Groundwater chemistry near an impoundment for produced water, Powder River Basin, Wyoming, USA
Journal of Hydrology(2011)
摘要
The Powder River Basin is one of the largest producers of coal-bed natural gas (CBNG) in the United States. An important environmental concern in the Basin is the fate of the large amounts of groundwater extracted during CBNG production. Most of this produced water is disposed of in unlined surface impoundments. A 6-year study of groundwater flow and water chemistry at one impoundment, Skewed Reservoir, has produced the most detailed data set for any impoundment in the Basin. Data were collected from a network of 21 observation wells and three suction lysimeters. A groundwater mound formed atop bedrock within initially unsaturated, unconsolidated deposits underlying the reservoir. Heterogeneity in physical and chemical properties of sediments resulted in complex groundwater flow paths and highly variable groundwater chemistry. Sulfate, bicarbonate, sodium, and magnesium were the dominant ions in all areas, but substantial variability existed in relative concentrations; pH varied from less than 3 to more than 9, and total dissolved solids concentrations ranged from less than 5000 to greater than 100,000 mg/L. Selenium was a useful tracer of reservoir water; selenium concentrations exceeded 300 μg/L in samples obtained from 18 of the 24 sampling points. Groundwater travel time from the reservoir to a nearby alluvial aquifer (a linear distance of 177 m) was calculated at 474 days on the basis of selenium concentrations. The produced water is not the primary source of solutes in the groundwater. Naturally occurring salts and minerals within the unsaturated zone, dissolved and mobilized by infiltrating impoundment water, account for most of the solute mass in groundwater. Gypsum dissolution, cation-exchange, and pyrite oxidation appear to be important reactions. The complex geochemistry and groundwater flow paths at the study site underscore the difficulty in assessing effects of surface impoundments on water resources within the Powder River Basin. Keywords Produced water Coal-bed natural gas Coal-bed methane Selenium Impoundment Powder River Basin 1 Introduction The Powder River Structural Basin covers an area of more than 61,000 km 2 in northern Wyoming and southern Montana ( Fig. 1 ). The Basin is an important source of energy resources, containing the largest coal resources of any coal field in the contiguous United States ( Glass, 1997 ) and substantial oil and natural gas resources. Recent technological advances have enabled economical development of natural gas stored in coal-beds. Development of coal-bed natural gas (CBNG) has increased rapidly in the Basin since the late 1990s. Between 2000 and 2010, more than 59,000 permits for CBNG wells were approved in the Wyoming part of the Basin [ Wyoming Oil and Gas Conservation Commission , http://wogcc.state.wy.us ; accessed January 29, 2011], numbers consistent with projections of environmental impact statements ( US Bureau of Land Management (2003a,b) ). Coal-beds in the Fort Union Formation at depths of 100–1000 m are the target of most CBNG development in the Powder River Basin. Overlying the Fort Union Formation in much of the Basin are shales, siltstones, and sandstones of the Wasatch Formation. Quaternary-age unconsolidated sediments overlie the Wasatch Formation in some areas. Natural gas is captured by withdrawing water from the coal-beds, which lowers the water pressure and releases the gas. Details on CBNG generation, reserves, and development within the Powder River Basin can be found in De Bruin et al. (2000), Bartos and Ogle (2002) , and Rice et al. (2002) . Disposal of water extracted with CBNG development (referred to as produced water) is an important concern in the Powder River Basin because of the quantities and quality of water produced. New wells in the Basin produce about 47 m 3 of water per day ( Wheaton and Brown, 2005 ), and water production declines gradually over the 7–10-year life of a well. Approximately 678 million barrels (108,000,000 m 3 ) of water were produced in the Basin in 2008 [ Wyoming Oil and Gas Conservation Commission , http://wogcc.state.wy.us ; accessed July 26, 2010). Produced water is generally a sodium-bicarbonate type with total dissolved solids (TDS) in the range of 200–4000 mg/L and sodium-adsorption ratios of 5.6–9, ( Rice et al., 2002; Bartos and Ogle, 2002; Jackson and Reddy, 2007a ). Sodium-adsorption ratio (SAR) is a measure of the concentration of sodium in water relative to the concentrations of calcium and magnesium. Water with an SAR in excess of 13 is undesirable for irrigation because soil structure can deteriorate as sodium replaces calcium and magnesium on clay particles ( Soil Survey Laboratory, 1995; Mace and Amrhein, 2001 ). Several approaches have been taken for disposing of produced water. A limited amount of direct discharge of untreated water to streams is allowed in the state of Wyoming. However, discharge of untreated water raises concerns about effects on downstream water use for irrigation, effects on aquatic habitat ( Davis et al., 2009 ), and nutrient concentrations in streams ( Harris and Smith, 2009; Smith et al., 2009 ). Disposal by reinjection of water into the subsurface has been proposed ( Ross and Zoback, 2008 ), but this requires transporting the water to a location where an appropriate geologic formation can be found. Treatments such as reverse osmosis ( Welch, 2009 ) and continuous countercurrent ion exchange ( Dennis, 2007 ) can be used to lower the SAR and TDS concentrations of produced water so that the water can be discharged to streams, but these treatments can be expensive. Some CBNG water is used for surface irrigation in the Basin ( Vance et al., 2008 ); however, successful operation usually requires treatment of the water or soil with elemental sulfur or gypsum ( Ganjegunte et al., 2008 ), and even with these treatments, increases in soil SAR can occur ( Johnston et al., 2008 ). Subsurface drip irrigation has been proposed as an alternative to surface irrigation to mitigate SAR increases in the root zone ( Engle et al., 2011 ). The final environmental impact statement for the Powder River Basin Oil and Gas Project (January 2003) cites as a preferred option the storage of CBNG water in impoundments from which water can infiltrate and evaporate ( US BLM, 2003b ). At present (2010), unlined impoundments remain the most popular option for disposal of CBNG water. The state of Wyoming has issued more than 4000 permits for impoundments in the Powder River Basin [written commun., Wyoming State Engineer’s Office , 2007]. Data on groundwater-quality in areas near impoundments in the Basin are available from a few sources. Unpublished data can be obtained from the US Bureau of Land Management (BLM) and the Wyoming Department of Environmental Quality (DEQ). BLM collects water-quality samples from networks of monitoring wells and suction lysimeters in the vicinity of eight impoundments in the Basin. DEQ maintains a database of groundwater-quality analyses for approximately 170 monitoring wells spanning 146 impoundment sites [ http://deq.state.wy.us/wqd/groundwater/downloads/CBM/Report%20August%202010.pdf ; accessed Aug. 8, 2010]. Wheaton and Brown (2005) present preliminary results of groundwater-quality monitoring at three impoundment sites. This paper describes groundwater movement and the changes in groundwater chemistry that occur as a result of infiltration from an impoundment for CBNG produced water. Findings are based on groundwater-level and groundwater-quality monitoring from a network of 21 observation wells and three suction lysimeters in the vicinity of Skewed Reservoir, Johnson County, Wyoming, from August 2003 through December 2009. Results of this study may provide insight on processes occurring at other impoundments within the Basin. A previous study at this site ( Healy et al., 2008 ) described water and sediment chemistry changes in the subsurface immediately underlying Skewed Reservoir from 2003 to 2005. Groundwater transport of solutes from the reservoir toward an alluvial aquifer for the period 2003–2009 is the subject of this paper. 2 Background The study was conducted at Skewed Reservoir ( Fig. 1 ), an impoundment constructed specifically for disposal of CBNG water. The 1-ha, unlined reservoir was constructed in a naturally occurring ephemeral drainage to Beaver Creek ( Fig. 1 ) by erecting a 6-m high dam of compacted native surficial sediments in July 2003. Produced water from 6 CBNG wells was pumped into the reservoir starting on August 16, 2003 and ending in early May 2004. In August 2005 the dam was torn down and the area reclaimed. Capacity of the reservoir was about 12,000 m 3 . Underlying the impoundment were 8 m of unconsolidated deposits on top of bedrock. The study area is typical of the semiarid grasslands of the Powder River Basin. Average temperature in Gillette, about 50 km to the northeast, is about 7 °C, and average annual precipitation is 398 mm [ Western Regional Climate Center , http://www.wrcc.dri.edu ; accessed July 19, 2010]. The unconsolidated Quaternary-age surficial sediments consist of silt and very fine to fine sand with lesser amounts of clay. In the vicinity of the reservoir, the unconsolidated sediments are primarily colluvium with some alluvium and residuum. Closer to Beaver Creek, to the south and west of well 12 ( Fig. 1 ), the sediments are primarily coarse-grained alluvium (sand and gravel). Bedrock of the Eocene-age Wasatch Formation at the site is primarily composed of shale and carbonaceous shale, mudstone, siltstone, and lesser amounts of sandstone; several thin coal-beds and “peat” zones (less than 0.5-m thick) are interbedded with these deposits. The bedrock surface is variable in terms of lateral continuity, elevation, and degree of weathering and fracturing. Along the center axis of the small valley that contained the reservoir, the bedrock surface dips towards the south southwest, eventually intersecting the Beaver Creek alluvial aquifer at a distance of about 177 m from the dam ( Figs. 1 and 2 ). Cation-exchange capacity of the geologic materials was fairly uniform (average of 24.5 meq/100 g sediment with a standard deviation of 4.4) ( Healy et al., 2008 ). Quartz is the predominant mineral, generally accounting for 30–50% of total mass. Gypsum, calcite, and dolomite are present throughout the profile, in abundances of 1–8%, and clay minerals account for about 30–35% of total mass ( Healy et al., 2008 ). Healy et al. (2008) demonstrated that water infiltrating from the reservoir flushed large quantities of chloride and nitrate from the underlying sediments to the groundwater mound and that a brine formed immediately below the reservoir soon after the reservoir began accepting water. The brine was a sodium–magnesium-sulfate type, and its occurrence was attributed to gypsum dissolution that was enhanced by calcium being removed from solution and exchanged for sodium and magnesium that were present on smectite clays. 3 Methods Prior to the reservoir going into operation, 21 50-mm-diameter observation wells were installed ( Fig. 1 ). Wells 0 and 1 were installed by using a solid-stem auger. Wells 3 and 9–18 were drilled with a rotary drill rig. The remaining wells were installed with a hollow-stem auger; sediment samples were collected through the hollow-stem for lithologic descriptions and for particle-size, mineralogical, and water-soluble ion analyses ( Healy et al., 2008; Rice et al., 2011 ). Most wells were screened at the bottom of the unconsolidated deposits ( Fig. 2 ), immediately overlying the bedrock. Descriptions of well construction and lithology and electrical conductivity and gamma borehole logs are contained in Smith et al. (2011) . Suction cup lysimeters (50-mm diameter) were installed into the sediments beneath the base of the reservoir at approximate depths of 3, 5, and 7 m. A hand auger was used to bore holes for the lysimeters. Suction lysimeters allow collection of water samples when the sediments are less than fully saturated. Only data for the 7-m lysimeter are included in this paper; data for the other lysimeters and a discussion of water transport through the unsaturated zone are contained in Healy et al. (2008) . Initially, groundwater was not encountered above bedrock, except in the Beaver Creek alluvial aquifer, south of the reservoir location. Ten wells (0, 1, 2s, 4s, 4d, 7, 8, 9, 10, and 11) were initially dry ( Figs. 1 and 2 ). Wells 13–17 were completed in the Beaver Creek alluvial aquifer ( Figs. 1 and 2 ) and produced water from the beginning. Six other wells (2d, 3, 5, 6, 12, and 18) were screened in bedrock and also produced water from the beginning ( Figs. 1 and 2 ). A confined sandstone aquifer was encountered at depths of about 33 m in well 3 and 18 m in well 18. Water samples were collected approximately monthly for the first year of the study and quarterly thereafter. Water samples from wells were obtained after measuring groundwater-levels and purging three casing volumes with either submersible pumps or bailers. Lysimeter water samples were obtained by applying suction to each lysimeter, waiting about 4 h, and then applying positive air pressure to push the water sample out of the lysimeter through a sampling tube. The first sample was discarded, the process was repeated, and the second sample was saved for analysis. Samples were analyzed for major dissolved-ion chemistry and metals using standard methods ( Rice et al., 2002; Bartos and Ogle, 2002 ). Samples obtained prior to January 2006 were analyzed in laboratories of the US Geological Survey [ http://energy.cr.usgs.gov/gg/geochemlab/ ; accessed August 8, 2010]; samples obtained later were analyzed by laboratories contracted with the US Bureau of Land Management [ http://www.energylab.com/anaserv.aspx ; accessed August 8, 2010]. Temperature, pH, specific conductance, and alkalinity were determined in the field. 4 Results 4.1 Infiltration from Skewed Reservoir The amount of water that infiltrated from the reservoir was estimated with a simple water budget. Inflow to the reservoir consisted of produced water pumped into it, precipitation falling directly on the reservoir, and surface runoff from precipitation falling on areas upslope from the reservoir. A flow meter measured the total volume of water discharged to the reservoir at approximately 95,000 m 3 , an average of 52 m 3 /day/well. Direct precipitation for the 2-year period between reservoir construction and reclamation was estimated at 7400 m 3 on the basis of precipitation measured at the National Weather Service (NWS) station at Gillette, Wyoming [ http://cdo.ncdc.noaa.gov/ancsum/ACS ; accessed July 20, 2010]. No identifiable stream channels led into the reservoir, so runoff from precipitation falling on upslope areas was assumed negligible. Outflow from the reservoir consisted of evaporation and infiltration. Evaporation was estimated by the Penman method ( Rosenberg et al., 1983 ) to be about 15,000 m 3 for the 2-year period by using NWS data from Gillette [ http://www7.ncdc.noaa.gov/CDO/cdo ; accessed July 20, 2010]. By equating reservoir inflows and outflows, a total of 87,400 m 3 of water is estimated to have infiltrated from Skewed Reservoir. 4.2 Groundwater occurrence A perched groundwater mound began forming above the bedrock surface soon after the reservoir began receiving water. Groundwater-levels within the mound tended to rise quickly and decline slowly ( Fig. 3 ). Water-levels rose in all of the initially dry wells. Well 2s, at a distance of 27 m from the reservoir was the first well to show a water-level rise in response to the reservoir, 39 days after reservoir operations began. The groundwater mound reached a height of 7 m at well 2s in June 2004; by March 2008, the height was still in excess of 3 m ( Fig. 3 ). The time it took for groundwater-levels to rise in response to the reservoir generally increased with increasing distance from the reservoir ( Fig. 4 ). However, water-levels began to rise in well 12 (distance of 143 m from the reservoir) before they started rising at wells 11, 7, and 10 (distances of 84 m, 88 m, and 98 m, respectively, from the reservoir, Fig. 1 ). The screened interval for well 12 extended about 2 m into bedrock, whereas the screens for wells 11, 7, and 10 were set immediately atop bedrock in unconsolidated deposits ( Smith et al., 2011 ). Well 12 initially tapped into groundwater, so the water-level rise could conceivably be a pressure–pulse response as opposed to an indication of arrival of reservoir water. As discussed in subsequent sections, however, distinct changes in water chemistry at about the same time as the water-level rise appear to indicate that the rise at well 12 is the result of arrival of reservoir water. Thin sandstones or coal seams, which were noted in the well log for well 12 ( Smith et al., 2011 ), may act as preferential flow paths within the bedrock. Water-levels in wells screened in the alluvial aquifer (wells 13, 14, 15, 16, and 17) did not appear to be affected by the reservoir, a not-unexpected result given the highly permeable nature of the aquifer. Water-levels in well 14 ( Fig. 3 ) are typical of those in the other alluvial aquifer wells; these are the only wells that displayed seasonal water-level fluctuations. The water-level rise in well 14 in late 2003 and early 2004 is most likely due to increased flow in Beaver Creek (water chemistry discussed in subsequent sections indicates no effect of reservoir water on water in well 14). Beaver Creek is historically an ephemeral stream, but it has flowed continuously since 2003 because of discharge of CBNG produced water upstream from Skewed Reservoir. Water-level data suggest that most water that infiltrated from the reservoir was stored in and moved through the unconsolidated sediments that overlie bedrock. Water-table contours for May 6, 2004 ( Fig. 5 ) indicate that groundwater flow was generally southward from the reservoir in alignment with the valley axis. Groundwater in the Beaver Creek alluvial aquifer (south of well 12) moved in a predominantly westerly direction (down the topographic gradient). Water-table contours were not evenly spaced from the reservoir, reflecting the variability in hydraulic properties of sediments. The water table was substantially higher at well 8 than it was at several wells that were closer to the reservoir ( Fig. 5 ), illustrating the nonuniformity in flow and perhaps suggesting a preferential flow path between the reservoir and well 8. Although water-levels declined after reaching peaks in spring and summer 2004, the general shape of the water-table surface remained unchanged from that of May 2004. 4.3 Initial water chemistry Skewed Reservoir water was similar in chemical composition to local CBNG produced water ( Table 1 ). CBNG water is a sodium-bicarbonate type with an average TDS concentration of 2275 mg/L ( Table 1 ). The reducing environment of the coal-beds leads to depleted concentrations of oxygen, nitrate, sulfate, and other electron acceptors. The CBNG water chemistry data in Table 1 represent average concentrations from nine gas production wells located within 2 km of Skewed Reservoir. These wells did not discharge to the reservoir, but they produce from the same coal-bed (Big George) at about the same depth (408 m) as the wells that did discharge to the reservoir. Skewed Reservoir water had higher concentrations of sulfate (SO 4 ), chloride (Cl), magnesium (Mg), and sodium (Na) than the average CBNG water, likely the result of exposure to the sediments in which the reservoir was constructed. Jackson and Reddy (2007a) noted qualitatively similar differences between CBNG and reservoir waters at other sites in the Powder River Basin. Insight on natural shallow groundwater chemistry at the Skewed Reservoir site is provided by data from observation wells 2d, 12, and 14 ( Fig. 1 , Table 1 ), which were sampled prior to being affected by reservoir water. Well 2d is screened over a depth interval of 12.5–16 m in sandstone; its water was originally a sodium-bicarbonate-sulfate type with a TDS concentration of 1050 mg/L. Water from well 14, which is in the Beaver Creek alluvial aquifer, is a sodium-sulfate type with TDS concentration of 6121 mg/L. Wells 13, 15, 16, and 17 are also completed in the alluvial aquifer; water from those wells have a chemistry similar to that of the well 14 water. Well 12 is completed in bedrock that may be hydraulically connected to the alluvial aquifer. Water chemistry suggests that initial water in well 12 could be derived from a mixture of water from the shallow sandstone (well 2d) and the alluvial aquifer; it is a sodium-sulfate type with TDS concentration of 4051 mg/L. Groundwater chemistries at wells 2d, 12, and 14 are consistent with that reported at other locations within the Wasatch Formation. Bartos and Ogle (2002) and Rice et al. (2002) found TDS concentrations in sandstone aquifers within the Formation in the range of 200 to about 4000 mg/L; sulfate and bicarbonate were the dominant anions, and calcium and magnesium were the dominant cations. Lowry et al. (1986) reported TDS concentrations as high as 8200 mg/L in groundwater from the formation. 4.4 Post-reservoir groundwater chemistry The effect of reservoir water on groundwater is detectable by water chemistry as well as groundwater-levels. Wells 2d, 3, 5, 12, 15, and 16 all tapped groundwater prior to the reservoir accepting water, and water chemistry in samples from each of these wells was affected in some way by water infiltrating from the impoundment. Wells 13, 14, 17, and 18 also initially produced water, but water chemistry in those wells did not appear to be affected by the impoundment. Wells 1, 2s, 4s, 4d, 7, 8, 9, 10, and 11 were screened above bedrock and were initially dry, but all of these wells eventually produced water samples as a result of the rising of the groundwater mound. It should be noted that the water table beneath the reservoir rose above the elevation of the 7-m lysimeter and remained above it through 2005; therefore, water samples obtained from the lysimeter represent groundwater as opposed to water in the unsaturated zone. Water chemistry data for wells 0, 6, and 18 are not included in this discussion because of uncertainties in the intervals over which these wells are screened. Groundwater chemistry varies considerably across the small study area because of the diverse nature of subsurface sediments. Three distinct categories of post-reservoir groundwater have been identified on the basis of major ion chemistry, total dissolved solids, and pH ( Figs. 6A and 7 ). Category 1, which includes the 7-m lysimeter and wells 1, 2d, 7, 10, and 11, is characterized by a magnesium–sodium-sulfate water, TDS concentrations generally in excess of 20,000 mg/L (TDS concentrations in samples from the 7-m lysimeter have exceeded 100,000 mg/L ( Healy et al., 2008; Rice et al., 2011 )), and pH values between 6.5 and 7.5. Category 2 includes wells 2s, 8, and 12. The major ion chemistry of Category 2 waters is virtually identical to that of the Category 1 waters ( Fig. 6 A), magnesium–sodium-sulfate type with TDS concentrations generally greater than 20,000 mg/L. However, pH values for waters from Category 2 wells, typically less than 4, are much less than pH values for waters of Category 1 wells ( Fig. 7 ). Category 3 wells are those in the Beaver Creek alluvium (wells 13–17). As described in the previous section for water from well 14, the water from the Category 3 wells is a sodium-sulfate type ( Fig. 6 A), with TDS concentrations of about 6000 mg/L and pH close to 7 ( Fig. 7 ), similar characteristics to those reported for alluvial aquifers in other parts of the Powder River Basin ( Engle et al., 2011 ; K. Brus, US Bureau of Land Management, 2010, personal commun.). Waters from wells 3 (magnesium–sodium-sulfate type), 4d (sodium–calcium-sulfate water type), 4s (magnesium–sodium–calcium-sulfate type), 5 (magnesium-sulfate type), and 9 (magnesium–sodium-sulfate type) are chemically unique from waters of other wells ( Fig. 6B and 7 ); for convenience in discussions, these wells are grouped in Category 4. The magnesium–sodium-sulfate type and high TDS concentration of the Category 1 and 2 waters are formed from infiltrating water reacting with sediments underlying the reservoir. Salts and minerals have accumulated in these sediments for thousands of years because natural rates of water percolation through the unsaturated zone are low in this semiarid region. As described by Healy et al. (2008) the primary reactions that form this water appear to be dissolution of gypsum (CaSO 4 ·2H 2 O) and cation-exchange of calcium (Ca) for sodium (Na) and magnesium (Mg) on smectite clays: (1) CaSO 4 · 2 H 2 O = Ca 2 + + SO 4 2 - + 2 H 2 O (2) Ca 2 + + MgX 2 = Mg 2 + + CaX 2 (3) Ca 2 + + 2 Na X 2 = 2 Na + + CaX 2 Piper diagrams ( Fig. 6 C) show the shift in water chemistry for samples from wells 2d, 3 (Category 1), and 12 (Category 2) from their initial water types to the magnesium–sodium-sulfate type described above. TDS concentrations in samples from the Category 1 and 2 wells reached peaks relatively quickly, within 6 months after the impoundment began accepting water, and then declined rather slowly over time, as illustrated by data for the 7-m lysimeter and well 12 ( Fig. 8 ). The dominant ions remained unchanged as concentrations decreased over time. The large TDS concentrations are surprising, but the reactions described in Eqs. (1)–(3) are reversible, and if the moving groundwater encounters smectite clays with calcium-saturated exchange sites, then gypsum could be reprecipitated, and sodium and magnesium could be pulled back out of solution. However, the fact that TDS concentrations are declining with no change in water type implies dilution rather than reversal of the cation-exchange process. The low pH values in water from the Category 2 wells ( Figs. 7 and 9 ) are attributed to pyrite (FeS 2 ) oxidation: (4) 2 FeS 2 + 7 O 2 + 2 H 2 O = 2 Fe 2 + + 4 SO 4 2 - + 4 H + (5) 4 Fe 2 + + O 2 + 4 H + = 4 Fe 3 + + 2 H 2 O Pyrite was observed in thin coal seams in soil cores collected during installation of wells 2s, 8, and 12. The extent and connectedness of the coal seams were not apparent from the soil cores, and whether the well screens of the three wells intercept identical seams cannot be determined. pH values in water samples from wells 2s and 8 declined soon after the impoundment went into operation, but values rose to 7 or more by early 2006 ( Fig. 9 , data for well 8 are not included in this figure because declining water-levels prohibited collection of samples from well 8 after March 2006). Because of the rising pH values, well 2s and 8 samples for 2006–2009 fall into the Category 1 classification instead of the Category 2 classification. In contrast, pH values for water from well 12 remained at or below 3.5 from 2004 through 2009 ( Fig. 9 ), perhaps because of an abundance of pyrite. Solubilities of metals in groundwater are dependent on pH. Low pH values in waters from the Category 2 wells mobilized a number of metals, including aluminum, iron, and zinc ( Fig. 9 ). Metal concentrations in water from wells 2s and 8 declined when pH increased in 2006 ( Fig. 9 ). Concentrations of metals in well 12 water remained elevated through 2009; of particular note were aluminum and iron. Aluminum concentrations in samples from well 12 reached peak values greater than 1000 mg/L in 2004; concentrations exhibited a generally downward trend after that time, but concentrations still exceeded 100 mg/L in 2009. Iron concentrations in well 12 water reached a peak value of 246 mg/L in July 2007. Why this peak occurred so long after the impoundment went into operation remains a puzzling question. In any regard, elevated concentrations of trace metals would be expected to persist only as long as a source of pyrite or other sulfide minerals was available. Coals are the most likely source of sulfides in the shallow subsurface of this region. Observation wells down gradient from well 12 did not intersect any obvious coal seams, and reduced pH or elevated metals concentrations were not found in water samples from these wells. Hence, trace metal concentrations in water from down gradient wells should be less than those in samples from well 12 due to a lack of sulfides and the effects of dilution in the alluvial aquifer. Major ion chemistry of groundwater from the Beaver Creek alluvial wells (Category 3) remained essentially unchanged over the period of the study. However, increases in concentrations of TDS, nitrate, selenium, and to a lesser degree chloride in water from well 15 provide evidence that water in the alluvial aquifer has been affected by Skewed Reservoir ( Fig. 8 ). TDS concentrations in water from well 16 rose gradually over time ( Fig. 8 ) and provide a subtle indication that water at well 16 has been affected by the reservoir; however, selenium concentrations provide much stronger evidence of impact. The difficulty in understanding and predicting changes in groundwater chemistry at the Skewed Reservoir site is highlighted by the Category 4 wells (3, 4s, 4d, 5, and 9) whose water chemistries are unique relative to that of all other sampling points. The variable groundwater chemistry is likely a reflection of diverse subsurface sediments from discontinuous geologic units. Although groundwater chemistry is consistent within each of the Category 1, 2, and 3 waters, there is a lack of spatial continuity among sampling points in Category 1 and 2 wells. Only the Category 3 wells in the Beaver Creek alluvial aquifer adhere to some kind of spatial pattern in regard to groundwater chemistry. 4.5 Selenium in groundwater Selenium appears to be useful for tracing the movement of infiltrated water from Skewed Reservoir in groundwater. Well-defined breakthrough curves occur at the 7-m lysimeter and at wells 2s, 12, 15, and 16 ( Fig. 8 ), with breakthrough times consistent with distance from the reservoir. Selenium concentrations in excess of 300 μg/L occur throughout the groundwater mound ( Fig. 7 ). Selenium concentrations in groundwater appear to be unaffected by pH and do not follow the pH-dependent trend shown in Fig. 9 for aluminum, iron, and zinc. Instead, selenium concentrations follow a trend similar to that of TDS, chloride, and nitrate ( Fig. 8 ). Unsaturated-zone sediments are the apparent source of selenium in groundwater. Selenium concentrations in CBNG produced water in the Powder River Basin are generally less than 2 μg/L ( Rice et al., 2002 ; Jackson and Reddy, 2007b ). Upper Cretaceous and Tertiary marine-sediment-derived bedrock is the usual source of selenium in soils in the western United States ( Seiler et al., 1999 ), but such bedrock is not present in the study area. Oxidation of selenium-containing pyrite in the geologic past was cited as the most likely source of selenium in unsaturated-zone sediments at a site in the southern Powder River Basin ( Dreher and Finkelman, 1992 ). Soils immediately underlying Skewed Reservoir likely provided much of the selenium in the groundwater mound; however, the rising water table also may have mobilized selenium in areas away from the reservoir, particularly in areas where the water table rose by several meters. No attempt has yet been made to determine the chemical form of selenium in groundwater at the study site; ongoing research will address this issue. What seems apparent from the data is that selenium occurs in a highly water-soluble form and is little affected by pH over the range of 3–8 or by reactions that would retard its movement in groundwater. Redox potential of groundwater was not determined. Under natural conditions in this semiarid region, shallow water-table aquifers are expected to be aerobic. However, the slow dissipation rate of the groundwater mound may allow anaerobic conditions to form in parts of the study area. Selenate ( SeO 4 2 - is the common form that selenium takes in aerobic environments ( Brown, 1991 ); selenate has a low adsorptivity. Selenite ( SeO 3 2 - ) is the more common form in reducing environments. Selenite is easily adsorbed onto clay particles. The fact that elevated concentrations of selenium persist may argue against the presence of selenite, although transformation from selenate to selenite can be a slow process ( Dreher and Finkelman, 1992 ). Selenium concentrations can be used to calculate arrival times of reservoir water at observation wells as follows: (6) T i = t i - t 0 where T i is arrival time at observation well i , t i is the date of initial rise in selenium concentration in observation well i , t 0 is the date that water was first pumped into the impoundment (August 16, 2003), and it is assumed that the selenium concentration front is produced at the reservoir and propagated by groundwater movement. Travel times determined on the basis of arrival of the selenium front ( Fig. 10 ) are similar to response times calculated on the basis of water-level rises ( Fig. 4 ), with the exception that wells 15 and 16 did not have identifiable rises in water-levels, so travel times could not be calculated on that basis. Arrival times determined on the basis of selenium concentrations were 474 days for well 15 (177 m from the reservoir) and 814 days for well 16 (198 m), which was the farthest sampling point from the impoundment to show effects from the CBNG water. Linear velocity between the impoundment and well 16 is about 0.25 m/d, as determined from selenium concentrations. 5 Discussion Variability in hydraulic properties of the geologic material at the Skewed Reservoir site complicates efforts to accurately delineate groundwater flow paths. Water-level data suggest that most water that infiltrated from the reservoir was stored in and moved through the unconsolidated sediments and that these sediments are hydraulically connected to flow paths within the bedrock. The arrival of reservoir water at well 12 prior to arrival at wells nearer the reservoir indicates the presence of a preferential flow path, perhaps a thin sandstone or coal seam, between the reservoir and the well. Groundwater is exposed to geologic material of differing chemical properties resulting in substantial variability in groundwater chemistry. Measured values of pH in groundwater ranged from less than 3 to more than 9; TDS concentrations ranged from less than 5000 to more than 100,000 mg/L. The primary reactions affecting groundwater chemistry are gypsum dissolution, cation-exchange, and pyrite oxidation. Four categories of groundwater were identified on the basis of water type, TDS concentration, and pH. With the exception of wells screened in the alluvial aquifer, there were no spatial patterns in groundwater chemistry. Elevated concentrations of TDS, chloride, nitrate, and selenium in water samples from wells 15 and 16 indicate that the alluvial aquifer was affected by Skewed Reservoir, even though water-levels in those wells appear unaffected. Concentrations of most constituents in the solute plume are decreasing gradually over time. As groundwater flows into the Beaver Creek alluvial aquifer, dilution may reduce concentrations further. An unexpected finding of this study is the high selenium concentrations in groundwater. Selenium is a naturally occurring element that most animals need in trace quantities for proper cell function; however, selenium can bioaccumulate within organisms to toxic levels. Aquatic life can be adversely impacted by chronic exposure to water with concentrations of 5 μg/L or higher ( Wyoming Department of Environmental Quality, 2000 ). Water samples from 18 of the 24 wells and lysimeters at the study site contained selenium concentrations in excess of 300 μg/L at some point during the study. A maximum concentration of 16 mg/L was measured in a sample obtained from the 7-m lysimeter. The specific chemical form of the selenium was not determined, but the mobility of selenium is noteworthy. Selenium is the most useful chemical for tracing subsurface movement of infiltrated water from Skewed Reservoir. Susceptibility of surface and groundwater to selenium contamination in Wyoming has been the topic of several studies. Most of this work has looked at irrigation practices ( Seiler et al., 2003 ) and mining practices ( Dreher and Finkelman, 1992; See et al., 1995 ). The map of areas susceptible to selenium contamination from irrigation within the mountain west region of the United States developed by Seiler et al. (2003) does not include the Powder River Basin as an area of high susceptibility, mainly because of the absence of upper Cretaceous or Tertiary marine-derived sedimentary deposits. Further research on the source and chemical form of selenium in the vicinity of Skewed Reservoir is ongoing. The interaction between surface water in Beaver Creek and groundwater within the underlying alluvial aquifer is unknown. Historically, Beaver Creek has been an ephemeral stream with surface flow only during certain times of the year. In semiarid regions of the United States, ephemeral streams such as this are commonly losing streams and may be sources of groundwater recharge ( Healy, 2010 ). Beaver Creek has flowed continuously since 2003 because of surface discharge of CBNG produced water upstream from Skewed Reservoir. The increased surface flow is reflected in groundwater-level rises in wells 13–17. It is conceivable that the creek is a sink for groundwater discharge along some reaches. In any regard, most water from Skewed Reservoir flows into the alluvial aquifer. Its course from there is unknown. It may flow into Beaver Creek; it may instead flow to the alluvial aquifer associated with the Powder River (about 2 km west of the study area), where it might discharge to the river. Will groundwater that originated in Skewed Reservoir eventually be discharged to land surface? If so, where and when? What will be the concentrations of constituents such as TDS, sodium, and selenium? Will aquatic habitat be affected? Answers to these important questions can come only with installation of additional monitoring points down gradient from the study area and years of continued data collection. It is important to discuss how the findings of this study relate to data collected at other locations in the Powder River Basin. On the basis of BLM and DEQ databases, TDS and Se concentrations in groundwater at other sites generally are much lower than those found at the Skewed Reservoir site. Unpublished BLM data from seven other sites in the Basin [K. Brus, US BLM, written commun., 2010) show that groundwater chemistry is highly variable. At four of the seven BLM monitoring sites, groundwater samples from at least one sampling point had selenium concentrations greater than 100 μg/L; concentrations in excess of 1 mg/L were found at four sampling locations. Total dissolved solids concentrations in samples from the BLM database were generally less than 15,000 mg/L; however, concentrations as high as 53,000 mg/L were reported for one well. Values of pH less than 5 were reported in samples from two of the BLM wells; iron concentrations in samples for these wells were greater than 100 mg/L. As of December 2010, selenium concentrations in excess of 100 μg/L were measured in water samples from 23 of the wells in the DEQ database [C. Steinhorst, DEQ, written commun., 2011); pH values less than 5 occurred in samples from 8 wells. Preliminary results of groundwater-quality monitoring at three CBNG impoundment sites in the Powder River Basin showed that TDS concentrations in groundwater generally increased due to infiltration from the impoundments ( Wheaton and Brown, 2005 ); the maximum reported TDS concentration was 12,400 mg/L. Groundwater chemistry at the Skewed Reservoir site may not be typical of that near other impoundments in the Powder River Basin, but these additional data indicate that phenomena identified at the study site occur at other sites within the Basin. Skewed Reservoir may be the most studied CBNG produced water impoundment in the Powder River Basin. Complexities in hydraulic and chemical properties of geologic materials demonstrate the difficulty in predicting the fate of solutes in groundwater at the study site and underscore the importance of including multiple observation wells for characterization of groundwater flow and chemistry at impoundment sites within the Basin. 6 Summary Groundwater movement and chemistry in the vicinity of an impoundment for CBNG produced water in the Powder River Basin, Wyoming were studied from 2003 through 2009. A groundwater mound formed atop bedrock within initially unsaturated unconsolidated deposits that historically have seen little natural recharge. Heterogeneity in physical and chemical properties of sediments is reflected in the highly variable groundwater chemistry. Sulfate, bicarbonate, sodium, and magnesium are the dominant ions in all areas, but substantial variability exists in their concentrations and relative proportions. Within the study area, pH varied from less than 3 to more than 9 in different areas, and TDS concentrations range from less than 5000 to greater than 100,000 mg/L (pH and TDS concentrations of the CBNG produced water were about 8.4 and 2274 mg/L, respectively). The produced water is not the source of most solutes in the groundwater mound; the primary source of solutes are salts and minerals that have accumulated in the unsaturated-zone sediments over thousands of years because of naturally low groundwater recharge rates. Important reactions that affect groundwater chemistry include cation-exchange, gypsum dissolution, and pyrite oxidation. Elevated trace metal concentrations were detected in samples from three wells with pH less than 5. Selenium concentrations in excess of 300 μg/L were detected in samples from most wells. Elevated concentrations of selenium in groundwater samples indicated that water from Skewed Reservoir arrived at an alluvial aquifer, at a distance of 177 m from the reservoir, 474 days after the reservoir began accepting water. The complexity in groundwater chemistry illustrates the difficulty in predicting effects of impoundments for CBNG produced water on surface and groundwater in the semiarid Powder River Basin and highlights the usefulness of multiple observation wells for impoundment site characterization. References Bartos and Ogle, 2002 Bartos, T.T., Ogle, K.M., 2002. Water quality and environmental isotopic analyses of ground-water samples collected from the Wasatch and Fort Union Formations in areas of coalbed methane development – implications to recharge and ground-water flow, eastern Powder River Basin, Wyoming, US Geol. Surv. Water Resour. Inv. Rep. 02-4045, 88 pp. Brown, 1991 Brown, T.H., 1991. Solubility, sorption, and redox relationships for selenium in reclaimed environments. In: Severson, R.C., Fisher Jr., S.E., Gough, L.P. (Eds.), Proceedings of the 1990 Billings Land Reclamation Symposium on Selenium in Arid and Semiarid Environments. US Geol. Surv. Circular 1064, pp. 25–34. Davis et al., 2009 W.N. Davis R.G. Bramblett A.V. Zale C.L. Endicott A review of the potential effects of coal bed natural gas development activities on fish assemblages of the Powder River geologic basin Rev. Fish. Sci. 17 3 2009 402 422 De Bruin et al., 2000 De Bruin, R.H., Lyman, R.M., Jones, R.W., Cook, L.W., 2000. Coalbed methane in Wyoming. Wyoming State Geol. Surv. Inform. Pamphlet No. 7. Dennis, 2007 Dennis, R.S., 2007. Continuous ion exchange for Wyoming CBM produced-water purification: proven experience. In: Proceedings of the Society of Petroleum Engineers E and P Environmental and Safety Conference 2007, pp. 321–324. Dreher and Finkelman, 1992 G.B. Dreher R.B. Finkelman Selenium mobilization in a surface coal mine, Powder River Basin, Wyoming, USA Environmental Geology and Water Sciences 19 3 1992 155 167 Engle et al., 2011 Engle, M.A., Bern, C.R., Healy, R.W. Sams, J.I., Zupancic, J.W., Schroeder, K.T., 2011. Tracking solutes and water from subsurface drip irrigation application of coalbed methane produced waters, Powder River Basin, Wyoming, Environ. Geosci. Ganjegunte et al., 2008 G.K. Ganjegunte L.A. King G.F. Vance Cumulative soil chemistry changes from land application of saline–sodic waters J. Environ. Qual. 37 Suppl. 5 2008 S128 S138 Glass, 1997 Glass, G.B., 1997. Coal geology in Wyoming, Wyoming State Geol. Surv. Reprint No. 63, reprinted from the 1997 Keystone Coal Industry Manual, 21 p. Harris and Smith, 2009 S.H. Harris R.L. Smith In situ measurements of microbially-catalyzed nitrification and nitrate reduction rates in an ephemeral drainage channel receiving water from coalbed natural gas discharge, Powder River Basin, Wyoming, USA Chem. Geol. 267 1–2 2009 77 84 Healy et al., 2008 R.W. Healy C.A. Rice T.T. Bartos M.P. McKinley Infiltration from an impoundment for coal-bed natural gas, Powder River Basin, Wyoming: evolution of water and sediment chemistry Water Resour. Res. 44 6 2008 Healy, 2010 R.W. Healy Estimating groundwater recharge 2010 Cambridge Univ. Press Cambridge Hem, 1992 Hem, J.D., 1992. Study and interpretation of the chemical characteristics of natural water, US Geol. Surv. Water Supply Pap. 2254, 263 pp. Jackson and Reddy, 2007a R.E. Jackson K.J. Reddy Geochemistry of coalbed natural gas (CBNG) produced water in Powder River Basin, Wyoming: salinity and sodicity Water, Air, Soil Pollut. 184 1–4 2007 49 61 Jackson and Reddy, 2007b R.E. Jackson K.J. Reddy Trace element chemistry of coal bed natural gas produced water in the Powder River Basin, Wyoming Environ. Sci. Technol. 41 17 2007 5953 5959 Johnston et al., 2008 C.R. Johnston G.F. Vance G.K. Ganjegunte Irrigation with coalbed natural gas co-produced water Agri. Water Manage. 95 11 2008 1243 1252 Lowry et al., 1986 Lowry, M.E., Wilson Jr., J.F., et al., 1986. Hydrology of Area 50, Northern Great Plains and Rocky Mountain coal provinces, Wyoming and Montana, US Geol. Surv. Open-File Rep. 83-545, 137 pp. Mace and Amrhein, 2001 J.E. Mace C. Amrhein Leaching and reclamation of soil irrigated with moderate SAR waters Soil Sci. Soc. Am. J. 65 2001 199 204 Rice et al., 2002 Rice, C.A., Bartos, T.T., Ellis, M.S., 2002. Chemical and isotopic composition of water in the Fort Union and Wasatch Formations of the Powder River Basin, Wyoming and Montana: Implications for coalbed methane development. In: Schwowchow, S.D., Nuccio, V.F. (Eds.), Coalbed Methane of North America, II, Rocky Mtn. Assoc. Geologists, Denver, Colo, pp. 53–70. Rice et al., 2011 Rice, C.A., Healy, R.W., Bartos, T.T., 2011. Hydrogeologic data collected in the vicinity of Skewed Reservoir, an impoundment for coal-bed natural gas produced water, Powder River Basin, Wyoming, US Geol. Surv. Open-File Rep. Rosenberg et al., 1983 N.J. Rosenberg B.L. Blad S.B. Verma Microclimate and the Biological Environment 2d ed. 1983 John Wiley and Sons New York 495 pp Ross and Zoback, 2008 H.E. Ross M.D. Zoback Sub-hydrostatic pore pressure in coalbed and sand aquifers of the Powder River Basin, Wyoming and Montana, and implications for disposal of coalbed-methane-produced water through injection Rocky Mt. Geol. 43 2 2008 155 169 See et al., 1995 See, R.B., Reddy, K.J., Vance, G.F., Fadlelmawla, A.A., Blaylock, M.J., 1995. Geochemical processes and the effects of natural organic solutes on the solubility of selenium in coal-mine backfill samples from the Powder River Basin, Wyoming, US Geol. Surv. Water Resour. Invest. Rep. 95-4200. 55 pp. Seiler et al., 1999 Seiler, R.L., Skorupa, J.P., Peltz, L.A., 1999. Areas susceptible to irrigation-induced selenium contamination of water and biota in the western United States, US Geol. Surv. Circular 1180. 36 pp. Seiler et al., 2003 Seiler, R.L., Skorupa, J.P., Naftz, D.L., Nolan, B.T., 2003. Irrigation-induced contamination of water, sediment, and biota in the western United States – synthesis of data from the National Irrigation Water Quality Program, US Geol. Surv. Prof. Paper 1655. 123 pp. Smith et al., 2011 Smith, B.D., Bartos, T.T., Sweat, M.J., McKinley, M.P., 2011. Induction Conductivity and Natural Gamma Logs for Monitor Wells, Skewed Reservoir Research Site, Powder River Basin, Johnson County, Wyoming, U.S. Geol. Surv. Open-File Rep. 2011-1046. Smith et al., 2009 R.L. Smith D.A. Repert C.P. Hart Geochemistry of inorganic nitrogen in waters released from coal-bed natural gas production wells in the Powder River Basin, Wyoming Environ. Sci. Technol. 43 7 2009 2348 2354 Soil Survey Laboratory, 1995 Soil Survey Laboratory, 1995. Soil Survey Laboratory Information Manual, Natural Resource Conservation Service. Soil Survey Invest. Rep. No. 45, Version 1.0, May 1995, 316 p. US Bureau of Land Management, 2003a US Bureau of Land Management, 2003a. Final environmental impact statement and proposed plan amendment for the Powder River Basin Oil and Gas Project (WY-070-02-065). US Bureau of Land Management, 2003b US Bureau of Land Management, 2003b. Record of decision and resource management plan amendments for the Powder River Basin Oil and Gas Project (WY-070-02-065). Vance et al., 2008 G.F. Vance L.A. King G.K. Ganjegunte Soil and plant responses from land application of saline–sodic waters: implications of management J. Environ. Qual. 37 Suppl. 5 2008 S139 S148 Welch, 2009 J. Welch Reverse osmosis treatment of CBM produced water continues to evolve Oil Gas J. 107 37 2009 45 50 Wheaton and Brown, 2005 Wheaton, J., Brown, T.H., 2005. Predicting changes in groundwater quality associated with coalbed natural gas infiltration ponds. In: Zoback, M.D. (Ed.), Western Resources Project Final Report–Produced Groundwater Associated With Coalbed Natural Gas Production in the Powder River Basin, WY State Geol. Surv. Rep. Invest. No. 55, pp. 45–70. Wyoming Department of Environmental Quality, 2000 Wyoming Department of Environmental Quality, 2000. Water quality rules and regulations (Chapter 1).
更多查看译文
关键词
Produced water,Coal-bed natural gas,Coal-bed methane,Selenium,Impoundment,Powder River Basin
AI 理解论文
溯源树
样例
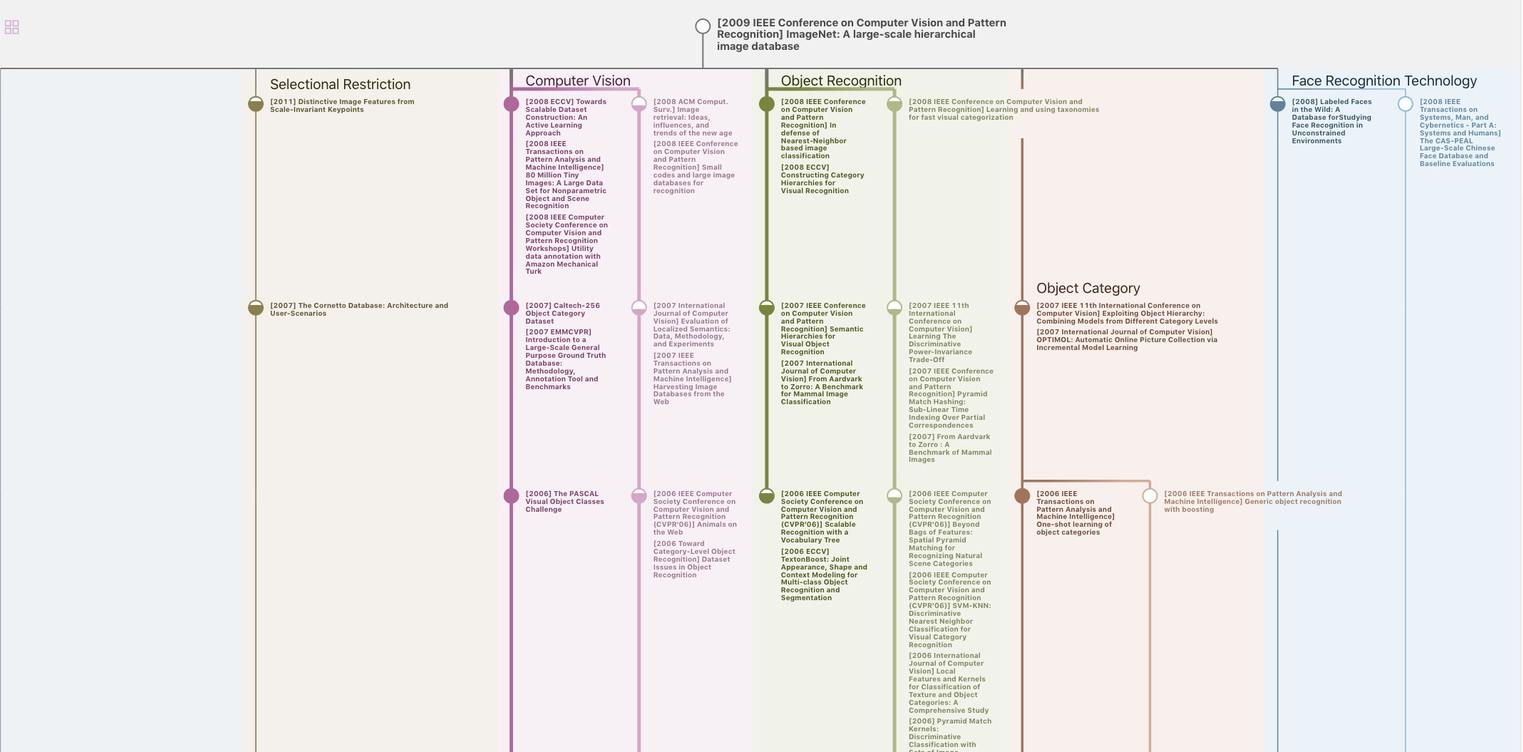
生成溯源树,研究论文发展脉络
Chat Paper
正在生成论文摘要