Brain hypoxanthine concentration correlates to lactate/pyruvate ratio but not intracranial pressure in patients with acute liver failure
Journal of Hepatology(2010)
摘要
Methods In 17 patients (aged 18–60 years) with acute liver failure and severe hyperammonemia (182 ± 36 μM (mean ± SD)), cerebral microdialysis was performed, and ICP and CPP were monitored. Microdialysate concentrations of hypoxanthine, inosine, lactate, and pyruvate were measured. Results The hypoxanthine concentration was 23.0 ± 12 μM in early samples and 11.7 ± 6.8 μM in late samples (normal level ∼2.0 μM). The inosine concentration was 7.2 ± 7.1 μM and 2.8 ± 1.6 μM, and the LP ratio was 55.8 ± 21.6 and 45.6 ± 20.8, respectively (normal level ∼18). Hypoxanthine correlated significantly to LP ratio ( r 2 = 0.40, p <0.01) while inosine did not. The purine levels and L/P ratio did not correlate to ICP or CPP, nor did they differ between patients with high ICP (>20 mmHg, n = 9) and patients without ( n = 8). Conclusions This study shows that the high cerebral LP ratio correlates to the hypoxanthine level in patients with acute liver failure. However, these metabolic alterations were not related to the development of intracranial hypertension. Abbreviations ALF acute liver failure ATP adenosine-tri-phosphate CBF cerebral blood flow CPP cerebral perfusion pressure ICH intracranial hypertension ICP intracranial pressure LP lactate/pyruvate MAP mean arterial pressure Keywords Acute liver failure Cerebral microdialysis Mitochondrial dysfunction Adenosine Inosine Hypoxanthine Lactate/pyruvate ratio Introduction Acute liver failure (ALF) is frequently complicated by intracranial hypertension (ICH) that may lead to serious brain damage and death [1,2] . The underlying pathogenesis is complex and not fully understood, but a multitude of events are likely to be involved [3] . One key factor is hyperammonemia, where ammonia enters the brain through capillaries and is detoxified by astrocytes [4,5] . Ammonia detoxification results in high levels of extra- and intracellular glutamine that has been demonstrated in vitro to induce oxidative stress and to compromise the mitochondrial function [6] . Clinically, both high arterial ammonia and cerebral extracellular glutamine content have been shown to correlate with cerebral edema and high intracranial pressure (ICP) [2,3,7,8] . Furthermore, our group has previously reported that the extracellular cerebral glutamine concentration also correlates with the extracellular lactate/pyruvate (LP) ratio in the brain of patients with ALF [9] . Using a rat model of acute hyperammonemia it has been demonstrated that the brain content of adenosine-tri-phosphate (ATP) decreases significantly [10] , and more recently, cerebral accumulation of the purines hypoxanthine, and xanthine (which are degradation products of adenosine) has been shown in the same model [11] . These results comply with the hypothesis of impaired mitochondrial function, and the increase in purine levels can be viewed as a spill-over of adenosine derivates due to reduced ATP synthesis. We performed this clinical study in order to translate these findings from in vitro and animal experiments. We hypothesized that the extracellular brain content of hypoxanthine, and the related purine inosine, correlated with the increased LP ratio in patients with ALF and persistent hyperammonemia or clinical signs of ICH. Accumulation of degradation products of adenosine, i.e. inosine and hypoxanthine, would be expected to be a consequence of both decreased synthesis of ATP, and release of intracellular adenosine and ATP to the extracellular space. Furthermore, we expected the purines and LP ratio to correlate positively with ICP and negatively with the cerebral perfusion pressure (CPP). By measuring ICP, CPP, and the cerebral extracellular levels of inosine, hypoxanthine, lactate, and pyruvate using microdialysis, we tested this hypothesis in the clinical setting of ALF. Patients and methods Seventeen patients (aged 18–60 years, 10 females) with ALF and persistent arterial hyperammonemia (>150 μM for 24 h) or clinical signs of ICH were included within 24 h after intubation. All patients were fully volume resuscitated before inclusion. Baseline characteristics are listed in Table 1 . Measurement of cerebral metabolism was done with microdialysis using a CMA70 microdialysis catheter (CMA, Solna, Sweden) placed frontally in the right hemisphere after administration of 2 units of pooled platelets, activated coagulation factor VII (NovoSeven®, Novo Nordisk A/S, Copenhagen, Denmark), and fresh frozen plasma. The procedure was performed by a consulting neurosurgeon (J.H. or L.J.). The microdialysis catheter was connected to a CMA106 micro-infusion pump and continuously perfused with artificial cerebrospinal fluid at a rate of 0.3 μl/min. Repeated samples of approximately 100 μl were collected and analyzed for lactate and pyruvate concentration and then stored at −80 °C for later measurement of hypoxanthine and inosine. For each patient, one sample was taken preferably within the first day of intracranial catheter insertion, and in addition another sample was taken the next day or subsidiary, one of the following days. Sampling was avoided during episodes of prolonged hypotension and seizures, and at the terminal phase of non-survivors, and the patients were allowed to participate simultaneously in other microdialysis protocols. This procedure influenced the sampling intervals between early and late samples, which ranged from 14 to 109 h. The microdialysis method has been evaluated in previous studies from both our group and others [8,9,12] and has an estimated recovery rate of 70–90% for small hydrophilic molecules with a membrane cut-off at 20 kDa and a dialysate flow-rate of 0.3 μl/min. Dialysate concentrations of lactate and pyruvate were determined by enzymatic oxidation and colorimetric measurements (CMA600 Microdialysis Analyzer). The CMA600 analyzer has a linear range from 0.1 to 20 mM for lactate and 0.01 to 15 mM for pyruvate. Analysis of hypoxanthine and inosine was performed by Pronexus Analytical AB (Stockholm, Sweden) by high performance liquid chromatography using a Nucleosil C18 column with 3 μm beads, 100 × 4 mm (Knauer, Germany), a mobile phase of 0.01 M ammonium phosphate pH 6.0, methanol 10%, and UV detection at 254 nm. ICP was monitored with a Camino pressure catheter (Integra, USA) placed in the cortical parenchyma of the frontal lobe together with the microdialysis catheter. A radial artery catheter was used for monitoring mean arterial pressure (MAP). ICP and MAP were registered at the time point where each micro-vial was taken for analysis. CPP was calculated as the difference between MAP and ICP. The patients received standard intensive care, were sedated on propofol and fentanyl, and mechanically ventilated (Servo 900, Siemens, Solna, Sweden). Patients with acute renal failure received renal replacement therapy with continuous venovenous hemodiafiltration (PrismaFlex, Gambro, Stockholm, Sweden). Patients with episodes of ICP >20 mmHg or neurological signs hereof, received infusion of mannitol, indomethacin, and/or hypertonic saline. The increase in ICP had to last for at least 5 min and to be unrelated to external factors such as airway suction or change of position in bed. Ethics Inclusion in the study was done after a written consent had been obtained from the closest relative and the clinical setup was approved by the Ethical-Scientific Committee in Copenhagen (Protocol No. KF 01-002/00) and conformed to the ethical guidelines of the 1975 Declaration of Helsinki. Statistics Correlations between inosine and hypoxanthine and LP ratio were evaluated by linear regression analysis. Pearson’s correlation coefficient was used for calculation of r 2 . Results are expressed as means ± 1 SD and are compared between groups (early/late and ICP >20 mmHg/ICP <20 mmHg) by the parametric student’s t -test (paired or unpaired, respectively). p -Values less than 0.05 were considered significant. Results At inclusion, the mean arterial ammonia concentration was 182 ± 36 μM. Collection of early microdialysis samples was started between three and 28 h after insertion of the microdialysis catheter, and collection of late samples was started 54 ± 30 h later. The cerebral inosine concentration was 7.2 ± 7.1 μM in the early samples and 2.8 ± 1.6 μM in the late samples, while hypoxanthine levels were 23.0 ± 12.0 and 11.7 ± 6.8 μM, respectively. The LP ratio (unitless) was 55.6 ± 20.9 and 45.6 ± 20.8 in the early and late samples, respectively. We found a positive correlation between cerebral extracellular levels of hypoxanthine and LP ratio in both the early and late sample groups ( r 2 = 0.38, p <0.05 and r 2 = 0.40, p <0.01, respectively), see Figs. 1 and 2 . The LP ratio and inosine showed a weaker and non-significant correlation ( r 2 = 0.18, NS and r 2 = 0.17, NS). The change from early to late samples in LP ratio, and hypoxanthine and inosine did also correlate significantly (LP ratio vs. hypoxanthine: r 2 = 0.35 and LP ratio vs. inosine: r 2 = 0.31, p <0.05) indicating that the purine concentrations and LP ratio tend to follow each other over time. In contrast to our hypothesis, we did not find a significant correlation between ICP and hypoxanthine (early samples: r 2 = 0.12, NS and late samples: r 2 = 0.15, NS), inosine (early samples: r 2 = 0.07, NS and late samples: r 2 = 0.02, NS) or LP ratio (early samples: r 2 = 0.21, NS and late samples: r 2 = 0.00, NS). Neither did CPP correlated with hypoxanthine (early samples: r 2 = 0.08, NS and late samples: r 2 = 0.00, NS), inosine (early samples: r 2 = 0.07, NS and late samples: r 2 = 0.02, NS) or LP ratio (early samples: r 2 = 0.01, NS and late samples: r 2 = 0.00, NS). The cerebral metabolites did not correlate to the Model of End-stage Liver Disease (MELD) score or the Sequential Organ Failure Assessment (SOFA) score. The mean CPP was 70 ± 12 mmHg in the early samples and 63 ± 18 mmHg in the late samples. Seven patients had a CPP indicating cerebral hypoperfusion (<60 mmHg) at either the early or late sample time. These samples did not differ significantly from the rest of the samples in regards to inosine or hypoxanthine but the LP ratio was significantly higher in the late set of samples of the patients with a CPP below 60 mmHg (56.6 ± 13.2 vs. 37.9 ± 22.2, respectively, p <0.05). Since only two patients had an ICP above 20 mmHg at the time point of microdialysis sampling, the low CPP must primarily be ascribed to hypotension and instability of the systemic circulation. No patients were hypoxic during the study. During the study period, 10 patients (59%) had episodes of ICP >20 mmHg or neurological signs of brain edema (pupillary abnormalities or seizures), and received either indomethacin (one patient), mannitol + indomethacin (four patients), mannitol + hypertonic saline (four patients) or mannitol + hypertonic saline + indomethacin (one patient). All but two patients responded to the treatment, i.e. ICP decreased below 20 mmHg within 1 h. The late samples were taken after the treatment except for two cases. Since the microdialysis sampling time was at least 6 h, and thereby exceeded the duration of ICH episodes, the late samples cannot reflect the actual brain metabolism during ICH but rather a mixture of microdialysate obtained before, during, and after surges of high ICP. Dividing the patients into groups, with or without episodes of ICP >20 mmHg during the entire study period, did not result in statistically significant differences in the concentrations of hypoxanthine (23.3 ± 10.1 μM vs. 22.6 ± 14.6 μM), inosine (5.3 ± 4.4 μM vs. 9.4 ± 9.1 μM), or LP ratio (55.0 ± 22.7 vs. 56.4.1 ± 20.1) in the early samples. The same applied to the late samples: hypoxanthine 12.0 ± 7.0 μM vs. 11.3 ± 7.0 μM, inosine 3.1 ± 2.1 μM vs. 2.5 ± 0.7 μM, and LP ratio 49.7 ± 22.8vs. 40.9 ± 16.3. The purines had a significant higher level in the early samples compared to the late samples, most marked by hypoxanthine (23.0 ± 12.0 vs. 11.7 ± 6.8 μM, p <0.01). Seven patients underwent high volume plasmapheresis within 24 h of inclusion. These patients did not differ from the rest of the patients in regard to the measured cerebral metabolites but, as expected, had a lower INR at the time of inclusion (2.6 ± 0.6 vs. 4.8 ± 2.3, p <0.05). Five out of the 17 patients (29%) survived for 30 days. Four patients underwent orthotopic liver transplantation and three of them survived. The predominant cause of death was multi-organ failure with a combination of cardiovascular collapse, renal, and hepatic failure. All but one patient with ICP exceeding 20 mmHg died, two of these patients died due to cerebral incarceration, one within 24 h after transplantation, while the rest of the patients died due to multi-organ failure. Discussion The pathogenesis of cerebral edema in ALF is incompletely understood, but a number of experimental studies have shown that the increased brain water content is of primary cytotoxic origin and related to a high cerebral ammonia level [3,13] . In vitro , astrocyte swelling correlates with mitochondrial dysfunction after induction of nitrosative stress by exposure to ammonia (5 mM) [14] , which is associated with intracellular glutamine accumulation and induction of the mitochondrial permeability transition [15] . A compromised oxidative metabolism is speculated to result in dysregulation of the cell volume, as maintaining ion and water homeostasis is dependent on energy-rich compounds such as ATP. The cell swelling is further promoted by the osmotic activity of high intracellular glutamine concentrations, which, however, can be counteracted to some extent by the cellular release of other organic osmolytes such as myoinositol and sorbitol [16,17] . The development of cerebral edema is also related to increased levels of proinflammatory mediators [18,19] and underlines the fact that the mechanisms involved are of a multifactorial nature. Previous clinical studies have shown clear correlations between e.g. (i) arterial ammonia concentration and the risk of cerebral herniation [1,2] , (ii) arterial ammonia and cerebral glutamine concentrations [8] (iii) as well as cerebral glutamine and LP ratio [9] . The present results show a correlation between the LP ratio and the ATP degradation products (hypoxanthine and inosine), a fact that could be explained by multiple modulations of the carbohydrate metabolism such as accelerated glycolysis, inhibition of rate limiting steps in the Krebs’ cycle, and compromised oxidative phosphorylation in the mitochondrial inner membrane [4,15,20] . However, our modest correlation coefficients indicate that other factors are affecting the extracellular purine levels as well. It has previously been demonstrated that acute hyperammonemia leads to accelerated ATP-consumption due to activation of NMDA-receptors and Na+/K+-ATPase [10] . Some of the variations in hypoxanthine levels could also be due to changes in purinergic signaling (e.g. active transport of intracellular ATP and adenosine to the extracellular compartment). We found rather high levels of the LP ratios compared to earlier reported reference values found in cortex of patients undergoing surgery for benign cerebral tumors (18 ± 5) [12] . Furthermore, the dialysate concentrations of hypoxanthine in our study, by far exceeded what has previously been reported (2–6 μM in patients with traumatic brain injury [21] and 1–2 μM in intact rat cortex [22] ). Although the literature is scarce on reference values for these substances in human cortex, our results appear to reflect a highly disturbed metabolism in the brain. Interestingly, increased extracellular purine levels have been associated to hypoxia and ischemia in previous studies [22,23] , but in contrast to what previously shown, here we demonstrate an association between high LP ratio and high hypoxanthine in the absence of hypoxia. Hypoperfusion was associated with an increased LP ratio but patients without hypoperfusion still had abnormally high LP ratios. Furthermore, CPP did not correlate well with hypoxanthine or inosine, indicating that other factors influence the purine levels such as ammonia toxicity and mitochondrial dysfunction. This finding could be the result of a metabolic condition described as “cytopathic hypoxia” or “dysoxia”, terms used in the sepsis literature. Direct measurement of ATP and lactate levels in the brain by e.g. MR-spectroscopy would have been an elegant way of investigating our hypothesis more thoroughly, but the clinical instability and the need for intensive monitoring of these patients did not allow us to follow this procedure. We also measured adenosine levels in the microdialysate but found extremely low or undetectable levels in most samples (data not shown). This could be explained if adenosine was mainly released to the extracellular space prior to our microdialysis study, i.e. in the first 24 h of coma grades III to IV, and/or reflects the fact that the short extracellular half-life of adenosine makes it difficult to collect in microdialysate, being dependent on diffusion across the brain tissue. In the extracellular compartment, adenosine is rapidly degraded to inosine through deamination by adenosine deaminase. Inosine is in turn deribosylated by purine nucleoside phosphorylase to hypoxanthine, which has a much longer half-life in vivo . Therefore, the concentration of adenosine in the microdialysate may poorly reflect transient increases in extracellular cortical adenosine, but inosine, and especially hypoxanthine levels, would remain high for a longer period of time and reflect previous fluctuations in adenosine concentration [24] . The purine levels were found to be lower in late samples, this result furthermore supports the above mentioned theory but could also be the consequence of depletion of the available adenosine, restoration of oxidative metabolism, increase in the oxidation of hypoxanthine to xanthine, or any combination of these processes. Unfortunately, the available data does not allow us to draw any firm conclusions. We did not find a significant correlation between ICP and the purine levels or significant differences in brain metabolites by dividing the patients into groups with or without ICP exceeding 20 mmHg during the study. In contrast to previous findings reported by our group [9] , we did not find a significant correlation between ICP and LP ratio. This could indicate that patients with acute liver failure belong to a rather heterogeneous group and that the pathogenesis of high ICP is more complex than a simplistic model stating that high arterial ammonia leads to high intracerebral glutamine followed by elevated LP ratio and hypoxanthine, and finally, increased ICP. Additionally, different degrees of low-grade brain edema [25] might have been present in spite of a normal ICP, however, we did not have the possibility to measure the water content of the brain. Our hypothesis was moreover challenged by the fact that CPP did not correlate well with hypoxanthine, inosine, or LP ratio. Clearly, other factors, such as fluctuations in cerebral blood flow (CBF) caused by systemic inflammation, infections, electrolyte disturbances, and cardiovascular instability, must contribute to the development of a disturbed metabolism in the brain and add to the difficulty of interpreting the influence of the individual parameters on e.g. ICP. Apart from the fact that we did not measure CBF, we did, however, not find it meaningful to perform multivariate analyses due to the limited number of patients in this study. Finally, it is noteworthy to mention that 9 (53%) of these high-risk patients developed high ICP during our study and only one of them survived for 30 days. Only two patients died due to cerebral incarceration but the cerebral edema contributed significantly to the picture of multi-organ failure. In contrast, four out of the eight patients who did not develop high ICP, were alive after 30 days. In conclusion, our results show that in patients with ALF and hyperammonemia, the cerebral concentration of hypoxanthine is increased and correlates to the LP ratio, which may indicate impaired cerebral oxidative metabolism. The extracellular hypoxanthine is most likely a product of transient increases in extracellular adenosine levels prior to our observations, but further studies, on the role of adenosine and purinergic signaling in ALF, are needed to clarify this hypothesis. Our data supports the hypothesis that patients with ALF have a compromised cerebral oxidative metabolism, primarily of mitochondrial origin, leading to “cytopathic hypoxia” or “dysoxia” in the brain. Inhibitors of the ammonia-induced mitochondrial dysfunction are well characterized in vitro (e.g. antioxidants, magnesium, minocycline. l -histidine and cyclosporine) [14,26,27] , and recently an interventional study of an ALF animal model found a promising inhibitory effect of l -histidine on brain edema [28] . Continuing this work will be important in order to discover novel neuroprotective therapies. Funding The Rigshospitalet Research Foundation, University of Copenhagen, Denmark. Savvaerksejer Jeppe Juhl and Wife Ovita Juhls Foundation. Conflict of interest The authors who have taken part in this study declared that they do not have anything to disclose regarding funding or conflict of interest with respect to this manuscript. References [1] J.O. Clemmesen F.S. Larsen J. Kondrup B.A. Hansen P. Ott Cerebral herniation in patients with acute liver failure is correlated with arterial ammonia concentration Hepatology 29 1999 648 653 [2] W. Bernal C. Hall C.J. Karvellas G. Auzinger E. Sizer J. Wendon Arterial ammonia and clinical risk factors for encephalopathy and intracranial hypertension in acute liver failure Hepatology 46 2007 1844 1852 [3] A.T. Blei Brain edema in acute liver failure Crit Care Clin 24 2008 99 114 [ix] [4] C. Zwingmann The anaplerotic flux and ammonia detoxification in hepatic encephalopathy Metab Brain Dis 22 2007 235 249 [5] J. Albrecht M.D. Norenberg Glutamine: a Trojan horse in ammonia neurotoxicity Hepatology 44 2006 788 794 [6] A.R. Jayakumar K.V. Rao C. Murthy M.D. Norenberg Glutamine in the mechanism of ammonia-induced astrocyte swelling Neurochem Int 48 2006 623 628 [7] M.D. Norenberg A.R. Jayakumar K.V. Rama Rao K.S. Panickar New concepts in the mechanism of ammonia-induced astrocyte swelling Metab Brain Dis 22 2007 219 234 [8] F. Tofteng J. Hauerberg B.A. Hansen C.B. Pedersen L. Jorgensen F.S. Larsen Persistent arterial hyperammonemia increases the concentration of glutamine and alanine in the brain and correlates with intracranial pressure in patients with fulminant hepatic failure J Cereb Blood Flow Metab 26 2006 21 27 [9] P.N. Bjerring J. Hauerberg H.J. Frederiksen L. Jorgensen B.A. Hansen F. Tofteng Cerebral glutamine concentration and lactate–pyruvate ratio in patients with acute liver failure Neurocrit Care 9 2008 3 7 [10] E. Kosenko Y. Kaminsky E. Grau M.D. Minana G. Marcaida S. Grisolia Brain ATP depletion induced by acute ammonia intoxication in rats is mediated by activation of the NMDA receptor and Na+, K+-Atpase J Neurochem 63 1994 2172 2178 [11] Y. Kaminsky E. Kosenko Brain purine metabolism and xanthine dehydrogenase/oxidase conversion in hyperammonemia are under control of NMDA receptors and nitric oxide Brain Res 1294 2009 193 201 [12] P. Reinstrup N. Stahl P. Mellergard T. Uski U. Ungerstedt C.H. Nordstrom Intracerebral microdialysis in clinical practice: baseline values for chemical markers during wakefulness, anesthesia, and neurosurgery Neurosurgery 47 2000 701 709 [13] P. Bjerring M. Eefsen B. Hansen F. Larsen The brain in acute liver failure. A tortuous path from hyperammonemia to cerebral edema Metab Brain Dis 24 2009 5 14 [14] K.V. Rama Rao A.R. Jayakumar M.D. Norenberg Role of oxidative stress in the ammonia-induced mitochondrial permeability transition in cultured astrocytes Neurochem Int 47 2005 31 38 [15] M.D. Norenberg K.V. Rama Rao A.R. Jayakumar Ammonia neurotoxicity and the mitochondrial permeability transition J Bioenerg Biomembr 36 2004 303 307 [16] D.L. Shawcross S. Balata S.W. Olde Damink P.C. Hayes J. Wardlaw I. Marshall Low myo-inositol and high glutamine levels in brain are associated with neuropsychological deterioration after induced hyperammonemia Am J Physiol Gastrointest Liver Physiol 287 2004 G503 G509 [17] F. Lang Mechanisms and significance of cell volume regulation J Am Coll Nutr 26 2007 613S 623S [18] G. Wright D. Shawcross S.W. Olde Damink R. Jalan Brain cytokine flux in acute liver failure and its relationship with intracranial hypertension Metab Brain Dis 22 2007 375 388 [19] Pedersen HR, Ring-Larsen H, Larsen FS. Abstract 848: Cerebral hyperemia and intracranial hypertension induced by co-administration of endotoxin and ammonia in the rat are mediated by cyclooxygenase. Hepatology, 2007 ed., vol. 46, No. 4 (Suppl. 1); 2008. p. 613. [20] P. Ott O. Clemmesen F.S. Larsen Cerebral metabolic disturbances in the brain during acute liver failure: from hyperammonemia to energy failure and proteolysis Neurochem Int 47 2005 13 18 [21] M.J. Bell C.S. Robertson P.M. Kochanek J.C. Goodman S.P. Gopinath J.A. Carcillo Interstitial brain adenosine and xanthine increase during jugular venous oxygen desaturations in humans after traumatic brain injury Crit Care Med 29 2001 399 404 [22] T. Zetterstrom L. Vernet U. Ungerstedt U. Tossman B. Jonzon B.B. Fredholm Purine levels in the intact rat-brain – studies with an implanted perfused hollow fiber Neurosci Lett 29 1982 111 115 [23] K. Matsumoto R. Graf G. Rosner N. Shimada W.D. Heiss Flow thresholds for extracellular purine catabolite elevation in cat focal ischemia Brain Res 579 1992 309 314 [24] J.O. Skarphedinsson M. Sandberg H. Hagberg S. Carlsson P. Thoren Relative cerebral-ischemia in Shr due to hypotensive hemorrhage – cerebral function, blood-flow and extracellular levels of lactate and purine catabolites J Cereb Blood Flow Metab 9 1989 364 372 [25] N.J. Shah H. Neeb G. Kircheis P. Engels D. Haussinger K. Zilles Quantitative cerebral water content mapping in hepatic encephalopathy Neuroimage 41 2008 706 717 [26] K.V. Rama Rao M. Chen J.M. Simard M.D. Norenberg Suppression of ammonia-induced astrocyte swelling by cyclosporin A J Neurosci Res 74 2003 891 897 [27] P.V.B. Reddy K.V.R. Rao M.D. Norenberg Inhibitors of the mitochondrial permeability transition reduce ammonia-induced cell swelling in cultured astrocytes J Neurosci Res 87 2009 2677 2685 [28] K.V.R. Rao P.V.B. Reddy X.Y. Tong M.D. Norenberg Brain edema in acute liver failure inhibition by l -histidine Am J Pathol 176 2010 1400 1408
更多查看译文
关键词
ALF,ATP,CBF,CPP,ICH,ICP,LP,MAP
AI 理解论文
溯源树
样例
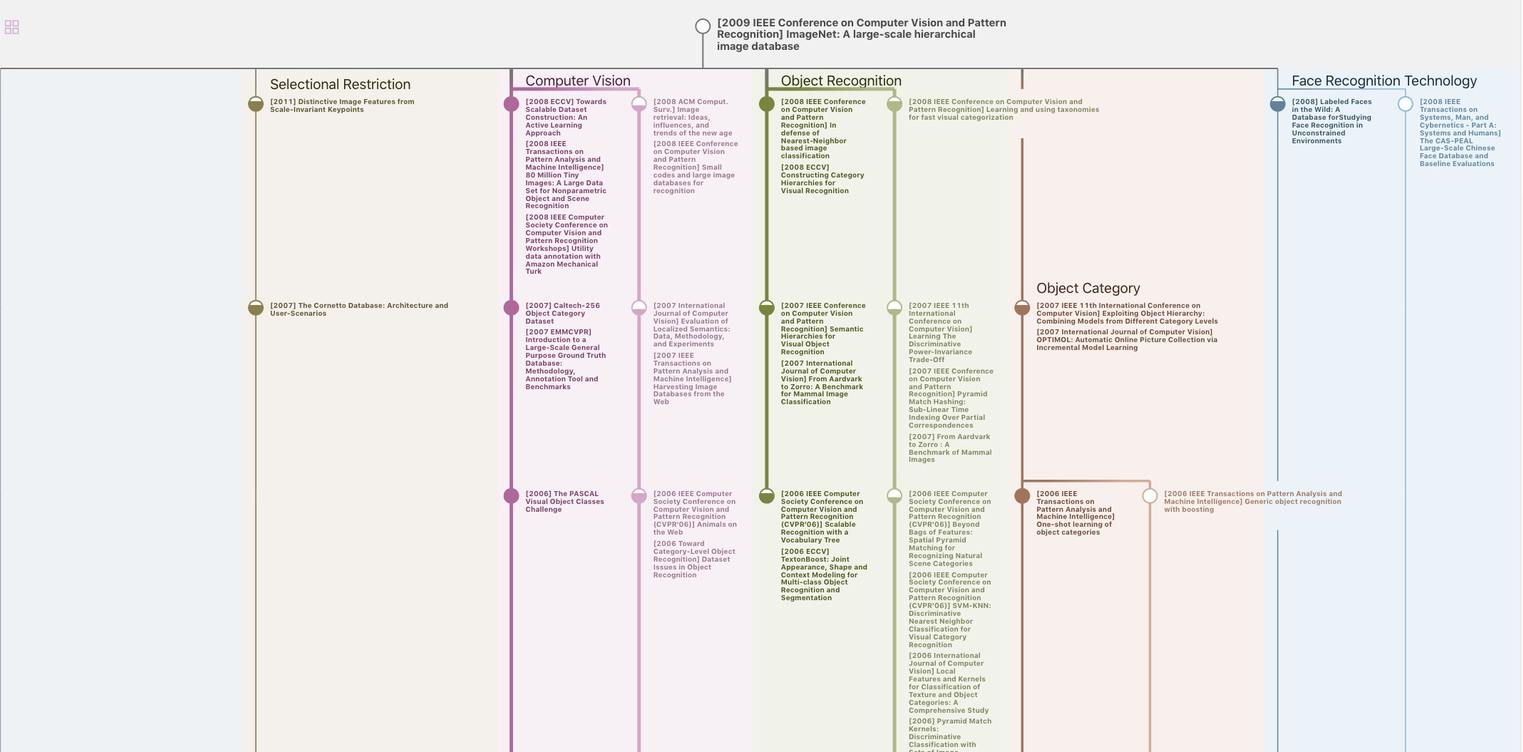
生成溯源树,研究论文发展脉络
Chat Paper
正在生成论文摘要