Preclinical validation: LV/IL-12 transduction of patient leukemia cells for immunotherapy of AML
Molecular Therapy - Methods & Clinical Development(2016)
摘要
Abstract Abstract • Introduction • Results • Discussion • Materials and Methods • References • Acknowledgements • Author information • Supplementary information Interleukin-12 (IL-12) is a potent cytokine that may be harnessed to treat cancer. To date, nearly 100 IL-12-based clinical trials have been initiated worldwide. Yet systemic administration of IL-12 is toxic. Different strategies are being developed to reduce such toxicities by restricting IL-12 distribution. Our previous studies employed lentivector-mediated expression of murine IL-12 in tumor cells and demonstrated effective protection in both mouse leukemia and solid tumor challenge models. In this study, we carried out preclinical validation studies using a novel lentivector to engineer expression of human IL-12 in acute myeloid leukemia blast cells isolated from 21 patients. Acute myeloid leukemia cells were transduced with a bicistronic lentivector that encodes the human IL-12 cDNA as a fusion, as well as a LNGFR (ΔLNGFR)/mutant thymidylate kinase cassette as a marking and cell-fate control element. A range of 20–70% functional transduction efficiencies was achieved. Transduced acute myeloid leukemia cells produced bioactive IL-12 protein and displayed dose-dependent sensitivity to the prodrug 3′-azido-3′-deoxythymidine. In vitro immortalization assays using transduced mouse hematopoietic stem cells demonstrated minimal genotoxic risk from our IL-12 vector. Scale-up transduction and cell processing was subsequently validated in a GMP facility to support our (now approved) Clinical Trial Application (CTA). Figures at a glance Displaying 7 of 7 figures | Figures index Figure 1 Efficient transduction of the OCI-AML1 cell line with LV/IL-12 and production of functional hIL-12. (a) Diagram of the LV, pDY.hIL-12.IRES.ΔLNGFR/TMPK (LV/IL-12) used in this study. LTR, long terminal repeat; Ψ, human immunodeficiency virus packaging signal; SD, 5′ splice donor site; ΔGAG, deleted group antigens; RRE, Rev responsive element; SA, 3′ splice acceptor site; cPPT, central polypurine tract; EF-1α, elongation factor 1 alpha; CO.hIL-12 (p40/p35), codon-optimized cDNA of human IL-12 β chain (p40) fused with the IL-12 α chain (p35) by a short Elastin linker; IRES, internal ribosome entry site; CO.ΔLNGFR/TMPK, codon-optimized truncated LNGFR-TMPK (F105Y) fusion; WPRE, woodchuck hepatitis virus post-transcriptional regulatory element; SIN/LTR, Self-Inactivating LTR. (b) Functional transduction efficiency of LV/IL-12 on the OCI-AML1 cell line determined by LNGFR (CD271) staining and flow cytometry. (c) Correlation of human LNGFR and IL-12 expression level in transduced cells. OCI-AML1 clones expressing different levels of LNGFR were cultured for 2 hours and the hIL-12 concentration in the supernatant was measured by ELISA. The geometric mean fluorescence intensity (Geo mean MFI) of the LNGFR signal obtained from the flow cytometry analyses was plotted against the secreted hIL-12 p70 level (ng cytokine secreted from 1 million cells in 2 hours). The data was analyzed by linear regression analysis and r2 represents the square of the correlation coefficient. LV-transduced (OCI-AML1-LV12.31) and nontransduced (NC: OCI-AML1-NC.3) OCI-AML1 clones were cultured in complete IMDM for 2 hours and the supernatant was collected. (d) Production of IFN-γ from NK-92 cells was determined by ELISA. The results are presented as the mean ± SD of triplet wells. AML1, acute myeloid leukemia1; ELISA, enzyme-linked immunosorbent assay; IFN-γ, interferon gamma; IL-12, interleukin-12; IMDM, Iscove’s Modified Dulbecco’s Medium; SD, standard deviation; LNGFR, low-affinity nerve growth factor receptor; LV, lentivector; MFI, mean fluorescence intensity; TMPK, thymidylate kinase. Figure 2 AZT-induced cell death in LV/IL-12-transduced OCI-AML1 clones. LV/IL-12-transduced (low level IL-12-producting clone: OCI-AML1-LV12.35 and high level IL-12-producing clone: OCI-AML1-LV12.31) and nontransduced (OCI-AML1-NC.2) clones were cultured in complete IMDM in the absence or presence of 20, 100, and 200 µmol/l AZT for up to 12 days. At 4, 8, and 12 days after treatment, cells were counted after Trypan blue staining. Viable cell numbers (a) and percentages of viable cells (b) were quantified. Data shown are mean ± SEM of triplet wells. AML, acute myeloid leukemia; AZT, 3’-azido-3’-deoxythymidine; IL-12, interleukin-12; IMDM, Iscove’s Modified Dulbecco’s Medium; LV, lentivector; SEM, standard error of the mean. Figure 3 In vitro growth of primary AML cells. PBMCs were isolated from AML patient blood and cryopreserved as described in Materials and Methods. 2 × 106 frozen cells were thawed and cultured in complete AIM V for up to 10 days. Viable cell numbers were quantified at the time of thawing and every 2 days post-thaw. Depending on their growth profiles, samples were grouped into three categories: (a) proliferated or at least maintained the number of input cells at 6 days post-thaw; (b) did not expand or maintain original cell numbers, but slowly recovered; (c) completely collapsed after 6 days of thaw. AML, acute myeloid leukemia; PBMC, peripheral blood mononuclear cells. Figure 4 LV transduction efficiency and VCN in primary AML cell samples. Differential transduction conditions were tested. Two days after thaw AML cells were transduced with LV/IL-12 either (a) at different MOIs (0, 2, 5, 10, 20 and 50), or (b) with 1 or 2 rounds of infections (as described in “Materials and Methods”). The percentage of LNGFR+ cells was determined by flow cytometry. Seventeen primary AML cell samples were mock-transduced or transduced once at MOI 10. The percentage of cells expressing LNGFR is shown in (c). Data shown are mean ± SEM. (d) The average VCN/genome of above bulk-transduced cells was determined by real-time PCR (as described in “Materials and Methods”). AML, acute myeloid leukemia; AZT, 3′-azido-3′-deoxythymidine; IL-12, interleukin-12; LNGFR, low-affinity nerve growth factor receptor; LV, lentivector; MOI, multiplicity of infection; PCR, polymerase chain reaction; SEM, standard error of the mean; VCN, viral copy number. Figure 5 IL-12 production from LV/IL-12-transduced primary AML cells. Three days after LV transduction, cells were washed and incubated in complete AIM V medium for 2 hours. Supernatant was collected and hIL-12 p70 protein levels were measured by ELISA. Results shown are mean ± SEM. AML, acute myeloid leukemia; ELISA, enzyme-linked immunosorbent assay; IL-12, interleukin-12; LV, lentivector; SEM, standard error of the mean. Figure 6 LV/IL-12 integration profile and genotoxicity analysis. Mouse lineage marker negative (Lin−) BM cells were transduced with LV/IL-12, or two control vectors: γ-retroviral vector RSF91 and lv-SF. Cell growth in each well was screened and scored (as described in “Materials and Methods”). (a) The number of wells that showed clonal expansion was used to calculate the replating frequency (RF); the RF per VCN was determined for each vector. The mean of RF/VCN is indicated by a bar. If no clonal outgrowth from any well in one plate was observed, the assay was scored as negative. Additional values (dots in light gray) of assays from previous results that used the same conditions following the same SOPs was included here to allow for statistical analysis. (b) The two clones from LV/IL-12-transduced cells that showed obvious outgrowth were processed to genomic DNA extraction and LAM-PCR analysis (as described in “Materials and Methods”). PCR products were run on a 2% agarose gel (Lane 1 and 6: 1 kb DNA ladder; 2: empty; 3 and 4: IL-12; 5: Mock). The DNA bands were excised and sequenced. Green numbers indicate that the sequence got a definitive BLAST hit in the murine genome, whereas red numbers indicated to the sequence did not give an unambiguous BLAST result from the murine genome. BM, bone marrow; IL-12, interleukin-12; LV, lentivector; LAM-PCR, linear amplification-mediated PCR; PCR, polymerase chain reaction. Figure 7 GMP scale-up production of LV-transduced primary AML cells. (a) Cryopreserved (under GMP) patient PBMCs were thawed and cultured for 2 days in a Cell Therapy Facility. Live cells were isolated and transduced with LV/IL-12 at MOI = 10. (b) Three days after transduction, cells were washed and resuspended in infusion buffer (Plasma Lyte A plus 0.5% human serum). An aliquot of cells were analyzed by flow cytometry to determine transduction efficiency by measure human LNGFR staining. (c) The stability of the cell product was tested at indicated time post-harvest and represented as total viable cells or % viable cells. AML, acute myeloid leukemia; GMP, good manufacturing practices; IL-12, interleukin-12; LV, lentivector; LNGFR, low-affinity nerve growth factor receptor; MOI, multiplicity of infection; PBMCs, peripheral blood mononuclear cells. Introduction Abstract • Introduction • Results • Discussion • Materials and Methods • References • Acknowledgements • Author information • Supplementary information Interleukin-12 (IL-12) activates several immune responses, such as cytotoxic immunity, Th1 cytokine secretion, and antibody production.1,2,3,4,5,6 The active human IL-12 (hIL-12) p70 protein functions as a heterodimer comprised of two covalently-linked subunits, p35 and p40.7 IL-12 p70 is secreted mainly by dendritic cells, macrophages, neutrophils, and B lymphocytes.1,2,3,5 IL-12 also acts as a growth factor to promote activated NK and T cell proliferation.8 Furthermore, angiogenesis, which often benefits tumor growth and metastasis, can be inhibited by IL-12.9 In animal models including solid tumors and hematologic malignancies, IL-12 addition is an effective antitumor therapy.10 Because of this, ~70 IL-12-based clinical trials have been initiated to date; among them more than 20 involve gene or cell therapies (http://www.clinicaltrials.gov). The early clinical studies demonstrated that systemic administration of recombinant hIL-12 into patients led to high toxicities with only marginal therapeutic responses in most cases.11,12,13,14 Side products including interferon-γ (IFN-γ) and tumor necrosis factor alpha (TNFα) were believed to contribute to such toxicities.15,16 Various strategies are being developed to reduce toxicities by limiting IL-12 distribution. Direct intratumoral injection of IL-12-expressing plasmids,14,17,18,19 viral vectors20,21 and autologous cells engineered to express IL-12,22,23 have been applied to treat lymphomas, digestive cancers, head and neck cancer, prostate cancer, ovarian cancer, breast cancer, melanoma, Merkel cell cancer, and certain other metastatic cancers (www.clinicaltrials.gov). Some of these studies demonstrated potent responses with tolerable toxicities. Acute myeloid leukemia (AML) accounts for approximately one-quarter of all leukemias in adults; it is the most frequent form of leukemia in the Western world.24 Chemotherapy and bone marrow transplantation are current treatments for AML. Though most patients who receive chemotherapy achieve remission, about half will undergo relapse eventually.25 Bone marrow transplantation is restricted by the lack of availability of matched donors as well as potential post-transplant mortality; there are also age-restrictions on its use in some jurisdictions. Our previous studies showed that injection of murine acute lymphoblastic leukemia (ALL) cells transduced to engineer expression of mouse IL-12 protected animals from challenge by nonmodified tumor cells.26 Only a small proportion (~1%) of IL-12-producing ALL cells were required for tumor rejection as long as the IL-12 expression levels reached a certain threshold. Leukemia cell-mediated antitumor immunity was highly specific, as animals challenged with a different leukemia cell line were not protected by the initial lentivector (LV)-transduced ALL cells. We also confirmed this in other tumor models including Squamous-cell carcinoma, Lewis lung carcinoma, prostate cancer, and osteosarcoma.27 These results prompted us to enact clinical translation of tumor cell-based LV/IL-12 immunotherapy targeting AML. In this study, we first constructed a novel LV that engineers a fusion form of hIL-12 along with a downstream cell fate-control (or “suicide”) element: mutant thymidylate kinase (TMPK). This mutant enzyme demonstrated increased activity to phosphorylate the nontoxic prodrug 3′-azido-3′-deoxythymidine (AZT), which, upon phosphorylation, can incorporate into and terminate DNA synthesis.28,29 We also developed optimized protocols to scale-up this schema for clinical implementation. With our protocol, we successfully transduced both human AML cell lines and primary patient AML cells with a near clinical-grade LV. A single overnight infection with a high titer virus led to functional transduction efficiencies of 20–70% in primary AML cell samples with an average of 0.29 viral copy number (VCN) per cell. Transduced AML cell lines were sensitive to AZT treatment. Furthermore, the therapeutic LV displayed minimal genotoxicity and no overt cytotoxicity in mouse bone marrow cells. Our study demonstrated the feasibility and safety of modifying patient leukemia cells ex vivo with a therapeutic LV to produce IL-12 and support our clinical implementation of this approach. Results Abstract • Introduction • Results • Discussion • Materials and Methods • References • Acknowledgements • Author information • Supplementary information In vitro transduction of AML cell lines with a “near-clinical grade” LV engineering expression of functional hIL-12 We constructed a novel bicistronic LV that contains the cDNA of a fusion form of hIL-12 (p40-p35) as the first component and the cDNA of a truncated human low-affinity nerve growth factor receptor (ΔLNGFR) fusion in-frame to a mutant human TMPK (TMPKF105Y) as the second component downstream of an internal ribosome entry site (IRES) (Figure 1a). The truncated LNGFR (ΔLNGFR, CD271) serves as a cell surface marker, allowing detection of functionally transduced cells. The mutant TMPK is a cell-fate control protein that can convert the prodrug AZT into a toxic form and either stop cell replication or induce apoptosis.29 ΔLNGFR/TMPK ensures a one-to-one correlation of cell labeling and cell killing, and has been proven to be functional both in vitro and in vivo.29 In addition, both cDNA components of the hIL-12 fusion and the ΔLNGFR/TMPK cassette were codon-optimized to further enhance expression in transduced human cells. “Near clinical-grade” virus was produced under contract by the Indiana University Vector Production Facility (IUVPF) in Indianapolis (see “Materials and Methods”). This facility also made the clinical-grade vector for our upcoming phase 1 trial with AML patients. The “near-clinical grade” and “clinical-grade” vectors are manufactured following identical procedures although the level of quality control/assurance is more extensive for the clinical-grade vector. Quality control was performed by the IUVPF and at the UHN. Figure 1 Efficient transduction of the OCI-AML1 cell line with LV/IL-12 and production of functional hIL-12. (a) Diagram of the LV, pDY.hIL-12.IRES.ΔLNGFR/TMPK (LV/IL-12) used in this study. LTR, long terminal repeat; Ψ, human immunodeficiency virus packaging signal; SD, 5′ splice donor site; ΔGAG, deleted group antigens; RRE, Rev responsive element; SA, 3′ splice acceptor site; cPPT, central polypurine tract; EF-1α, elongation factor 1 alpha; CO.hIL-12 (p40/p35), codon-optimized cDNA of human IL-12 β chain (p40) fused with the IL-12 α chain (p35) by a short Elastin linker; IRES, internal ribosome entry site; CO.ΔLNGFR/TMPK, codon-optimized truncated LNGFR-TMPK (F105Y) fusion; WPRE, woodchuck hepatitis virus post-transcriptional regulatory element; SIN/LTR, Self-Inactivating LTR. (b) Functional transduction efficiency of LV/IL-12 on the OCI-AML1 cell line determined by LNGFR (CD271) staining and flow cytometry. (c) Correlation of human LNGFR and IL-12 expression level in transduced cells. OCI-AML1 clones expressing different levels of LNGFR were cultured for 2 hours and the hIL-12 concentration in the supernatant was measured by ELISA. The geometric mean fluorescence intensity (Geo mean MFI) of the LNGFR signal obtained from the flow cytometry analyses was plotted against the secreted hIL-12 p70 level (ng cytokine secreted from 1 million cells in 2 hours). The data was analyzed by linear regression analysis and r2 represents the square of the correlation coefficient. LV-transduced (OCI-AML1-LV12.31) and nontransduced (NC: OCI-AML1-NC.3) OCI-AML1 clones were cultured in complete IMDM for 2 hours and the supernatant was collected. (d) Production of IFN-γ from NK-92 cells was determined by ELISA. The results are presented as the mean ± SD of triplet wells. AML1, acute myeloid leukemia1; ELISA, enzyme-linked immunosorbent assay; IFN-γ, interferon gamma; IL-12, interleukin-12; IMDM, Iscove’s Modified Dulbecco’s Medium; SD, standard deviation; LNGFR, low-affinity nerve growth factor receptor; LV, lentivector; MFI, mean fluorescence intensity; TMPK, thymidylate kinase. Full size image Figures/tables index Next We first transduced an AML cell line, OCI-AML1, with the “near-clinical grade” LV/hIL-12.ΔLNGFR/TMPK (LV/IL-12) at multiplicity of infection (MOI) of 10. An overnight infection protocol was used (see “Materials and Methods”). Cells were stained with an Alexa Fluor 647-conjugated mouse antibody against human LNGFR; the functional LV transduction efficiency was determined by flow cytometry. Positive staining for LNGFR (~54%) was observed with the transduced cells showing a range of LNGFR expression as measured by mean fluorescence intensity (Figure 1b). Single-cell clones expressing different levels of LNGFR were isolated and expanded, and the level of secreted hIL-12 (p70) protein from each clone was determined by enzyme-linked immunosorbent assay (ELISA). These cells displayed a strong positive correlation between LNGFR mean fluorescence intensity levels and hIL-12 secretion levels (Figure 1c). One of the main functions of hIL-12 is to facilitate NK cell maturation. In the presence of human IL-2, coincubation of hIL-12 with NK-92 cells (a human NK cell line) stimulates IFN-γ secretion.30 To examine the functionality of secreted hIL-12 from the LV/IL-12-transduced AML cells, we harvested supernatant from a high level hIL-12-producing clone (OCI-AML1-LV12.31) and diluted it to various concentrations. The diluted supernatant was coincubated with human IL-2 and NK-92 cells for 24 hours. In parallel, diluted supernatant from a nontransduced AML clone OCI-AML1-NC.3, or diluted commercial purified rhIL-12 was cocultured with NK-92 cells in the presence of IL-2 as well. After coculture, supernatant was collected and secreted IFN-γ was measured with Human IFN-γ ELISA MAX Deluxe kit. As shown in Figure 1d, IFN-γ levels increased accordingly with increased IL-12 concentrations. At all tested concentrations, both rhIL-12 and IL-12 from LV-transduced cells produced similar amounts of IFN-γ. Even at as low as 3.9 pg/ml of IL-12, secreted IFN-γ levels from rhIL-12 (773.1 pg/ml) and from LV-transduced cell supernatants (827.9 pg/ml) were still significantly higher than background levels from either nontransduced cells (409.1 pg/ml) or culture medium alone (393.8 pg/ml). Targeted cell-killing of LV-transduced AML cell clones by the TMPK/AZT cell-fate control system In order to test the efficacy of the cell-fate control element in this context, we treated LV-transduced AML cell clones with AZT at increasing doses (0, 20, 100, and 500 µmol/l) for up to 12 days. A nontransduced cell clone (OCI-AML1-NC.2) was tested in parallel as a negative control. At 4, 8, and 12 days post-treatment the numbers of viable and dead cells were counted. Growth and survival curves were plotted (Figure 2a,b). Nontransduced cells proliferated exponentially through the entire time course; AZT treatment had no effect on cell growth or viability. Similarly, two LV-transduced cell clones either expressing a low (OCI-AML1-LV12.35) or a high (OCI-AML1-LV12.31) level of hIL-12 showed normal growth and survival rates in the absence of added AZT. In contrast, treatment with AZT significantly reduced the growth and survival of these cells. At 20 µmol/l, AZT caused a 10-fold growth rate reduction in OCI-AML1-LV12.35 cells after 8 days of treatment. Furthermore, higher concentrations of AZT (100 and 500 µmol/l) almost completely inhibited OCI-AML1-LV12.35 cell proliferation and killed ~40–60% cells after 4 days of drug treatment. The killing effect of AZT in OCI-AML1-LV12.31cells was even more pronounced. About 20 µmol/l drug nearly stopped cell proliferation and reduced cell viability to ~50%. Higher AZT (100–500 µmol/l) concentrations fully inhibited cell growth and led to more than 90% cell death after 4 days of incubation. While the data shown are for a discreet window of time, we have previously failed to grow AZT nonresponsive variants in longer-term cultures suggesting complete elimination of transduced cells.29 These results demonstrated that LV/IL-12-transduced AML cells were selectively killed by AZT in a dose-dependent manner, and that cells expressing higher levels of the mutant TMPK fusion cassette were more sensitive. Figure 2 AZT-induced cell death in LV/IL-12-transduced OCI-AML1 clones. LV/IL-12-transduced (low level IL-12-producting clone: OCI-AML1-LV12.35 and high level IL-12-producing clone: OCI-AML1-LV12.31) and nontransduced (OCI-AML1-NC.2) clones were cultured in complete IMDM in the absence or presence of 20, 100, and 200 µmol/l AZT for up to 12 days. At 4, 8, and 12 days after treatment, cells were counted after Trypan blue staining. Viable cell numbers (a) and percentages of viable cells (b) were quantified. Data shown are mean ± SEM of triplet wells. AML, acute myeloid leukemia; AZT, 3’-azido-3’-deoxythymidine; IL-12, interleukin-12; IMDM, Iscove’s Modified Dulbecco’s Medium; LV, lentivector; SEM, standard error of the mean. Full size image Previous Figures/tables index Next Optimization of ex vivo culture conditions for maintenance of patient primary AML cells Ex vivo culture of primary AML cells in serum-free medium is achievable but challenging depending on the basal medium used, the supplemental cytokines added, and patient-to-patient variability.31 We used AIM V, a serum-free and therapeutic-grade medium suitable for ex vivo growth of human leukocytes, to culture patient peripheral blood mononuclear cells (PBMCs). To enhance cell proliferation, we supplemented the medium with human cytokines IL-3 and Stem Cell Factor (SCF). A total of 21 AML patient blood samples were obtained; PBMCs were isolated by Ficoll gradients and cryopreserved as described in Materials and Methods. Cells were then thawed and cultured in complete AIM V medium (containing 20 ng/ml hIL-3 and 100 ng/ml hSCF), and cell viability was monitored every other day. About 8 out of 21 patient samples (38%) either expanded in cell number or at least maintained the number of input cells at 6 days post-thaw (Figure 3a). Three patient samples showed more than 50% recovery after 6 days of culture, but did not reach the input cell number (Figure 3b). Lastly, 10 samples either collapsed or did not recover to half of the input cell number at 6 days post-thaw (Figure 3c). The phenotype of PBMCs from each patient was examined by staining cells with antibodies against the common leukocyte antigen CD45 and monocyte marker CD14 followed by flow cytometry analyses. Most samples consisted of two or three distinct leukocyte populations (CD45+): a major population of AML blasts (39.2–93.7%), a small population of lymphocytes (0.5–11.5%). Some samples had a population of monocytes (0–47.9%) (Supplementary Figure S1). One sample (#130472) contained 62.9% granulocytes and only 6.9% blasts. Another sample (#130284) had 46.5% monocytes and only 13.4% blasts. These latter two samples did not grow well in our ex vivo culture conditions (Figure 3b,c). For all samples, after 5 days of culture in complete AIM V medium the populations of monocytes and lymphocytes decreased and the majority remaining/proliferated cells were AML blasts (Supplementary Figure S2a). Figure 3 In vitro growth of primary AML cells. PBMCs were isolated from AML patient blood and cryopreserved as described in Materials and Methods. 2 × 106 frozen cells were thawed and cultured in complete AIM V for up to 10 days. Viable cell numbers were quantified at the time of thawing and every 2 days post-thaw. Depending on their growth profiles, samples were grouped into three categories: (a) proliferated or at least maintained the number of input cells at 6 days post-thaw; (b) did not expand or maintain original cell numbers, but slowly recovered; (c) completely collapsed after 6 days of thaw. AML, acute myeloid leukemia; PBMC, peripheral blood mononuclear cells. Full size image Previous Figures/tables index Next In order to try and improve cell survival, we supplemented the medium with different sera or KnockOut serum replacement, or grew cells in flat or round-bottom culture plates. However, none of above conditions significantly improved the survival outcome of those “poor growers” 2 days post-thaw, or was able to bring the cell number back to input after 6 days of culture (Supplementary Figures S3 and S4). Therefore, we decided to use AIM V medium supplemented with 20 ng/ml hIL-3 and 100 ng/ml hSCF for the rest of the study. Efficient transduction of primary AML cells with a “near-clinical grade” LV prep Currently, many gene therapy protocols are hampered by the limited amounts of clinical-grade lentivirus that can be produced or qualified. To achieve high transduction efficiency with a minimal number of input viral particles, we first tested different MOIs (0, 2, 5, 10, 20 and 50) for viral transduction with the “near-clinical grade” LV we obtained by contract from the IUVPF. Readouts were of a functional nature—as we measured LNGFR expression on the transduced cells. Transduction efficiencies reached a plateau at MOI of 10 and did not significantly increase at an estimated MOI of 20 or even 50 (Figure 4a). Next, we tested whether two rounds of infections could increase transduction efficiency. Patient cell samples were infected once or twice with LV/hIL-12 at an MOI of 10. The percentage of LNGFR-positive cells was quantified and only a small increase (from 32.6 ± 2.8% to 38.7 ± 0.3%) was observed following two rounds of infection (Figure 4b). Thus one infection with an MOI of 10 was used for the rest of this study. As shown in Figure 4c, 17 patient samples were transduced an MOI of 10 and an average of ~40% cells were found to be expressing LNGFR. We further examined VCN by quantitative polymerase chain reaction (qPCR) in bulk-transduced cells from four samples and found an average of 0.29 VCN/genome (Figure 4d). LV transduction did not change the phenotype in a marked fashion since the major populations of cells were still AML blasts 3 days after infection, and a portion of these cells were LNGFR-positive (Supplementary Figure S2b). Figure 4 LV transduction efficiency and VCN in primary AML cell samples. Differential transduction conditions were tested. Two days after thaw AML cells were transduced with LV/IL-12 either (a) at different MOIs (0, 2, 5, 10, 20 and 50), or (b) with 1 or 2 rounds of infections (as described in “Materials and Methods”). The percentage of LNGFR+ cells was determined by flow cytometry. Seventeen primary AML cell samples were mock-transduced or transduced once at MOI 10. The percentage of cells expressing LNGFR is shown in (c). Data shown are mean ± SEM. (d) The average VCN/genome of above bulk-transduced cells was determined by real-time PCR (as described in “Materials and Methods”). AML, acute myeloid leukemia; AZT, 3′-azido-3′-deoxythymidine; IL-12, interleukin-12; LNGFR, low-affinity nerve growth factor receptor; LV, lentivector; MOI, multiplicity of infection; PCR, polymerase chain reaction; SEM, standard error of the mean; VCN, viral copy number. Full size image Previous Figures/tables index Next To determine levels of secreted IL-12 from the transduced primary AML cells, LNGFR-positive cells were enriched for by magnetic-activated cell sorting using anti-LNGFR-coated magnetic beads and cultured in complete medium. Secreted hIL-12 in the medium was measured; the IL-12 p70 level ranged from 0.05 ng to 16.06 ng/106 cells/2hr from 13 tested samples (Figure 5). Figure 5 IL-12 production from LV/IL-12-transduced primary AML cells. Three days after LV transduction, cells were washed and incubated in complete AIM V medium for 2 hours. Supernatant was collected and hIL-12 p70 protein levels were measured by ELISA. Results shown are mean ± SEM. AML, acute myeloid leukemia; ELISA, enzyme-linked immunosorbent assay; IL-12, interleukin-12; LV, lentivector; SEM, standard error of the mean. Full size image Previous Figures/tables index Next LV integration profile and genotoxicity analyses In order to assess the potential genotoxicity of our clinically-directed LV/IL-12 construct we performed an in vitro immortalization assay in collaboration with investigators at the Hannover Medical School. This assay examines the ability of LV preps to cause a cell growth advantage by insertional mutagenesis. Primary murine lineage marker negative (Lin−) bone marrow cells were transduced with different vectors including our LV/IL-12, control LV/RRL.PPT.SF.eGFP.pre* (lv-SF),32 which is a self-inactivating (SIN)-configuration with the eGFP transgene expression under the control of the strong internal retroviral enhancer/promoter from the spleen focus-forming virus, and a positive control γ-retroviral vector pRSF91.GFPgPRE (RSF91). Since higher integrated VCNs in transduced cells may have a higher potential to cause insertional mutagenesis, we carried out two rounds of transductions using a range of MOIs to obtain a VCN between 2 and 10 (Supplementary Table S1). Transduced cells were expanded and cultured for 15 days, then seeded at 100 cells/well in 96-well plates after limiting dilution. Following two more weeks of clonal expansion, each well was screened for cell outgrowth by microscopy and 3-(4,5-Dimethylthiazol-2-yl)-2,5-diphenyltetrazolium bromide (MTT) assay. MTT, a yellow tetrazolium dye, is reduced to purple formazan in living cells.33 The colorimetric measurement of the formazan directly correlates to the number of cells in the well and represents an indirect measure of cell proliferation. The number of wells that were above the MTT background threshold was scored and used to calculate the replating frequency (RF) (see “Materials and Methods”). As shown in Figure 6a, the RF per VCN from mock-transduced cells was negative, suggesting no clonal expansion occurred. In contrast, an average of 0.002 RF/VCN was obtained from the positive-control RSF91-transduced cells, whereas negative-control lv-SF-transduced cells showed a more than 10-fold lower RF/VCN. More importantly, cells infected with our clinically-directed LV/IL-12 only displayed <0.00001 RF/VCN, which was more than 200-fold lower than the positive control vector RSF91. It is worth further noting that for the RSF91 vector, 99 wells (out of 576) showed high proliferation with a pH-change in the medium, whereas for IL-12 vector only two wells (out of 864) showed this kind of high proliferation. These results demonstrate that the induction of cell immortalization by LV/IL-12 integration is much lower than RSF91. Figure 6 LV/IL-12 integration profile and genotoxicity analysis. Mouse lineage marker negative (Lin−) BM cells were transduced with LV/IL-12, or two control vectors: γ-retroviral vector RSF91 and lv-SF. Cell growth in each well was screened and scored (as described in “Materials and Methods”). (a) The number of wells that showed clonal expansion was used to calculate the replating frequency (RF); the RF per VCN was determined for each vector. The mean of RF/VCN is indicated by a bar. If no clonal outgrowth from any well in one plate was observed, the assay was scored as negative. Additional values (dots in light gray) of assays from previous results that used the same conditions following the same SOPs was included here to allow for statistical analysis. (b) The two clones from LV/IL-12-transduced cells that showed obvious outgrowth were processed to genomic DNA extraction and LAM-PCR analysis (as described in “Materials and Methods”). PCR products were run on a 2% agarose gel (Lane 1 and 6: 1 kb DNA ladder; 2: empty; 3 and 4: IL-12; 5: Mock). The DNA bands were excised and sequenced. Green numbers indicate that the sequence got a definitive BLAST hit in the murine genome, whereas red numbers indicated to the sequence did not give an unambiguous BLAST result from the murine genome. BM, bone marrow; IL-12, interleukin-12; LV, lentivector; LAM-PCR, linear amplification-mediated PCR; PCR, polymerase chain reaction. Full size image Previous Figures/tables index Next The two wells containing highly-proliferating LV/IL-12-transduced cells were expanded and genomic DNA was extracted for linear amplification-mediated PCR analysis. This method uses primers directed to the LTR-region of the vector and extends the sequence into the flanking genomic host DNA.34 The resulting amplicons were visualized on 2% agarose gels. Since the mean VCN in the LV/IL-12-transduced cells was 6–7 (Supplementary Table S1), proviral insertion must have occurred into multiple sites within one cell in at least a portion of the transduced population. Hence, multiple bands should be observed on the gel. As shown in Figure 6b, DNA from mock-transduced cells yielded a nonspecific background smear. DNA from the two LV/IL-12-transduced clones generated multiple bands on the gel, however. These bands were excised from the gel, purified, and sequenced. The bands with red numbers had a low sequencing quality, with no clear match to the mouse genome or were the amplified internal control bands from the vector. The green numbers indicated a successful BLAST hit from analysis against the murine genome. The sequencing results are listed in Table 1. Table 1: LV insertion site sequencing results Full table Figures/tables index In clone #2 (lane 4, sequence 4) we found an insertion inside contactin-associated protein-like 2 (Cntnap2). A similar integration has been found as a prominent insertion site in a deep sequencing study (our unpublished data). However, the insertion did decrease over time in mice and did not lead to any kind of leukemia (our unpublished data). Cntnap2 is involved in positioning of potassium channels in murine central nervous system, but Cntnap2 mutant mice show no overt phenotype.35 Another insertion from clone #2 (lane 4, sequence 5) was identified to be downstream of Gm15091, which has an unknown gene function. In clone #1 (lane 3, sequence 8) from the same plate, an integration was found inside the gene encoding methionine adenosyltransferase 2 subunit beta, for which there has not been any direct link to any hematologic malignancy established to date. We also examined cell growth after transduction over the first 15 days of the assay. There was no significant difference in cell number over time for all tested vectors versus mock-transduced cells (Supplementary Figure S5), suggesting that the vector preparation/transduction regimen had no toxic effect on the target cells. Validation of scale-up LV-transduction and cell production under GMP conditions Two AML samples previously cryopreserved in our laboratory and tested to be “good growers” were selected for the scale-up transduction study. Cells were thawed, cultured, and transduced at an MOI = 0 or 10 with a research-grade LV/IL-12 produced in our laboratory. Cells were subsequently washed and expanded for two more days. As shown in Supplementary Table S2, transduction frequencies of these two samples were 18.0 and 33.6%, respectively, as determined by the percentage of LNGFR-positive cells. An average of 1.4 and 1.2 VCN per genome from each sample, respectively, was achieved. Secreted hIL-12 levels from the final cell product were determined to be 24.0 and 92.3 ng/2hr/106 cells. Next we conducted a scale-up validation experiment at the Philip S. Orsino Cell Therapy Facility at the UHN in compliance with Good Manufacturing Practices (GMP). AML patient blood samples were collected, processed, and cryopreserved according to corresponding SOPs. One vial of cryopreserved PBMCs (2.0 × 108) from patient (Unique number: 151258) was thawed (Day 1) and cultured for 2 days in GMP-grade complete AIM V medium for the GMP validation study. Viable cells (63.75%) were recovered after isolation from Ficoll-Paque gradient (Figure 7a). Live cells (1 × 107) were subjected to an overnight LV transduction (see “Materials and Methods”). The next morning (Day 4), cells were washed and cultured in fresh medium for two more days. On day 6, 74% of input cells were recovered from the time of transduction initiation (Figure 7a). The final cell product was obtained by washing and resuspending cells with infusion buffer (Plasma-Lyte A plus 0.5% human serum). Figure 7 GMP scale-up production of LV-transduced primary AML cells. (a) Cryopreserved (under GMP) patient PBMCs were thawed and cultured for 2 days in a Cell Therapy Facility. Live cells were isolated and transduced with LV/IL-12 at MOI = 10. (b) Three days after transduction, cells were washed and resuspended in infusion buffer (Plasma Lyte A plus 0.5% human serum). An aliquot of cells were analyzed by flow cytometry to determine transduction efficiency by measure human LNGFR staining. (c) The stability of the cell product was tested at indicated time post-harvest and represented as total viable cells or % viable cells. AML, acute myeloid leukemia; GMP, good manufacturing practices; IL-12, interleukin-12; LV, lentivector; LNGFR, low-affinity nerve growth factor receptor; MOI, multiplicity of infection; PBMCs, peripheral blood mononuclear cells. Full size image Previous Figures/tables index The recipe of the above infusion buffer was developed from two small-scale transduction experiments in which we tested the stability of transduced cell product in different buffer conditions. As shown in Supplementary Figure S6, more than 50% of cells were lost after being washed and resuspended in Plasma-Lyte A without human serum. Those cells that were recovered only showed ~50% viability. Supplementing Plasma-Lyte A with small amount of human serum significantly improved cell recovery and viability to more than 80%. In the presence of 0.5% human serum, cell recovery and viability reached more than 90% even after overnight storage and was the best condition among tested concentrations. Therefore, we chose Plasma-Lyte A containing 0.5% human serum as the final infusion buffer. Aliquots of the final product were sampled for standard sterility testing and subjected to flow cytometry analyses to determine transduction efficiency. LNGFR-positive staining was observed in 16.6% of the cells in the final product (Figure 7b). The stability of the final cell product at room temperature was tested by counting cell viability at each hour time point after product completion. Fidelity was preserved over 24 hours (Figure 7c) with >95% of cells remaining viable. Discussion Abstract • Introduction • Results • Discussion • Materials and Methods • References • Acknowledgements • Author information • Supplementary information Clinical trial studies have demonstrated intolerable toxicities in patients when IL-12 is systemically administered and the dose-schedule is nonoptimal. Evaluating alternative delivery routes to avoid systemic toxicity is a focus of current studies of IL-12-based therapy. Localized delivery of the IL-12 cDNA in several phase 1/2 trials has showed promising results. In the first, intraperitoneal injection of an hIL-12 plasmid formulated with a synthetic polyethyleneglycol-polyethyleneimine-cholesterol lipopolymer into ovarian cancer patients achieved encouraging outcomes (a response of 31% stable disease and 69% progressive disease), and was well-tolerated.17 In a second study, direct intratumoral injection of an IL-12 plasmid, accompanied with electroporation, to treat metastatic melanoma demonstrated no adverse effects related to IL-12 and anticancer responses were observed in 53% of patients.19 Intratumoral injection of adenoviral vector that locally expresses IL-12 in advanced digestive tumors (stablizing disease in 29% patients) did not reach dose-limiting toxicity.20 A recent phase 1 clinical trial using autologous tumor-infiltrating lymphocytes engineered by γ-retrovirus infection to express hIL-12 to treat metastatic melanoma showed objective responses in 69% of patients.23 The above results notwithstanding, administration of plasmids or adenoviral vectors gives short-term production of IL-12 and repeated injections are often required. Gammaretroviral vectors have higher risk causing oncogenesis by insertional mutagenesis.36,37 Lentivirus vectors have been proven to be safe and give long-term efficacy in gene therapy. They can infect a variety of primary human cells with a high efficiency, and they integrate more randomly into the genome than gammaretroviruses. Clinical trials using LVs to deliver functional cDNAs into hematopoietic stem cells for treating genetic diseases and to deliver chimeric antigen receptors into T cells to treat B cell lymphomas have been successful.38,39,40,41,42 Currently more than 140 LV-based clinical trials are open for cancer and inherited diseases (Gene Therapy Trials Worldwide, in www.wiley.com/legacy/wileychi/genmed/clinical/). We evaluated the feasibility of using a clinically-directed recombinant LV to deliver hIL-12 into primary AML cells in this report in support of our CTA. We designed a novel recombinant LV to express hIL-12 in ex vivo-cultured patient AML cells. We aim to infuse the modified AML cells back into the patient so that IL-12 is secreted at high levels at localized immunological synapses and induces a systemic immune response against the leukemia. We have noticed that ~30% of tested primary AML samples (21 here and >50 independent samples since) showed good growth throughout 6–10 day culture, therefore we will test the growth of each patient’s cryopreserved PBMCs ahead of time and only those having adequate number of live cells will be eligible for the trial. This requirement now forms part of our inclusion/exclusion criteria. In our phase 1 clinical trial protocol, cryopreserved patient PBMCs will be thawed and cultured for 2 days, transduced overnight with LV/IL-12, and expanded for two more days. Cell product will be harvested on day 6 and subsequently infused back to the patient. We have shown in this report that the cell product contains proviral integrations and expresses functional IL-12. From our mouse data, we expect these transduced cells will stably produce IL-12 in the AML patient’s blood once they are reinfused. As mentioned above, a major concern in IL-12-based clinical trials is the unacceptable toxic effects associated with high systemic doses of IL-12. Tumor-infiltrating lymphocytes engineered by gammaretroviral vector infection to express hIL-12 for immunotherapy of melanoma led to clinical responses in 63% patients at doses of 0.3–3 × 109 cells, however the responses were short and higher cell doses induced toxic effects such as high fever, liver dysfunction, and hemodynamic instability.23 One patient developed life-threatening hemodynamic abnormalities and had to be treated with anti-IL-12 antibody and drugs to eliminate lymphocytes. The LV we designed harbors a cell-fate control (aka “suicide”) cassette, ΔLNGFR/TMPK, as a safety control mechanism. In this construct, the safety control cDNA fragment is downstream of the IRES following the hIL-12 fusion cDNA. This arrangement allows a single mRNA transcript to contain both the IL-12 fusion and ΔLNGFR/TMPK factor to help ensure synchronized expression. The mutant TMPK we utilize can initiate catalysis of AZT-MP into downstream toxic forms, which can induce cell death.28 Therefore, LV-transduced cells can be selectively eradicated by treatment with AZT. The cell-fate control element is a safety system in this context in case infected cells show any tumorigenic growth from viral insertion or induce severe toxicity due to overexpression of IL-12 and need to be eliminated. Indeed, we showed here that LV-transduced AML cell lines were sensitive to AZT. Moreover, the cells targeted in our protocol by the LV transduction to produce IL-12 are patient leukemia cells, which eventually will be killed should an antitumor response is initiated. This will avoid extensive expansion of IL-12-producing cells and should also limit potential toxicity caused by high IL-12 levels. We have tested different medium and culture conditions to grow primary AML cells in this study. Under the most optimal ex vivo culture conditions, 8 out of 21 patient samples expanded or maintained the number of input cells 6 days after thaw. We successfully transduced these cells with a “near-clinical grade” LV manufactured in a GMP facility. Our previous study in an ALL mouse model showed that only a small proportion of LV-transduced cells were required for tumor rejection once the IL-12 expression level exceeded a certain threshold.26 Although, we are unaware of the effective threshold for human AML, the LV-transduced cells in this study were heterogeneous in expression level and a high producing population was detected. Our results suggest that it is feasible to use ex vivo cultured patient AML cells engineered to express hIL-12 from a recombinant LV infection to treat AML patients for a phase 1 clinical study. Materials and Methods Abstract • Introduction • Results • Discussion • Materials and Methods • References • Acknowledgements • Author information • Supplementary information Reagents Alexa Fluor 647-conjugated mouse antihuman CD271 (LNGFR) antibody, clone: C40-1457 (BD Biosciences, Franklin Lakes, NJ), FITC-anti-human CD45 (Biolegend, San Diego, CA) and PE-anti-human CD14 (BD Biosciences) antibodies, and 7-AAD (BD Biosciences) were used for flow cytometry. Recombinant human IL-12 (p70), hIL-2, 7-Aminoactinomycin D (7-AAD) and Trypan blue stain (0.4%) were purchased from BioLegend, R&D Systems (Minneapolis, MN), BD Biosciences, and Gibco by Life Technologies (Carlsbad, CA), respectively. Human AB serum (Invitrogen, Carlsbad, CA), fetal bovine serum (FBS; Life Technologies), horse serum (Invitrogen) and KnockOut Serum (Life Technologies) were used for cell culture. Other reagents include MTT: (Sigma-Aldrich, St. Louis, MO), AZT: (ViiV Healthcare, Brentford, Greater London, UK), and protamine sulfate (Sigma-Aldrich). Cell culture Cell lines. OCI-AML1 cells were cultured in Roswell Park Memorial Institute medium (RPMI) 1640 (Sigma-Aldrich) or Iscove’s Modified Dulbecco’s Medium (IMDM, Invitrogen) supplemented with 10% FBS, 2 mmol/l glutamine, 100 U/ml penicillin, and 100 mg/ml streptomycin. Human embryonic kidney (HEK) 293T cells were cultured in Dulbecco’s Modified Eagle’s Media (DMEM) (Gibco by Life Technologies) supplemented with 10% FBS and 1×PSQ (2 mmol/l glutamine, 100 U/ml penicillin, and 100 mg/ml streptomycin, Gibco by Life Technologies). NK-92 cell line was purchased from ATCC (Manassas, VA) and cells were cultured in α-MEM (Gibco by Life Technologies) supplemented with 0.2 mmol/l myo-inositol (Sigma-Aldrich), 0.02 mmol/l folic acid (Sigma-Aldrich), 0.1 mmol/l 2-mercaptoethanol (Thermo Fisher Scientific, Waltham, MA), 12.5% horse serum (Invitrogen), 12.5% fetal bovine serum (Gibco, Life Technologies) and 2 ng/ml hIL-2. Human primary AML cells were cultured in AIM V medium (research and clinical grade, Life technologies) supplemented with 20 ng/ml recombinant human IL-3 (R&D Systems) and 100 ng/ml recombinant human SCF (R&D Systems). All cells were maintained in a humidified incubator at 37°C with 5% CO2. Isolation and cryopreservation of patient PBMCs All studies involving patient samples were approved by the UHN Research Ethics Board. Blood samples were drawn from patients that had been guided through the informed consent process by qualified personnel in the Princess Margaret Cancer Centre at UHN. 5 ml anticoagulant-treated blood was collected, mixed with 5 ml Plasma-Lyte A (Baxter, Deerfield, IL), and loaded on top of 10 ml Ficoll-Paque Premium (GE, Schenectady, NY) in a 50 ml conical centrifuge tube. After centrifugation at 400g for 30 minutes at 18°C, the layer of mononuclear cells at the interface was transferred to a 15 ml conical centrifuge tube. Three volumes of Plasma-Lyte A was added onto the cells and mixed by pipetting. The resulting cell suspension was then centrifuged at 200g for 5 minutes at room temperature. The pellet containing mononuclear cells was resuspended in Human Serum Plus (GEMINI, West Sacramento, CA) to a density of 2 × 108 cells/ml. The range of cell numbers collected was 3 × 107–1 × 109 cells from 5 ml peripheral blood. Equal volumes of freshly prepared 80% Human Serum Plus plus 20% DMSO (Cryoserv, Bioniche PHARMA, Lake Forest, IL) was subsequently added onto the cell suspension dropwise to achieve a final cell density of 1 × 108 cells/ml. Cells were then aliquoted into cryovials and transferred into a freezing container. Cells were stored at −80°C overnight and transferred to vapor-phase nitrogen for long-term storage. LV construction A DNA cassette comprising a Kozak consensus sequence, the codon-optimized cDNA of human IL-12 β chain (p40) fused to the α chain (p35) by an Elastin linker (VPGVGVPGVG), the IRES sequence, and the CD19ΔTmpkF105Y fusion cDNA29 was synthesized by Genscript (Piscataway, NJ) and subcloned into the 3′SIN, HIV-1-based, lentiviral backbone pDY.cPPT-EF1α.WPRE previously generated in our laboratory,29 between the EcoRI and BamHI restriction enzyme sites. The codon-optimized cDNA of human ΔLNGFR/TMPK was amplified from a previously described vector pDY.LNGFRΔTmpk,29 and flanked by XbaI and BamHI restriction enzyme sites. Then the CD19ΔTmpkF105Y fragment was swapped out with the ΔLNGFR/TMPK fragment between the XbaI and BamHI sites. The resulting vector was denoted as pDY.hIL-12.IRES. ΔLNGFR/TMPK and verified by restriction enzyme digestion and DNA sequencing. DNA sequencing revealed two mutations in the plasmid sequence which are not known polymorphisms. One is a Cysteine to Glycine change at the second amino acid in p40 subunit, which is within the signal sequence of IL-12. The other one is a Leucine to Serine change at the 165 amino acid in p35 subunit. This is a very conservative substitution and is not likely projected to be in the cytokine/receptor interface.43 No other sequence alterations were found. Purification of research and “near-clinical grade” LV and functional titer analyses Research-grade LV/IL-12 was produced in our laboratory at UHN as described previously.44 The “near-clinical grade” LV/IL-12 used in this study was produced at the Indiana University Vector Production Facility (IUVPF, Indianapolis, IN) in compliance with Good Manufacturing Practices (GMP) for potential human clinical trial use under an Investigational New Drug (IND) submission. The LV particles are produced using HEK293T packaging cells from a certified Master Working Cell Bank maintained by the IUVPF. The packaging cells were expanded to 4 l culture volume and transiently cotransfected by the LV packaging plasmids (pCMVΔR8.91 and pMDG) and transfer plasmid (pDY.hIL-12.IRES. ΔLNGFR/TMPK) which are provided by our laboratory. LV particles were harvested twice, yielding a total 8 l of unconcentrated LV-containing supernatant. The LV-containing supernatant was purified by Mustang Q ion-exchange chromatography, concentrated by tangential flow filtration, and buffer-exchanged into ~100 ml clinical-grade CTS AIM V Medium. Aliquots of the final vector product were retained by the IUVPF for quality control analyses, including confirmation of the vector identity by Southern blot analysis, titer by p24 ELISA, and testing for aerobic and anaerobic sterility, mycoplasma levels, endotoxin levels, residual DNA levels, and residual benzonase levels. Our laboratory at UHN performed functional titer testing of all LV vector preps by transduction of HEK293T cells using serial dilutions of the vector followed by analysis of LNGFR expression on the surface of transduced cells by flow cytometry. LV transductions Frozen AML cells were thawed and cultured in complete AIM V medium for 48 hours at a density of 8 × 106 cells/ml. Every 10 ml of cell suspension was transferred into separate 15 ml conical tubes and 2 ml Ficoll-Paque Premium was carefully added at the bottom of the cell suspension. After centrifugation at 400g for 10 minutes at 18°C, the layer of live cells was drawn out by a pipette and transferred into a 15 ml centrifuge tube. Then three volumes of AIM V medium was added in and mixed with the cells. The resulting cell suspension was centrifuged at 200g for 5 minutes at room temperature and the cell pellet was resuspended in 10 ml complete AIM V. Cell count by Trypan blue exclusion was performed to determine live cell number, and then cells were pelleted by centrifugation at 200g for 5 minutes at room temperature. The cell pellet was resuspended with desired volume of LV to reach desired MOIs, and supplemented with complete AIM V and protamine sulfate (final concentration at 8 µg/ml) to reach a density of 1 × 106 cells/ml. During the transductions, cells were incubated overnight in a humidified incubator at 37°C with 5% CO2, then washed and resuspended in fresh culture medium the next morning. For cells receiving two rounds of transductions, the second infection was started 2 days after cells were washed following the first transduction. AZT treatment Cells derived from OCI-AML1 clones were seeded in a multiwell plate and cultured in complete IMDM in the absence or presence of 20, 100, and 200 µmol/l AZT for up to 12 days. Media and AZT were refreshed every 2 days in the cell culture. At 4, 8, and 12 days after treatment, aliquots of cells were stained with 0.4% Trypan Blue and counted to quantify total numbers and the percentages of live cells. Measurements of secreted human IL-12 p70 and analysis of IL-12 bioactivity from LV-transduced cells LV-transduced OCI-AML1 cells or primary leukemia PBMCs were washed twice and resuspended with prewarmed culture media to a density of 1 × 106 cells /ml, then incubated at 37°C with 5% CO2 for 2 hours. The cell culture supernatant was then collected and the amount of human IL-12 p70 was measured with OptEIA Human IL-12 (p70) ELISA Set (BD Biosciences) according to the manufacturer’s instructions. LV-transduced (OCI-AML1-LV12.31) and nontransduced (OCI-AML1-NC.3) OCI-AM1 clones were cultured in IMDM medium supplemented with 10% FBS for 2 hours and the supernatant was collected. The IL-12 p70 protein in the OCI-AML1-LV12.31 supernatant was measured with OptEIA Human IL-12 (p70) ELISA Set (BD Biosciences) and diluted to different concentrations with the above medium. The recombinant hIL-12 purchased from R&D Systems (Minneapolis, MN) as a positive control was diluted to the same concentrations in the same medium. To measure IFN-γ levels by ELISA, diluted supernatant from LV/IL-12-transduced clone and commercial rhIL-12 were cocultured with NK-92 cells in the presence of 2 ng/ml hIL-2 at a density of 1 × 106 cells/ml. Supernatant from the nontransduced clone was also diluted to the highest dose as done for the LV-transduced clone, and used as a negative control in parallel. After 24-hour incubation at 37°C, 5% CO2, the supernatant was collected and the secreted IFN-γ was measured with Human IFN-γ ELISA MAX Deluxe (BioLegend). Quantitative real-time PCR Three days post-LV transduction, cells were collected and washed once in phosphate buffered saline (PBS). 1 × 106 cells were lysed and genomic DNA was extracted using the Gentra Puregene Cell Kit (QIAGEN, Venlo, Limburg, Netherlands) according to the manufacturer’s instructions. A specially designed primer set (WPRE-RT-F: 5′ TCCTGGTTGCTGTCTCTTTATG 3′ and WPRE-RT-R: 5′ TGACAGGTGGTGGCAATG 3′) and a TaqMan probe (5′ FAM- TGCTGACGCAACCCCCACTGGT-TAMRA 3′), synthesized by Life Technologies were used to detect a unique LV-specific region (WPRE) of the integrated provirus. Fifty ng genomic DNA prepared from an AML cell clone containing one copy provial integration (established in our laboratory and verified by Southern Blot)45 was serially diluted with genomic DNA from a nontransduced AML cell clone to generate DNA standards. Real-time PCR was performed on these DNA standards to generate a cycle threshold (Ct) value versus VCN curve for quantification of test samples in parallel. VCN in transduced cells (copy number/genome) was determined by plotting the Ct value from transduced cells against the standard curve. Human β-actin DNA levels were detected with a TaqMan gene expression assay kit (Life Technologies, #4331182, Gene ID: Hs03023880_g1) and was used to normalize DNA levels between test samples and the standards. In vitro immortalization assays Transduction and clonal expansion of murine bone marrow cells. Mouse lineage-marker negative (Lin−) bone marrow cells were isolated and kept at −80°C to allow for the same cell quality for all assays. The next day, cells were thawed and plated at 1 × 105 cells/well in StemSpan medium supplemented with Complete Growth Medium Supplements (CGMS: 1% Penicillin/Streptomycin, 50 ng/ml mouse SCF, 100 ng/ml Flt-3L, 100 ng/ml IL-11, and 20 ng/ml mouse IL-3 (all from Peprotech, Rocky Hill, NJ) in a 24-well plate for 2 days. The viral vectors were preloaded on Retronectin (Takara Bio, OTSU-SHI, SHG, Japan)-coated wells and the prestimulated Lin− cells were transferred to the vector-loaded wells for the first transduction round. On the following day (day 0), vectors were preloaded on a new Retronectin-coated plate and the previous-day transduced cells were transferred into these second round transduction wells. At day 1, cells were transferred to 12-well plates and cultured in IMDM (Biochrom, Cambridge, UK) supplemented with CGMS. Cells were further expanded up to day 15. The cells were then seeded at 100 cells/well in 96-well plates after limiting dilution and cultured up to day 28–31. On days 1, 4, 6, 8, 11, and 15, aliquots of cells were counted by Trypan blue exclusion. Genomic DNA was isolated on day 4 and subjected to qPCR analysis to determine VCN/cell. Determination of VCN. The mean VCN in this assay was determined by quantitative real-time PCR. Samples were measured in triplicate from 100 ng of genomic DNA. Primers for the WPRE element of the vector were used to determine the amount of viral sequences, which was further normalized to a genomic reference sequence of the Ptbp2 gene.32 The serial dilution of a plasmid standard containing both sequences was measured in parallel to perform an absolute quantification. Clonal scoring and calculation of RFs. At day 28–31, each well of cells was screened by microscopy and MTT assay for clonal outgrowth. Basically, two investigators independently screened each well of a 96-well plate microscopically and scored the clonal outgrowth in four categories. Category 1 is a robust outgrowth with a visible pH-change of the medium. Category 2 is obvious outgrowth of cells. The investigators decide which of the category 1 or 2 clones will be expanded for integration site analysis. Category 3 clones show some proliferation inside the wells and may be assigned to category 2 in case of a positive result in the MTT assay. To distinguish background absorbance readings from real cell growth, a threshold value was determined. In earlier assays, four times the standard deviation of all readings adequately recapitulated the microscopic scoring and was thus used as the threshold value. In case the measured absorbance was above the threshold, the well was scored positive in the MTT readout matrix. Category 4 described wells with no cell growth, which also had to score negative in the MTT assay. The number of wells above threshold was used to calculate the RF, using the L-Calc software version 1.1 from StemCell Technologies, according to Poisson distributions. The risk of insertional mutagenesis is correlated to the VCNs per cell. To account for the different chances of the vectors to immortalize cells due to variability of infectivity, RFs were normalized to the VCN. Insertion site analysis. About 300 ng of genomic DNA were used as a template for linear amplification-mediated PCR as previously described.34 Linear amplification of the vector genome junctions was first performed and magnetic bead capture was then used prior to restriction digestion with Tsp509I. After ligating a Tsp509I-specific linker to the samples and two rounds of nested PCRs, the amplicons were visualized on a 2% agarose gel. Specific bands were cut out, purified, and directly sequenced. References Abstract • Introduction • Results • Discussion • Materials and Methods • References • Acknowledgements • Author information • Supplementary information Heufler, C, Koch, F, Stanzl, U, Topar, G, Wysocka, M, Trinchieri, G et al. (1996). Interleukin-12 is produced by dendritic cells and mediates T helper 1 development as well as interferon-gamma production by T helper 1 cells. Eur J Immunol 26: 659–668. ISI CAS PubMed Article Hsieh, CS, Macatonia, SE, Tripp, CS, Wolf, SF, O’Garra, A and Murphy, KM (1993). Development of TH1 CD4+ T cells through IL-12 produced by Listeria-induced macrophages. Science 260: 547–549. ISI CAS PubMed Article Kobayashi, M, Fitz, L, Ryan, M, Hewick, RM, Clark, SC, Chan, S et al. (1989). Identification and purification of natural killer cell stimulatory factor (NKSF), a cytokine with multiple biologic effects on human lymphocytes. J Exp Med 170: 827–845. ISI CAS PubMed Article Metzger, DW, Buchanan, JM, Collins, JT, Lester, TL, Murray, KS, Van Cleave, VH et al. (1996). Enhancement of humoral immunity by interleukin-12. Ann NY Acad Sci 795: 100–115. Article Nizzoli, G, Krietsch, J, Weick, A, Steinfelder, S, Facciotti, F, Gruarin, P et al. (2013). Human CD1c+ dendritic cells secrete high levels of IL-12 and potently prime cytotoxic T-cell responses. Blood 122: 932–942. CAS PubMed Article Stern, AS, Podlaski, FJ, Hulmes, JD, Pan, YC, Quinn, PM, Wolitzky, AG et al. (1990). Purification to homogeneity and partial characterization of cytotoxic lymphocyte maturation factor from human B-lymphoblastoid cells. Proc Natl Acad Sci USA 87: 6808–6812. CAS PubMed Article Gubler, U, Chua, AO, Schoenhaut, DS, Dwyer, CM, McComas, W, Motyka, R et al. (1991). Coexpression of two distinct genes is required to generate secreted bioactive cytotoxic lymphocyte maturation factor. Proc Natl Acad Sci USA 88: 4143–4147. ISI CAS PubMed Article Gately, MK, Desai, BB, Wolitzky, AG, Quinn, PM, Dwyer, CM, Podlaski, FJ et al. (1991). Regulation of human lymphocyte proliferation by a heterodimeric cytokine, IL-12 (cytotoxic lymphocyte maturation factor). J Immunol 147: 874–882. ISI CAS PubMed Voest, EE, Kenyon, BM, O’Reilly, MS, Truitt, G, D’Amato, RJ and Folkman, J (1995). Inhibition of angiogenesis in vivo by interleukin 12. J Natl Cancer Inst 87: 581–586. CAS PubMed Article Lasek, W, Zagożdżon, R and Jakobisiak, M (2014). Interleukin 12: still a promising candidate for tumor immunotherapy? Cancer Immunol Immunother 63: 419–435. ISI PubMed Article Leonard, JP, Sherman, ML, Fisher, GL, Buchanan, LJ, Larsen, G, Atkins, MB et al. (1997). Effects of single-dose interleukin-12 exposure on interleukin-12-associated toxicity and interferon-gamma production. Blood 90: 2541–2548. ISI CAS PubMed Atkins, MB, Robertson, MJ, Gordon, M, Lotze, MT, DeCoste, M, DuBois, JS et al. (1997). Phase 1 evaluation of intravenous recombinant human interleukin 12 in patients with advanced malignancies. Clin Cancer Res 3: 409–417. ISI CAS PubMed Portielje, JE, Kruit, WH, Schuler, M, Beck, J, Lamers, CH, Stoter, G et al. (1999). Phase 1 study of subcutaneously administered recombinant human interleukin 12 in patients with advanced renal cell cancer. Clin Cancer Res 5: 3983–3989. ISI CAS PubMed Lenzi, R, Edwards, R, June, C, Seiden, MV, Garcia, ME, Rosenblum, M et al. (2007). Phase 2 study of intraperitoneal recombinant interleukin-12 (rhIL-12) in patients with peritoneal carcinomatosis (residual disease < 1 cm) associated with ovarian cancer or primary peritoneal carcinoma. J Transl Med 5: 66. CAS PubMed Article Gollob, JA, Mier, JW, Veenstra, K, McDermott, DF, Clancy, D, Clancy, M et al. (2000). Phase 1 trial of twice-weekly intravenous interleukin 12 in patients with metastatic renal cell cancer or malignant melanoma: ability to maintain IFN-gamma induction is associated with clinical response. Clin Cancer Res 6: 1678–1692. ISI CAS PubMed Barrios, B, Baez, NS, Reynolds, D, Iribarren, P, Cejas, H, Young, HA et al. (2014). Abrogation of TNFα production during cancer immunotherapy is crucial for suppressing side effects due to the systemic expression of IL-12. PLoS One 9: e90116. Article Anwer, K, Barnes, MN, Fewell, J, Lewis, DH and Alvarez, RD (2010). Phase-1 clinical trial of IL-12 plasmid/lipopolymer complexes for the treatment of recurrent ovarian cancer. Gene Ther 17: 360–369. Article Heinzerling, L, Burg, G, Dummer, R, Maier, T, Oberholzer, PA, Schultz, J et al. (2005). Intratumoral injection of DNA encoding human interleukin 12 into patients with metastatic melanoma: clinical efficacy. Hum Gene Ther 16: 35–48. ISI CAS PubMed Article Daud, AI, DeConti, RC, Andrews, S, Urbas, P, Riker, AI, Sondak, VK et al. (2008). Phase 1 trial of interleukin-12 plasmid electroporation in patients with metastatic melanoma. J Clin Oncol 26: 5896–5903. ISI CAS PubMed Article Sangro, B, Mazzolini, G, Ruiz, J, Herraiz, M, Quiroga, J, Herrero, I et al. (2004). Phase 1 trial of intratumoral injection of an adenovirus encoding interleukin-12 for advanced digestive tumors. J Clin Oncol 22: 1389–1397. ISI CAS PubMed Article Linette, G, Hamid, O, Whitman, E, Nemunaitis, J, Chesney, J, Agarwala, S, et al. (2013). A phase 1 open-label study of Ad-RTS-hIL-12, an adenoviral vector engineered to express hIL-12 under the control of an oral activator ligand, in subjects with unresectable stage III/IV melanoma. J Clin Oncol 31: abstr 3022. Mazzolini, G, Alfaro, C, Sangro, B, Feijoó, E, Ruiz, J, Benito, A et al. (2005). Intratumoral injection of dendritic cells engineered to secrete interleukin-12 by recombinant adenovirus in patients with metastatic gastrointestinal carcinomas. J Clin Oncol 23: 999–1010. ISI CAS PubMed Article Zhang, L, Morgan, RA, Beane, JD, Zheng, Z, Dudley, ME, Kassim, SH et al. (2015). Tumor-infiltrating lymphocytes genetically engineered with an inducible gene encoding interleukin-12 for the immunotherapy of metastatic melanoma. Clin Cancer Res 21: 2278–2288. CAS PubMed Article Deschler, B and Lübbert, M (2006). Acute myeloid leukemia: epidemiology and etiology. Cancer 107: 2099–2107. ISI PubMed Article Wiernik, PH (2014). Inching toward cure of acute myeloid leukemia: a summary of the progress made in the last 50 years. Med Oncol 31: 136. Article Labbe, A, Nelles, M, Walia, J, Jia, L, Furlonger, C, Nonaka, T et al. (2009). IL-12 immunotherapy of murine leukaemia: comparison of systemic versus gene modified cell therapy. J Cell Mol Med 13(8B): 1962–1976. Article Wei, LZ, Xu, Y, Nelles, EM, Furlonger, C, Wang, JC, Di Grappa, MA et al. (2013). Localized interleukin-12 delivery for immunotherapy of solid tumours. J Cell Mol Med 17: 1465–1474. PubMed Article Sato, T, Neschadim, A, Konrad, M, Fowler, DH, Lavie, A and Medin, JA (2007). Engineered human tmpk/AZT as a novel enzyme/prodrug axis for suicide gene therapy. Mol Ther 15: 962–970. ISI CAS PubMed Article Scaife, M, Pacienza, N, Au, BC, Wang, JC, Devine, S, Scheid, E et al. (2013). Engineered human Tmpk fused with truncated cell-surface markers: versatile cell-fate control safety cassettes. Gene Ther 20: 24–34. Article Hodge, DL, Martinez, A, Julias, JG, Taylor, LS and Young, HA (2002). Regulation of nuclear gamma interferon gene expression by interleukin 12 (IL-12) and IL-2 represents a novel form of posttranscriptional control. Mol Cell Biol 22: 1742–1753. ISI CAS PubMed Article Bruserud, O, Gjertsen, BT, Foss, B and Huang, TS (2001). New strategies in the treatment of acute myelogenous leukemia (AML): in vitro culture of AML cells–the present use in experimental studies and the possible importance for future therapeutic approaches. Stem Cells 19: 1–11. CAS PubMed Article Rittelmeyer, I, Rothe, M, Brugman, MH, Iken, M, Schambach, A, Manns, MP et al. (2013). Hepatic lentiviral gene transfer is associated with clonal selection, but not with tumor formation in serially transplanted rodents. Hepatol 58: 397–408. Article Mosmann, T (1983). Rapid colorimetric assay for cellular growth and survival: application to proliferation and cytotoxicity assays. J Immunol Methods 65: 55–63. ISI CAS PubMed Article Schmidt, M, Schwarzwaelder, K, Bartholomae, C, Zaoui, K, Ball, C, Pilz, I et al. (2007). High-resolution insertion-site analysis by linear amplification-mediated PCR (LAM-PCR). Nat Methods 4: 1051–1057. ISI CAS PubMed Article Poliak, S, Salomon, D, Elhanany, H, Sabanay, H, Kiernan, B, Pevny, L et al. (2003). Juxtaparanodal clustering of Shaker-like K+ channels in myelinated axons depends on Caspr2 and TAG-1. J Cell Biol 162: 1149–1160. ISI CAS PubMed Article Oldham, RA, Berinstein, EM and Medin, JA (2015). Lentiviral vectors in cancer immunotherapy. Immunotherapy 7: 271–284. PubMed Article Rothe, M, Modlich, U and Schambach, A (2013). Biosafety challenges for use of lentiviral vectors in gene therapy. Curr Gene Ther 13: 453–468. ISI CAS PubMed Article Kalos, M, Levine, BL, Porter, DL, Katz, S, Grupp, SA, Bagg, A et al. (2011). T cells with chimeric antigen receptors have potent antitumor effects and can establish memory in patients with advanced leukemia. Sci Transl Med 3: 95ra73. CAS PubMed Article Porter, DL, Levine, BL, Kalos, M, Bagg, A and June, CH (2011). Chimeric antigen receptor-modified T cells in chronic lymphoid leukemia. N Engl J Med 365: 725–733. ISI CAS PubMed Article Grupp, SA, Kalos, M, Barrett, D, Aplenc, R, Porter, DL, Rheingold, SR et al. (2013). Chimeric antigen receptor-modified T cells for acute lymphoid leukemia. N Engl J Med 368: 1509–1518. ISI CAS PubMed Article Hacein-Bey Abina, S, Gaspar, HB, Blondeau, J, Caccavelli, L, Charrier, S, Buckland, K et al. (2015). Outcomes following gene therapy in patients with severe Wiskott-Aldrich syndrome. JAMA 313: 1550–1563. PubMed Article Aiuti, A, Biasco, L, Scaramuzza, S, Ferrua, F, Cicalese, MP, Baricordi, C et al. (2013). Lentiviral hematopoietic stem cell gene therapy in patients with Wiskott-Aldrich syndrome. Science 341: 1233151. CAS PubMed Article Jones, LL and Vignali, DA (2011). Molecular interactions within the IL-6/IL-12 cytokine/receptor superfamily. Immunol Res 51: 5–14. CAS PubMed Article Wang, JC, Felizardo, TC, Au, BC, Fowler, DH, Dekaban, GA and Medin, JA (2013). Engineering lentiviral vectors for modulation of dendritic cell apoptotic pathways. Virol J 10: 240. Article Walia, JS, Neschadim, A, Lopez-Perez, O, Alayoubi, A, Fan, X, Carpentier, S et al. (2011). Autologous transplantation of lentivector/acid ceramidase-transduced hematopoietic cells in nonhuman primates. Hum Gene Ther 22: 679–687. ISI PubMed Article Download references Acknowledgements Abstract • Introduction • Results • Discussion • Materials and Methods • References • Acknowledgements • Author information • Supplementary information This study was supported by the Krembil Foundation and the Princess Margaret Cancer Foundation. This work was done in Toronto, Canada and Hannover, Germany. Author information Abstract • Introduction • Results • Discussion • Materials and Methods • References • Acknowledgements • Author information • Supplementary information Author notes Current address: Medical College of Wisconsin, Milwaukee, Wisconsin, USA Jeffrey A Medin Affiliations University Health Network, Toronto, Ontario, Canada Ju Huang , Yuanfeng Liu , Bryan C Au , Dwayne L Barber , Andrea Arruda , Mark D Minden , Christopher J Paige & Jeffrey A Medin Department of Laboratory Medicine & Pathobiology, University of Toronto, Toronto, Ontario, Canada Dwayne L Barber Department of Medical Biophysics, University of Toronto, Toronto, Ontario, Canada Dwayne L Barber , Mark D Minden , Christopher J Paige & Jeffrey A Medin Institute of Experimental Hematology, Hannover Medical School, Hannover, Germany Axel Schambach & Michael Rothe Division of Hematology/Oncology, Boston Children’s Hospital, Harvard Medical School, Boston, Massachusetts, USA Axel Schambach Department of Immunology, University of Toronto, Toronto, Ontario, Canada Christopher J Paige Institute of Medical Science, University of Toronto, Toronto, Ontario, Canada Mark D Minden & Jeffrey A Medin Authors Search for Ju Huang in: NPG journals • PubMed • Google Scholar Search for Yuanfeng Liu in: NPG journals • PubMed • Google Scholar Search for Bryan C Au in: NPG journals • PubMed • Google Scholar Search for Dwayne L Barber in: NPG journals • PubMed • Google Scholar Search for Andrea Arruda in: NPG journals • PubMed • Google Scholar Search for Axel Schambach in: NPG journals • PubMed • Google Scholar Search for Michael Rothe in: NPG journals • PubMed • Google Scholar Search for Mark D Minden in: NPG journals • PubMed • Google Scholar Search for Christopher J Paige in: NPG journals • PubMed • Google Scholar Search for Jeffrey A Medin in: NPG journals • PubMed • Google Scholar Competing interests C.J. P. and J.A.M. are Academic Founders and Shareholders in AVROBIO Inc. The other authors declared no conflict of interest. Corresponding authors Correspondence to Christopher J Paige or Jeffrey A Medin. Supplementary information Abstract • Introduction • Results • Discussion • Materials and Methods • References • Acknowledgements • Author information • Supplementary information Word documents Supplementary Tables Supplementary Tables PDF files Supplementary Figures
更多查看译文
关键词
research techniques,basic,clinical,cell,gene,therapy,genome editing,cell reprogramming,pluripotent cells,vector delivery,biomaterials,nanoparticles,regenerative medicine,biodistribution
AI 理解论文
溯源树
样例
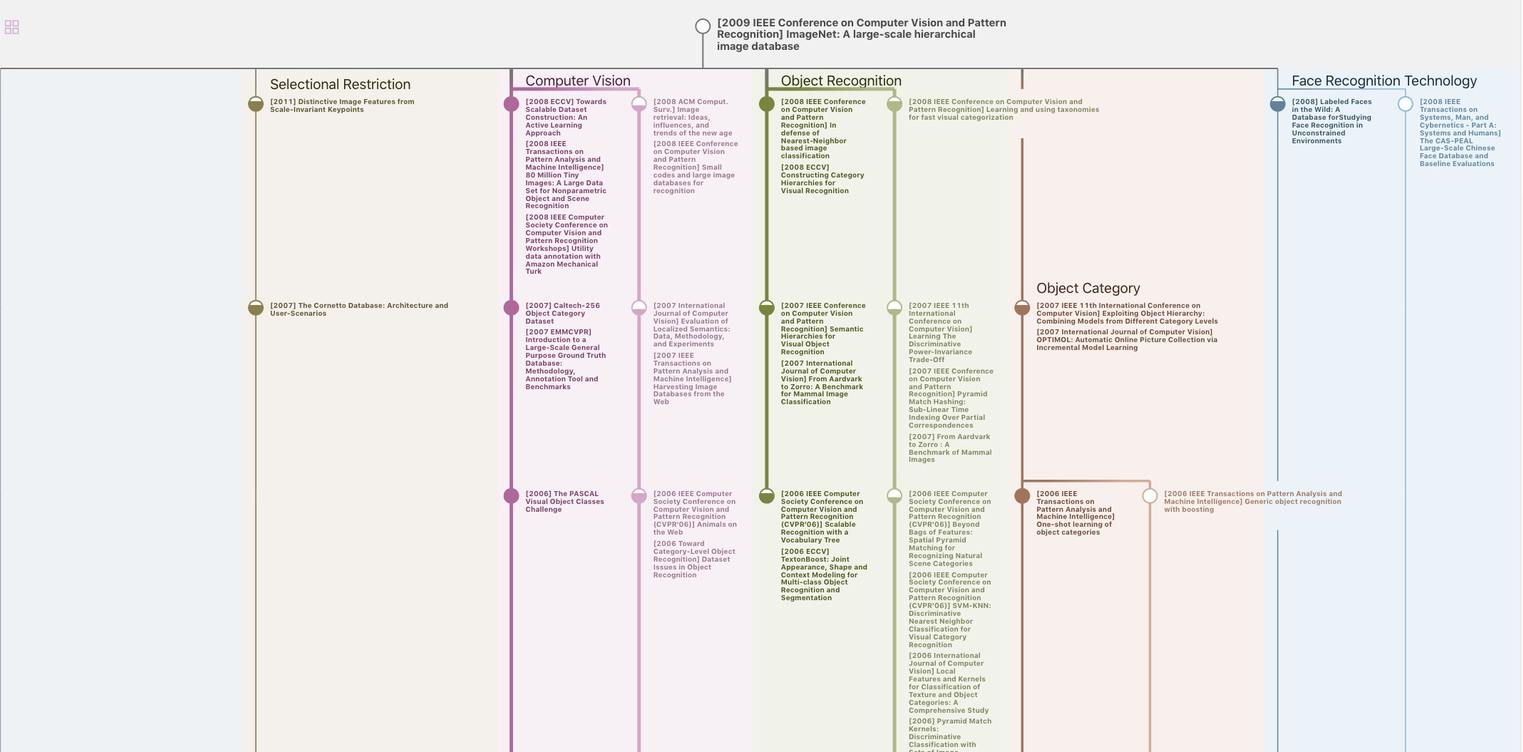
生成溯源树,研究论文发展脉络
Chat Paper
正在生成论文摘要