Stability diagram of perpendicular magnetic tunnel junction with a composite free layer
2017 IEEE International Magnetics Conference (INTERMAG)(2017)
摘要
Magnetic tunnel junctions (MTJs) have become a basic building block for various types of spintronics devices, such as magnetic random access memory (MRAM) cells, magnetic field sensors and microwave generators or detectors. There are a few ways to realize perpendicularly magnetized MTJ, which are characterized by greater thermal stability than their in-plane magnetized counterparts. Taking advantage of the interface anisotropy yields the best results, especially in terms of high tunnel magnetoresistance (TMR) measured typically in MTJs with CoFeB/MgO/CoFeB trilayer. Recent studies on perpendicular MTJ resulted in the TMR ratio exceeding 200 % [1] thanks to careful optimization of both the free layer (FL) and reference layer (RL) structure. We report on the perpendicular MTJ with a composite CoFeB/W/CoFeB FL [2,3] which is characterized by high perpendicular magnetic anisotropy and spin polarization resulting in up to 180 % TMR measured at room temperature and above 280 % TMR at low temperature. The multilayers with the following structure were deposited: buffer / SyF / spacer / CoFeB(1) / MgO(1.2) / CoFeB(t
FL
) / W(0.3) / CoFeB(0.5) / MgO(1) / capping (thicknesses in nm), with t
FL
ranging from 1 up to 1.6 nm and RA product equal to 10 Ωμm
2
. For the 1.5 nm thick CoFeB sublayer, the TMR of 180 % at room temperature and 280 % at 20 K was measured. The stability diagram was modeled based on Ref. [4]. Additionally, it was assumed that in-plane all (out-of-plane al) torque component scales linearly (quadratically) with the applied current [5]. To account for additional physical effects that contribute to the stability diagram, namely VCMA and temperature effect, the coercive field HC is scaled by the factor: HC = HK(1-V
K
V-(T/ kT)1/2) where V is the applied voltage, HK is the anisotropy field, V
K
is the VCMA coefficient and Ta is the ambient temperature. kT represents the dependence of the temperature on the coercive field. The damping factor was measured independently by the broadband FMR technique. An increase of the TMR from 140% for t
FL
= 1.1 nm up to TMR = 180% for t
FL
= 1.5 nm is explained by an increase of the spin polarization for thicker ferromagnetic layer. A rapid reduction of the TMR for t
FL
= 1.6 nm is caused by the transition of the FL magnetization vector to the sample plane. Electronic transport properties were measured in perpendicular magnetic field (see Fig. 1). It has been already established that apart from the conventional STT effect in MTJs with a relatively thin MgO barrier, the switching process can be also affected by the VCMA effect in a thin FL. To investigate the switching process in more detail, we repeated the R(V) loops measurement, with different constant magnetic field. The stability diagram was obtained in this way both at low (T = 20 K) and room temperature (T = 300 K) for the MTJ with t
FL
= 1.1 nm. The 300 K result is presented in Fig. 2. The diagram was modelled using STT, VCMA and thermal effects taken into account. In addition, for the precise derivation of the STT coefficients, the magnetization damping was calculated based on an independent FMR measurement. Fitting the experimental stability diagram to the model yielded the temperature coefficients of kT = 0.0014 T/K. This parameter was kept constant for MTJs with different t
FL
. Remaining parameters of the stability diagrams for each t
FL
were modeled independently. For t
FL
= 1.1 nm, the following STT components were obtained: all = 0.024 T/V and al = 0.02 T/V, however, we note that the modeled stability diagram is insensitive to al, which agrees with another macrospin approach based on Landau-Lifshitz-Gilbert equation presented in Ref. [6]. In-plane torque τll was thereafter recalculated and as the result we obtained τll = 4.5*10
-19
Nm/V, which agrees well with literature values of STT in the case of an in-plane MTJ [5,7]. Regarding VCMA, the best results for MTJ with t
FL
= 1.1 nm were obtained for V
K
= 0.14 1/V. Based on the following relation: V
K
= κ/μ
0
H
C
M
S
t
FL
t
B
, where t
B
= 1.2 nm is the tunnel barrier thickness, VCMA coeficient of κ = 77 fJ/ Vm was calculated, which fits well commonly measured values for CoFeB/ MgO devices [3,8]. The project is supported by Polish National Center for Research and Development grant No. LIDER/467/L-6/14/NCBR/2015 and grant Preludium UMO-2015/17/N/ST3/02276 from National Science Center, Poland.
更多查看译文
关键词
stability diagram,perpendicular magnetic tunnel junction,composite free layer,spintronics devices,magnetic random access memory,MRAM cells,magnetic field sensors,microwave generators,microwave detectors,thermal stability,interface anisotropy,tunnel magnetoresistance,optimization,perpendicular magnetic anisotropy,spin polarization,temperature effect,coercive field,VCMA coeffcient,damping factor,broadband FMR technique,ferromagnetic layer,switching process,STT model,magnetization damping,Landau-Lifshitz-Gilbert equation,tunnel barrier thickness,temperature 293 K to 298 K,temperature 20 K,size 1.5 nm,temperature 300 K,size 1.6 nm,CoFeB-MgO-CoFeB-W-CoFeB
AI 理解论文
溯源树
样例
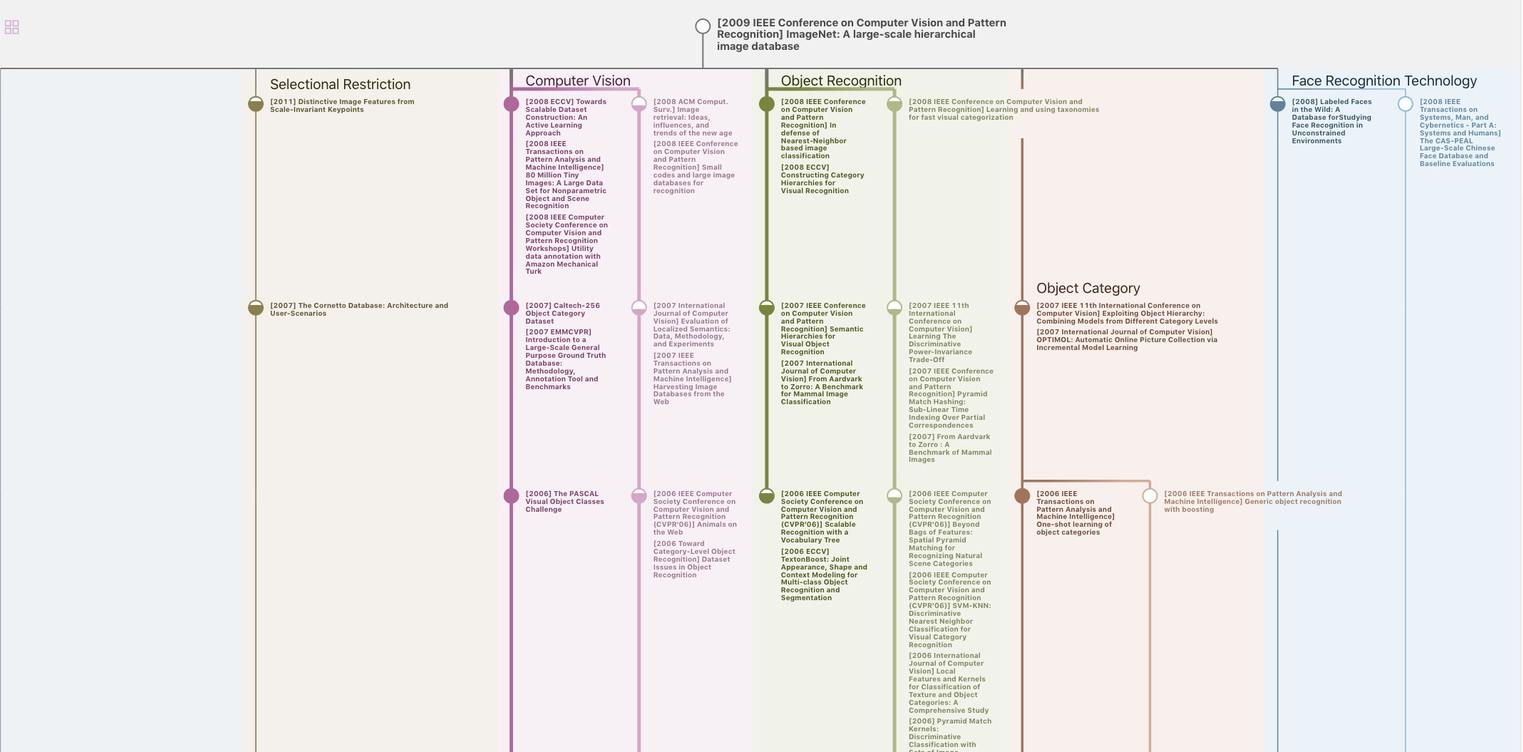
生成溯源树,研究论文发展脉络
Chat Paper
正在生成论文摘要