannheim, Germany) were used as a normal diploid copy number reference sample DNA in all digitalMLPA experiments. For each reaction, in total 20 to 100 ng DNA sample was used. Concentration of cell line DNA was measured by Nanodrop (Nanodrop-8000; Thermo Fisher Scientific, Waltham, MA), and subsequent dilution was made with TE buffer (T10E1 buffer: 10 mmol/L Tris-HCl, pH 8.5, and 0.1 mmol/L EDTA) whenever possible to obtain DNA samples with 10 ng/μL concentration. Cell line identification by digitalMLPA SNP profiling uses the intranormalized read ratio on a set of 254 SNP probes. The read ratios of an unknown cell line are compared against those of known cell lines. In case of an impure unknown cell line, the main cell line will be identified in this manner. The contaminating cell line and the degree of contamination may be identified on the basis of the nonzero ratios in the unknown sample for SNP probes that are absent in the main cell line, as described in detail below. Single-blinded samples of various human cancer cell lines, obtained from the Leibnitz Institute German Collection of Microorganisms and Cell Cultures for the cell line contamination study, were prepared by MRC Holland (L.A.), containing either DNA from a single cell line or spike-in cell line DNA from two different cell lines with varying proportions (1% to 10%) of spike-in DNA. Blinded samples were prepared at MRC Holland by a laboratory scientist (L.A.) and labeled with unique pseudonymized identifiers, which were used for digitalMLPA analysis. Data were analyzed separately by a bioinformatician (I.C.) using pseudonymized identifiers, and results were passed back to the laboratory scientist for depseudonymization and matching of results. SNP profiling of cell lines was performed in multiple steps. In the first analysis step, FASTQ conversion was performed, where each read of MiSeq FASTQ output file was assigned to a particular reaction (using the barcode sequence) and to a particular digitalMLPA probe. After FASTQ conversion, intrasample normalization was done to normalize the read count for each SNP-specific probe in two steps: for each sample, the median total reference probe read count was calculated, and the read count of each probe is divided by the median total reference probe read count:Rsp=CspMedianr=1m(Csr)(1) Where Csp is the read count of probe p in sample s, Csr is the read count of reference probe r in sample s, and Rsp is the (within-sample) ratio of probe p in sample s. For an unidentified sample, Rsp ratios of the 254 SNP probes were compared with the ratios in known cell lines. For each known cell line, the sum of squares of the differences between the ratios was calculated:SSc=∑p=1254(Rcp−Rup)2(2) Where Rcp is the ratio of known cell line c on SNP probe p and Rup is the ratio of the unidentified sample u on the same SNP probe p. The known cell line c with lowest (near 0) sum of squares SSc was the best identification for sample u. The same method was also used to identify the predominant cell line from a mix-up of two multiple myeloma cell line DNA samples. In that case, SSc will be further from 0, depending on the degree of impurity. Once the predominant cell line was identified, sample impurity became apparent when investigating the SNP probes that have an Rsp ratio of 0 in the pure cell line of the main ingredient. Such SNP probes were called informative probes. Any nonnegligible ratios (Rsp ≥ 0.005) in the unknown sample u on such informative probes were indicators of a cell line impurity. By investigating the informative probes, it was possible to identify which cell line caused the impurity, given that there was information on that cell line SNP profile. For each cell line, an informative SNP measure was calculated, as follows:mc=∑i=1nif(Rui>0;if(Rci>0;1;−1);if(Rci=0;1;0))(3) where Rui is the ratio of the contaminated sample u on informative probe i and Rci is the ratio of the potential contaminant cell line c on the same informative probe i, and using the structure if[logical test;(value if true);(value if false)]. In words: sum was calculated over all n informative probes. A nonnegligible ratio Rui on informative SNP probe i in mix-up sample u was considered to be likely caused by presence of contaminant. Therefore, if a nonzero ratio Rci was found in potential contaminant c, mc measure was raised by 1. However, if ratio Rci was 0, penalty of −1 was added to mc, as this cell line failed to explain the nonzero ratio Rui. When an Rui of 0 coincided with a zero ratio Rci, mc was raised by 1. When an Rui of 0 mismatched with a nonzero ratio Rci, 0 was added instead of a penalty as low contaminant concentrations might have led to some contaminant signals falling below the noise cutoff (0.5% of median total reference probe read count but at least 10 reads). Finally, cell line c with the highest mc was considered as the most likely candidate for the contaminant. Once the most likely predominant and the contaminating cell line were identified, a percentage of contamination was estimated as follows:Pc=∑i=1nRuiRcin(4) where Rui is the ratio of the contaminated sample u on informative probe i and Rci is the ratio of the contaminant cell line c on the same informative probe i, and i is each of the n probes that have an Rsp ratio of 0 in the pure cell line of the main ingredient, but not in the contaminant. Likewise, the percentage of the main ingredient was estimated by:Pm=∑i=1nRuiRmin(5) where Rmi is the ratio of the main ingredient m on informative probe i, and i is each of the n probes that have an Rsp ratio of 0 in the pure cell line of the contaminant but not in the main ingredient. Ideally, Pm should approach (1 − Pc). Above described estimation of percentage depends strongly on the number of informative probes and can vary greatly depending on the cell line content. Read counts of all SNPs per cell line were converted to distances with the maximum number of 141 unique SNP values. A heat map was generated using R version 3.6.1 (https://www.R-project.org, last accessed April 16, 2020) and Rstudio version 1.2.5001 (http://www.rstudio.com, last accessed April 16, 2020). A 142-step color gradient was generated with 0 set to white and the maximum value of 141 set to black using the R package RColorBrewer (https://CRAN.R-project.org/package=RColorBrewer, last accessed April 16, 2020) and subsequently plotted using the R package pheatmap (https://CRAN.R-project.org/package=pheatmap, last accessed April 16, 2020) using supervised clustering. For copy number characterization, digitalMLPA experiments using D006-X2 probemix were analyzed with in-house software by MRC Holland, as previously described.4Benard-Slagter A. Zondervan I. de Groot K. Ghazavi F. Sarhadi V. Van Vlierberghe P. De Moerloose B. Schwab C. Vettenranta K. Harrison C.J. Knuutila S. Schouten J. Lammens T. Savola S. Digital multiplex ligation-dependent probe amplification for detection of key copy number alterations in T- and B-cell lymphoblastic leukemia.J Mol Diagn. 2017; 19: 659-672Abstract Full Text Full Text PDF PubMed Scopus (18) Google Scholar The FASTQ is converted to read counts, and subsequently to ratios. The final analyzed data show the read ratio–relative number of reads for each probe in each reaction, as compared with reference reactions—commercially obtained blood-derived genomic DNA of male donors (Human Genomic DNA: G1471; Promega), where ratio of 1.0 corresponds to two copies of probe's target DNA (n = 2), a read ratio of 0.5 corresponds to a single copy, and a read ratio of 1.5 corresponds to three copies in a homogeneous sample with 100% tumor cell percentage consisting of only one major clone. To investigate the molecular subgroups represented by the cell lines tested, CNA profiles were clustered using R version 3.6.1 (https://www.R-project.org, last accessed April 16, 2020), Rstudio version 1.2.5001 (http://www.rstudio.com, last accessed April 16, 2020), and the ComplexHeatmap package (Bioconductor release 3.10, https://bioconductor.org/packages/ComplexHeatmap, last accessed April 16, 2020) using k-means clustering.5Gu Z. Eils R. Schlesner M. Complex heatmaps reveal patterns and correlations in multidimensional genomic data.Bioinformatics. 2016; 32: 2847-2849Crossref PubMed Scopus (2034) Google Scholar First, the optimal number of clusters was calculated using an elbow plot, and then clustering was performed using the standard k-means algorithm. In total, nine MM cell lines (JIM-3, JJN-3, KMS-11, KMS-12-BM, L-363, MM1-R, MM1-S, NCI-H929, and RPMI-8226) were cultured in culture medium with fetal bovine serum and, if indicated, supplements, as recommended by the respective cell line repository that provided viable cells (see above). All cells were grown in humidified incubators in 5% CO2 at 37°C, passaged in recommended intervals, and kept at recommended densities. Cells were harvested after 10, 20, 30, 40, and 50 passages in RLTplus buffer (Qiagen, Hilden, Germany). DNA was extracted using the AllPrep kit (Qiagen) and sent to MRC Holland for copy number and SNP profiling by digitalMLPA analysis. DigitalMLPA, a high-throughput method for SNP and focused CNA assessment, was applied to profile a range of widely used human MM cell lines, the majority directly sourced from public repositories. Genetic fingerprint profiles for each of the MM cell lines were generated from SNP data and subsequently used to determine digitalMLPA capability for blinded cell line identification and detection of spike-in contamination based on algorithms specifically designed and developed for this purpose by the bioinformatics team at MRC Holland (see Materials and Methods). To test capabilities of digitalMLPA in conjunction with developed algorithms for detecting cell lines and their potential contamination, 41 samples were analyzed in a blinded manner, of which 12 were prepared by scientists at the ICR myeloma laboratory (K.M.) and 29 by scientists at MRC Holland (L.A., M.S., and I.C.). Data analysis was performed centrally by the MRC Holland bioinformatics team, who were blinded to cell identity and to whether samples contained a unique cell line source or a potential spike in from another cell line. In total, 4 of 41 samples contained a single cell line DNA source only and no spike-in contamination. Of the 37 samples that had DNA from a second cell line spiked in, 21 of 37 had a spike in of ≥5% and 16 of 37 had a spike in of <5%, the latter specifically to test the potential limits of detection. Individual cell line and spike-in sample digitalMLPA SNP read counts are shown in Supplemental Table S1. Results in Table 1 demonstrate that the dominant cell line was correctly identified using the fingerprint profiles of all 41 samples (100%). Across all experiments, detection of any contamination by spike in with a second cell line was achieved for 34 of 37 cell lines. The three samples for which spike-in contamination was not detected contained low (1%) spike-in DNA. There were no false-positive predictions of contamination for the samples without spike in. For 29 of the 34 samples with spike in, the identity of the spiked-in cell line was correctly predicted on the basis of SNP profiles, and an estimate of the level of contamination similar to the spiked-in amount was predicted for most. The five samples for which contamination was detected but the spiked-in cell line could not be identified had low spike ins of <5%. These results led us to consider 5% as a potential high confidence cutoff for contamination detection. When excluding the samples with <5% spike-in DNA, contamination in the remaining 21 samples was detected and identity of the contaminating cell line was identified correctly for all (100%) by digitalMLPA (Table 1).Table 1Cell Line Contamination Detection by DigitalMLPA SNP Profiling in Single-Blinded Cell Line DNA Samples with and without Spike In from Another Cell LineBlindingBlinded mix-up sample IDCell lineCell line identified by digitalMLPASpike-in/contaminating cell lineSpike in/contamination predicted by digitalMLPA% Of contaminating cell line% Of contaminating cell line, as calculated by digitalMLPAExternal (ICR)1KMS-11+L-363+5122RPMI-8226+NCI-H929+433JJN-3+LP-1+50414KMS12BM+-–005JIM3+-–006MM1∗Either MM1.R or MM1.S cell line; both are derived from the same origin and have identical SNP profiles.+KMS-12-BM+147JIM3+L363+238KMS-11+MM1∗Either MM1.R or MM1.S cell line; both are derived from the same origin and have identical SNP profiles.–†Only two informative SNPs present to allow identification of contaminating cell line.,‡A contamination was detected, but the contaminating cell line was misidentified or technically not feasible to identify.409MM1∗Either MM1.R or MM1.S cell line; both are derived from the same origin and have identical SNP profiles.+-–0010RPMI-8226+KMS-11+3211RPMI-8226+JJN-3+2212LP-1+MM1∗Either MM1.R or MM1.S cell line; both are derived from the same origin and have identical SNP profiles.+51Internal (MRC Holland)13AMO-1+RPMI-8226+403814AMO-1+SK-MM-2+202215ARH-77+KMS-12-BM+10816ARH-77+OPM-2+505117COLO-677+LP-1+‡A contamination was detected, but the contaminating cell line was misidentified or technically not feasible to identify.2.5–18COLO-677+NCI-H929+303619EJM+MOLP-8+‡A contamination was detected, but the contaminating cell line was misidentified or technically not feasible to identify.5–20IM-9+LP-1+404521JJN-3+MOLP-2+2.5222KMS-12-PE+LOPRA-1+302523L-363+JJN-3+5324LOPRA-1+KMS-12-PE+10925MOLP-8+SK-MM-2+202126MOLP-8+EJM+51027NCI-H929+IM-9+302828OPM-2+IM-9+5429RPMI-8226+U-266+‡A contamination was detected, but the contaminating cell line was misidentified or technically not feasible to identify.1–30U-266+L-363+504831AMO-1+-–0032AMO-1+NCI-H929+2533EJM+MOLP-8+‡A contamination was detected, but the contaminating cell line was misidentified or technically not feasible to identify.5334IM-9+ARH-77+51235IM-9+ARH-77+1536IM-9+NCI-H929+1237LOPRA-1+JJN-3–1038LOPRA-1+KMS-12-BM–1039OPM-2+MOLP-8–1040OPM-2+EJM+1241SKMM2+JJN-3+54+, Yes; –, no; digitalMLPA, digital multiplex ligation-dependent probe amplification; ICR, Institute of Cancer Research; ID, identifier; SNP, single-nucleotide polymorphism.∗ Either MM1.R or MM1.S cell line; both are derived from the same origin and have identical SNP profiles.† Only two informative SNPs present to allow identification of contaminating cell line.‡ A contamination was detected, but the contaminating cell line was misidentified or technically not feasible to identify. Open table in a new tab +, Yes; –, no; digitalMLPA, digital multiplex ligation-dependent probe amplification; ICR, Institute of Cancer Research; ID, identifier; SNP, single-nucleotide polymorphism. For the samples with <5% spiked-in DNA, contamination was correctly detected in 12 of 16 samples and not detected (false negative) in the remaining four. On the basis of this heterogeneity in detection, SNP heterogeneity analysis of cell lines was performed. Several distinct clusters were identified, and cases for which contamination was difficult to detect tended to be within similar clusters. Different clusters were enriched for similar ethnic background of the patients from whom the respective cell lines were originally derived, as determined from publicly available information (Figure 1). Furthermore, for human cancer cell lines other than MM, detection of any contamination by spike in with a second cell line or the absence of it was achieved for 16 of 16 samples (Supplemental Table S2). The 282 CNA detection probes, curated to include frequent areas of somatic CNAs in MM, were used to molecularly characterize cell lines. Normalized digitalMLPA copy number counts per probe are provided in Supplemental Table S3. For subsequent analysis, Epstein-Barr virus (EBV)–positive cell lines and those of uncertain origin (AMO-1, ARH-77, COLO-677, and IM-9) were excluded, although including these cell lines did not fundamentally change the results (Supplemental Figure S1). Of interest, results including the above mentioned cell lines demonstrate the similarity in molecular profiles of RPMI-8226 and COLO-677, the latter identified as a contaminant cell line of RPMI-8226 in 2010.6Capes-Davis A. Theodosopoulos G. Atkin I. Drexler H.G. Kohara A. MacLeod R.A.F. Masters J.R. Nakamura Y. Reid Y.A. Reddel R.R. Freshney R.I. Check your cultures! a list of cross-contaminated or misidentified cell lines.Int J Cancer. 2010; 127: 1-8Crossref PubMed Scopus (305) Google Scholar Molecular profiles of EBV-positive cell lines IM-9 and ARH-77 showed CNA patterns atypical for MM, with absence of and unusual chromosomal location of CNAs, including del(1q) and del(3q), respectively. Next, confirmed MM cell lines were clustered on the basis of their CNA profiles. Most cell lines clustered in one of the three clusters, with EJM showing a distinct, fourth CNA profile cluster (Figure 2). Clusters differed in specific regional characteristics: cell lines in cluster3 consistently showed amplification of 1q and del(13), cluster2 was characterized by presence of copy number gain of the MYC locus, gain(11), and del(17p), and cluster1 cell lines mostly carried gain(1q) and cell lines in this cluster seemed to carry overall fewer CNAs (MM.1S/R, MOLP-8, and L-363) (Figure 2). Clusters were associated with pathogenic Ig heavy locus translocation subtypes: all cell lines in cluster3 (with the exception of LOPRA-1, for which no information is available in the public domain) carried t(4; 14), cell lines in cluster2 had t(11; 14), and those in cluster1 contained predominantly MAF or MAFB translocated cell lines. Regional deletions of chromosome 1p were present across all clusters, and CDKN2C homozygous deletion was detected in 8 of 18 cell lines (44%; no detectable copies), whereas complete loss of tested exons of FAM46C was identified in only two cell lines. As described before, TP53 deletions were detected in most cell lines, including three with complete loss of TP53 exons. EJM showed distinct and marked gain of MAP3K14 on chromosome 17q21, up to 12-fold from baseline copy number, encoding for the NF-κB signaling inducing kinase NIK. The digitalMLPA probemix contains a mutation detection probe for the canonical BRAF V600E mutation. None of the tested cell lines carried this mutation, in line with previously published data.7Sondka Z. Bamford S. Cole C.G. Ward S.A. Dunham I. Forbes S.A. The COSMIC Cancer Gene Census: describing genetic dysfunction across all human cancers.Nat Rev Cancer. 2018; 18: 696-705Crossref PubMed Scopus (450) Google Scholar In addition to germline sample identity, genetic heterogeneity in somatic tumor aberrations has been recently described, with potential impact on reproducibility of research findings. Nine commonly used MM cell lines (JIM-3, JJN3, KMS-11, KMS12-BM, L-363, MM1.R, MM1.S, NCI-H929, and RPMI-8226), obtained from public repositories in early passages, were kept in long-term culture under recommended culturing conditions for up to 50 passages; and DNA was harvested during passage after every 10 passages. The digitalMLPA probemix interrogates key candidate loci of CNA in MM but also provides a virtual karyogram across all chromosome arms. Overall, the virtual karyogram remained relatively stable throughout the 50 passages across the cell lines (Figure 3). However, there were regional changes in copy number, some of which occurred relatively early. Early changes were noted in MM1.R, where a region of chromosome 17 gained extra copies from 10 passages onward (Supplemental Figure S2), but also RPMI-8226, with copy number gain of chromosome 7 at baseline changing toward a neutral pattern after 10 passages (Supplemental Figure S2). Of note, NCI-H929 acquired additional copies of IRF4, a key myeloma oncogene on chromosome 6p over baseline from passage 30 onwards (Figure 4). Also, RPMI-8226 lost additional copies of BIRC2/BIRC3 and ATM on chromosome 11, present at baseline, beyond passage 30 (Figure 4).Figure 4Detail of focal copy number aberration drift of multiple myeloma cell lines with increasing passages. Copy number ratio profiles of digital multiplex ligation-dependent probe amplification (digitalMLPA) probes, including identifiers of genes assayed directly by digitalMLPA.
更多查看译文
AI 理解论文
溯源树
样例
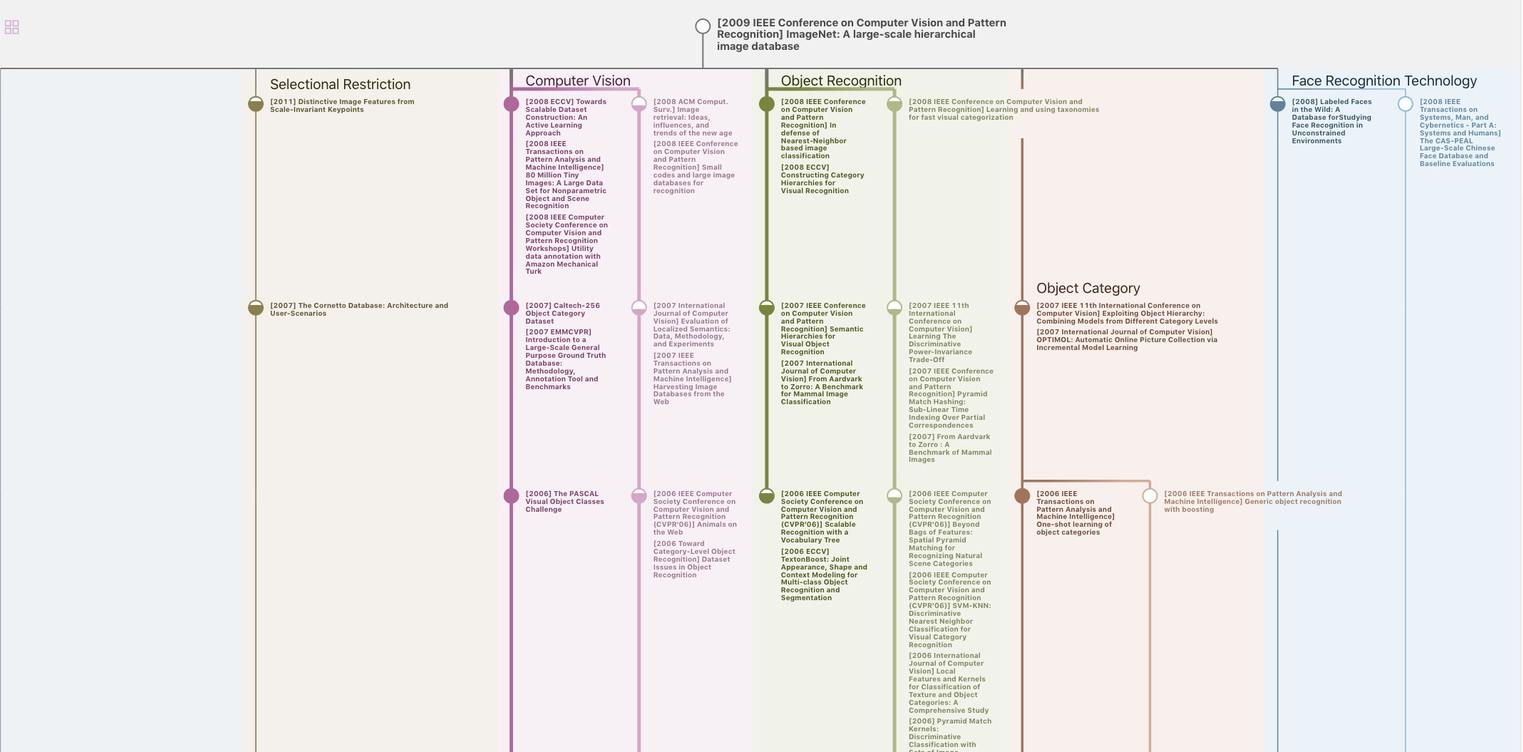
生成溯源树,研究论文发展脉络
Chat Paper
正在生成论文摘要