Liver and pancreatic fat content and metabolism in healthy monozygotic twins with discordant physical activity
Journal of Hepatology(2013)
摘要
Results Twins with higher physical fitness had 23% less liver fat (1.3 ± 1.3% vs. 2.1 ± 2.6%, p = 0.022) but no such difference was observed in the pancreatic fat (8.2 ± 9.3% vs. 9.8 ± 8.5%, respectively, p = 0.3). Hepatic fat content was inversely associated with VO 2max . A positive association was found between pancreatic and liver fat contents ( β = 5.18, p = 0.012). Pancreatic fat content was also associated with insulin sensitivity indexes and plasma adiponectin and glutamyltransferase concentrations. Conclusions Pancreatic fat content is associated with insulin resistance and hepatic fat content. An active lifestyle seems to beneficially influence hepatic fat metabolism. Keywords Magnetic resonance spectroscopy Physical activity Monozygotic twins Introduction Pancreatic lipid metabolism is currently of considerable interest. Previously, ectopic fat in myocardium and liver have been associated with insulin resistance. Recently, increased pancreatic fat has also been suggested to relate to impaired insulin secretion and type 2 diabetes. [1–4] It has been suggested that excess ectopic pancreatic fat disturbs β-cell function and decreases insulin secretion [4–7] . Pancreatic insulin secretion plays a central role in the pathology of type 2 diabetes. Decreased insulin secretion disturbs insulin signalling in the hypothalamus, thus causing increased appetite and obesity and leading to insulin resistance. In addition, it raises plasma glucose levels through impaired suppression of hepatic glucose production and decreased muscle glucose uptake. Finally, it also increases lipolysis in adipocytes, thus elevating plasma NEFA concentration, which further leads to insulin resistance and impaired β-cell function. It has been suggested that impaired β-cell function is present even years before type 2 diabetes is diagnosed [4] . As pancreatic fat accumulation has been suggested to be one mechanism leading to the impairment of insulin secretion, the early indication of pancreatic lipomatosis is of importance in the prevention of type 2 diabetes [1] . To date, there are very few studies which used MRI for pancreatic fat measurement in humans [5,8–11] , and the magnetic resonance spectroscopy ( 1 H MRS) method was used in only two of them [5,11] . Recently, pancreatic fat assessment was validated with 1 H MRS in rats and, in the same study, pancreatic fat was shown to be associated with weight and impaired glucose tolerance in humans [11] . Tushuizen and co-workers [5] measured pancreatic and hepatic fat content in men with and without type 2 diabetes. They showed that pancreatic fat is associated with β-cell function in nondiabetic subjects. To our knowledge, the effects of physical activity and fitness on pancreatic fat content have not been previously studied. Lipid accumulation in the liver impairs the insulin-induced suppression of hepatic glucose production, resulting in hyperglycaemia and insulin resistance [12,13] . In healthy subjects, visceral fat mass is suggested to be directly related to liver fat content [14] and is a better indicator than BMI or subcutaneous fat for liver fat accumulation [15] . Physical activity and exercise training decreases intra-abdominal fat mass [16–20] . However, studies investigating the effects of physical activity and exercise training on intrahepatic fat content show contradictory findings both in healthy lean [18,21,22] and obese humans [19–21,23] . In the present study, to minimize the effects of genetic factors, we investigated monozygotic twins with discordant physical activity and fitness (18% mean difference in VO 2max between twin brothers) and hypothesized that the brother with higher physical activity would have lower hepatic and pancreatic fat content compared to the less active brother. We also hypothesized that intra-abdominal fat masses measured by MRI would be associated with pancreatic fat content measured using 1 H MRS, indicating possible β-cell dysfunction. We have previously shown that increased physical activity decreases hepatic free fatty acid uptake in monozygotic twins who have discordant physical activity and fitness measured with positron emission tomography (PET) and [ 18 F]-fluoro-6-thia-heptadecanoic acid ([ 18 F] FTHA) [16] . Thus, we used this same study sample in the present study. Methods Ethical approval Human studies The study protocol was approved by the Ethics Committee of the Hospital District of Southwest Finland and the studies were conducted according to the principles of the Declaration of Helsinki. Prior to any clinical assessments, written informed consent was obtained after the purpose, nature, and potential risks of the study were carefully explained to the subjects. Animal studies The animals in this study, 10 Finnish landrace pigs and 5 minipigs, were obtained immediately upon their termination in a (non-related) second study focusing on cardiac imaging. In order to minimize the amount of animals used, the pig 1 H MRS measurements of pancreatic fat and pancreas biopsies in the current study were specifically planned to coincide with the termination time point of the animals in the other study. Therefore, no animals were sacrificed for the current study. On the study day, animals were premedicated with ketamine (34 mg/kg i.m.) and diazepam (10 mg i.v.). After administration of pancuronium (4 mg i.v.), the animals were incubated via tracheotomy. The animals were connected to a volume-triggered respirator and ventilated with room air at the frequency of 16 breaths per minute. The anaesthesia was maintained with continuous intravenous infusion of ketamine (0.27 mg/kg/min) and pancuronium (0.007 mg/kg/min). Animals were sacrificed by potassium chloride overdose. The protocol was reviewed and approved by the Ethics Committee for Animal Experiments of the University of Turku. Subjects Subjects consisted of eight monozygotic (MZ) twin pairs (16 healthy males, age 25.8 ± 1.8 years). The subjects were recruited from five consecutive twin birth cohorts from the Central Population Register of Finland, and are participating in the ongoing FinnTwin16 study. The subject selection process, inclusion criteria, subject details, and determination of zygosity are described elsewhere in more detail [24] . Two to eight weeks before the MRI and MRS measurements, physical activity was studied using a questionnaire by Baecke et al. [25] , and a maximal cycle ergometer test was performed to determine VO 2max [24] . According to the criteria of significant differences in physical activity and at least 9% difference in VO 2max, nine twin pairs were selected for the study. Magnetic resonance spectroscopy data were obtained from eight twin pairs. The co-twins with higher physical activity and VO 2max constituting the more active group were compared to the less active group with lower physical activity and VO 2max . The more active group had 18 ± 11% higher VO 2max compared to the less active group (51.2 ± 5.4 vs. 43.7 ± 7.1 ml min −1 kg −1 , p = 0.0002). According to the Baecke [25] questionnaire, the more active group had 4.0 ± 2.9 and the less active group had 1.7 ± 1.5 ( p = 0.003) conditioning exercise workouts per week. The average time per week spent on conditioning exercise was 229 ± 156 min in the more active group and 98 ± 71 min in the less active group ( p = 0.013). The time per week spent on other physical activities was on average 4.3 ± 2.1 in the more active and 4.4 ± 4.3 in the less active group ( p = 0.96). The average time per week spent on other physical activities was 144 ± 110 in the more and 157 ± 162 min in the less active group ( p = 0.77). More details about the physical activity habits of the study groups have been previously described elsewhere [24] . Animal studies Validation of pancreatic fat measurement by 1 H MRS Animal study design To validate 1 H MRS measurements of pancreatic fat in-vivo, a pig model was used. In total, 15 animals were used in the current study. From 10 pigs (average weight 31 kg, age 3–6 months) the pancreas was surgically removed within a few minutes after termination of the animal. To prevent the pancreatic enzymes from affecting pancreatic fat content, biopsies from the caput, corpus, and cauda were frozen in liquid nitrogen and sent for biochemical analysis (see below). The remaining five animals, mini-pigs (average weight 39.3 ± 2.7 kg), were subjected to a 60 min MRI and 1 H MRS immediately upon termination. After MR examination, the pancreas was surgically dissected, biopsies were taken of corresponding areas for the 1 H MRS examinations (see below), and biochemical analysis was performed. MRI and 1 H MRS measurements MRI of the abdominal area and pancreas were performed using a 1.5T system (Gyroscan Intera Nova Dual, Philips Medical Systems, Best, The Netherlands) with a 4-channel SENSE-body surface coil. T1-weighted axial fast field (dual) echo images were obtained, with a slice thickness of 3.5 mm for accurate localization of the pancreas. A single-voxel 1 H MRS examination was performed in the largest available anatomical region of the pancreas, avoiding visible vascular structures and pancreatic ducts as much as possible. The point resolved spectroscopy (PRESS) sequence used had the following parameters: Echo Time (TE) 27 ms, Time of Repetition (TR) 3000 ms. Biochemical lipid analysis Selectivity of triglyceride (TAG) fatty acid hydrolysis by pancreatic lipases was studied by determining the total fatty acid composition of samples using two different methylation methods. While the sodium methoxide method methylates only glycerolipids, the boron trifluoride method is also able to esterify free fatty acids. As there were no differences in the fatty acid composition between the two methods, it was determined that some fatty acids were not hydrolyzed in favour of others and that hydrolysis did not prevent TAG fatty acid composition analysis. However, selectivity of catabolism beyond fatty acid hydrolysis was not studied. Hydrolysis was kept at a minimum by immersing the samples in liquid nitrogen once removed from the body and stored at −70 °C. Frozen pieces of pancreas were homogenized in chloroform. Lipids were extracted with chloroform–methanol [26] , and TAGs separated with solid phase extraction as described [27] . Fatty acid methyl esters – prepared from the TAG fraction for fatty acid composition analysis [28] – were analyzed by gas chromatography (PerkinElmer AutoSystem, Norwalk, CT) with DB-23 column (60 m × 0.25 mm i.d., 0.25 μm film thickness (Agilent Technologies, Palo Alto, CA)). Triheptadecanoylglycerol was used as an internal standard. Calculation of pancreas triglyceride from 1 H MRS data Fat and water amplitudes were corrected for different T2 decay and molar concentrations of 1 H nuclei in fat and water. The T2 for pancreas water was obtained from T2 measurements and a value of 47.3 ms was used. The T2 for pancreas fat was assumed to be identical to liver fat and a value of 71 ms was used [29] . The results of the contribution of the individual fatty acids, as obtained by biochemical analysis ( Table 1 ) were used for the correction of differences in proton density between water and fat. Finally, pancreas fat content was expressed as fat in relation to the total weight of pancreas tissue, assuming a pancreas water content of 71% [29] . Correlation of 1 H MRS and biochemical measurements No significant difference between pancreas fat content measured using 1 H MRS or biochemical methods was observed (2.37% vs. 2.06%, p = 0.66) The correlation of the local pancreas fat content measurement using 1 H MRS and the result of biochemical analysis of corresponding tissue biopsies is shown in Fig. 1 . A good and significant agreement between the two methods was observed ( R 2 = 0.876, p = 0.019). Human studies Liver and pancreas fat measurements using 1 H MRS Patients were instructed to fast for a period of 2 h prior to the MR examination. A 1.5 T MR scanner (Signa Horizon LX, GE Medical Systems, Milwaukee, WI) was used, with a linear general-purpose-flex surface coil (for liver MRI and 1 H MRS) and an integrated body coil (for pancreas MRI and 1 H MRS). First, the upper abdomen, involving both the entire liver and pancreas, was imaged using a transverse T1 W dual-echo FSPGR (in-and-out-of-phase) sequence during breath-hold. The imaging parameters were: repetition time 150 ms, echo time 2.1 ms (out-of-phase) and 4.4 ms (in-phase), slice thickness 10 mm, matrix size 256 × 256, and acquisition time 23 s. Using the obtained images, a single voxel for spectroscopy was positioned in the right liver lobe, outside the area of the great vessels. The voxel for 1 H MRS of the pancreas was placed within the largest anatomical area of the organ. In both examinations, voxel dimensions were adjusted to avoid visible vascular structures and care was taken to account for the effect of the chemical shift artefact on actual voxel location. Voxel dimensions and location were recorded both graphically and numerically, allowing for identical voxel positioning within each twin pair. A PRESS 1 H MRS sequence (PROBE-SV) was used with the following parameters: TR = 3000 ms, TE = 25 ms, number of signals = 48 (liver) or 128 (pancreas). Liver 1 H MRS data were obtained during consecutive breath holds over a 2-min and 36-s period. During 1 H MRS of the pancreas, patients were instructed to breathe superficially and regularly during the 7-min 24-s data acquisition time. A typical location for the pancreas voxel is shown in the T1W MR-image in Fig. 2 . Calculation of final liver and pancreas triglyceride content by 1 H MRS Final liver fat content was calculated as previously described [30] . Final pancreas fat content was calculated in an identical way to the animal pancreas fat content (see above, animal studies), thus using the information of the TAG distribution in the pig pancreas (first 10 animals) for the correction of differences in proton density between pancreas fat and water. MRI of abdominal fat masses Abdominal subcutaneous, retroperitoneal, and visceral fat masses were determined using MRI. The MRI studies were performed on a 1.5 T MR imager (Signa Horizon LX, GE Medical Systems, USA) using a body coil. A single T1W FSE image was obtained at the level of the intervertebral disc, L2–L3, for analysis of abdominal adipose tissue masses as described previously [31] . To convert the measured volumes into weight, an adipose tissue density of 0.9196 g ml −1 was used. The MRI studies were performed on the same day for twin brothers. Positron emission tomography (PET) studies Tissue free fatty acid uptake was assessed using PET and [ 18 F]-labelled 6-thia-hepta-decanoic acid ([ 18 F] FTHA) in liver, myocardium, and skeletal muscles [16] and abdominal visceral, and subcutaneous tissues [32] as described previously. Glucose tolerance test One or two days after MRI studies, the subjects underwent a 2-h, 75-g oral glucose tolerance test (OGTT) after at least 12 h fasting. Blood samples were drawn at 0, 10, 20, 30, 60, 90, and 120 min to evaluate the degree of glucose tolerance and the β-cell response to the oral glucose load. Insulin response was calculated as the area under the plasma insulin concentration curve [33] . The homeostasis model assessment (HOMA) index was used as an inverse measure of insulin sensitivity, as described earlier [34] . The homeostasis model assessment of β-cell function (HOMA-B) [34] and insulinogenic index (insulin (mU/L) at 30 min during OGTT – fasting insulin (mU/L))/(glucose (mmol/min) at 30 min during OGTT-fasting glucose (mmol/min)) were applied to assess β-cell function. The Matsuda index was calculated as described earlier [35] . Biochemical analyses Plasma glucose was determined with the glucose oxidase method (Analox GM9 Analyzer, Analox Instruments Ltd., London, UK). Serum FFA and plasma lactate were both measured by the enzymatic colorimetric methods (ACS–ACOD method, Wako Chemicals USA, Inc., VA, USA for FFA, and Roche Diagnostics GmbH, Mannheim, Germany for lactate). Both methods were analyzed with the Roche Modular P800 automatic analyzer (Roche Diagnostics GmbH, Mannheim, Germany). Serum insulin was measured using an automated time-resolved immunofluorometric assay (AutoDELFIA, Wallac, Turku, Finland). Analysis of oxidized LDL was based on determination of the baseline level of conjugated dienes in LDL lipids [36,37] . The appearance of conjugated diene double bonds is characteristic of peroxidation of all polyunsaturated fatty acids, and in the in vitro and ex vivo studies on LDL oxidation, diene conjugation has been commonly used as the index of LDL oxidation. The assay procedure consisted of the following steps: (a) isolation of the lipoprotein fraction, (b) extraction of lipoprotein lipids, and (c) spectrophotometric analysis of conjugated dienes in the lipoprotein lipids. LDL was isolated by precipitation with buffered heparin. The isolation procedure was validated for the purpose and did not affect the level of oxidized lipids [37] . Lipids were extracted from isolated LDL by chloroform–methanol (2:1), dried under nitrogen, and redissolved in cyclohexane. The amount of peroxidized lipids in LDL was assessed spectrophotometrically at 234 nm. Validation studies for the assay have ruled out interference by nonspecific substances, and have shown that diene conjugation is a measure of oxidative LDL modification found in all LDL lipid classes. The coefficient of variation (CV) for the within-assay precision for determining oxidized LDL lipids was 4.4% and the CV for the between-assay precision was 4.5%. C-reactive protein (CRP) concentrations were analyzed using a Cobas Integra 700 automatic analyzer with reagents and calibrators as recommended by the manufacturer [Hoffmann-La Roche Ltd., Basel, Switzerland; COBAS® Integra C-Reactive Protein (Latex)]. A typical detection limit for CRP was 0.25 mg/L (0.025 mg/dl) [38] . Concentrations of plasminogen activator inhibitor 1 (PAI-1) were determined as described earlier [39] . Human leptin (Human Leptin Ria kit, Linco Research) and adiponectin (Human Adiponectin Ria kit, Linco research, St. Charles, USA) concentrations were measured with radioimmunoassay. Statistical analyses Statistical analyses were performed using the SAS/STAT statistical analysis program package, version 8.2 (SAS Institute Inc., Cary, NC, USA). Animal data Normality of variables was assessed by the Shapiro–Wilk test. Proportions of fatty acids in caput, corpus, and cauda were compared with either the ANOVA or Kruskall–Wallis test depending on the normality of the data. As there were no significant differences in TAG fatty acid composition between the different parts of the pancreas, the results of each pig were averaged. Human data Normality of variables was assessed by the Shapiro–Wilk test. Student’s paired t -test was used for both normally and non-normally distributed variables to determine whether there were differences between groups. Non-normally distributed variables underwent logarithmic transformation in order to obtain normality. Because the subjects were related (MZ twins), we were unable to calculate normal Pearson correlation values for the pooled population. Instead, associations between continuous parameters in the whole group (i.e. all individuals) were evaluated using a linear mixed model in which twin pair membership was used as a random effect. The resulting β-coefficient is equivalent to the regression slope of the relationship between the dependent and the independent variable. p values below 0.05 were considered statistically significant. All results are expressed as mean standard deviation (SD). Results Anthropometry and metabolic data The more active twins had less abdominal subcutaneous and intraperitoneal fat, although no statistically significant differences were observed in body weight, BMI, or body fat percentage between the groups ( Table 2 ). All subjects had normal blood insulin and FFA concentrations, plasma glucose levels at baseline, and also during the OGTT at the time point of 120 min. The more active twins had lower HOMA (1.8 ± 1.5 vs.1.6 ± 1.0) index and higher apolipoprotein A1 (1.26 ± 0.11 vs. 1.35 ± 0.18 g l −1 ) concentration compared to their less active brothers. Fat content in liver and pancreas Hepatic fat content was 23% lower in the more active twins ( Fig. 3 ). Hepatic fat content was negatively associated with VO 2max ( Fig. 4 ) and positively associated with several anthropometrical measurements and intra-abdominal fat masses ( Table 3 ). Hepatic fat was also positively associated with insulin sensitivity and resistance indexes, with plasminogen activator inhibitor-1, leptin, and glutamyltransferase concentration ( Table 4 ). A significant association was also found between hepatic fat content and HDL cholesterol ( β = −2.349, SE = 0.52, p = 0.003), oxidized LDL ( β = 0.041, SE = 0.012, p = 0.011) and apolipoprotein A1 ( β = −2.191, SE = 0.894, p = 0.044). Pancreatic fat content was not significantly different between the groups, and no association was observed between pancreatic fat content and VO 2max . Pancreatic fat content was significantly associated with hepatic fat content ( β = 5.18, p = 0.012), insulin sensitivity, and resistance indexes, and with adiponectin and glutamyltransferase concentrations ( Table 4 .). Pancreatic fat was not significantly associated with anthropometric measurements or blood lipid parameters. Association between tissue fat content and FFA uptake Pancreatic fat content was positively associated with hepatic ( β = 4.640, SE = 1.771, p = 0.034), myocardial ( β = 0.929, SE = 0.341, p = 0.030), and abdominal subcutaneous adipose tissue FFA uptake ( β = 24.223, SE = 9.425, p = 0.037). A trend was also observed with visceral adipose tissue FFA uptake ( β = 21.471, SE = 10.384, p = 0.078). No associations were observed between hepatic fat content and FFA uptake in different tissues. Discussion The results of the present study show that subjects with higher physical activity and fitness have lower hepatic fat content than less active and less fit individuals. Contrary to our hypothesis, physical fitness showed no effect on pancreatic fat content. Moreover, contrary to our hypothesis, pancreatic fat content showed no association with intra-abdominal fat masses. However, pancreatic fat content associated positively with hepatic fat content and glutamyltransferase level, and inversely with adiponectin level. Adiponectin and glutamyltransferase levels have previously been connected to hepatic fat accumulation and insulin resistance [40–42] . The hepatic lipid content was on average 23% lower in subjects with about 18% higher VO 2max . The present study also showed an inverse association between hepatic lipid content and VO 2max . These findings are in accordance with our earlier finding of lower hepatic FFA uptake in the more active twins [16] in this same study sample. In our previous study, we suggested that the more active twins have a lower lipolysis rate due to decreased adiposity and increased insulin-mediated suppression of adipose tissue lipolysis. Thus, we suggested that the lower lipolysis rate and probably increased gluconeogenesis in the more active twins would lead to metabolic shunting of glucose to the liver, thus reducing the hepatic FFA uptake compared to less active twins. This suggestion still stands although only a trend towards lower whole body fat percentage was observed in the twins with higher VO 2max . Previously, Perseghin and co-workers [22] demonstrated that lower hepatic fat content associates with a higher level of habitual physical activity, determined by the physical activity questionnaire. Also, habitual physical activity has been shown to prevent hepatic fat accumulation in a longitudinal population-based twin study. However, training studies in lean humans are sparse and have not shown changes in liver fat content [21] . In the study of Devries et al. [21] , liver fat content was measured after 12 weeks of endurance training in lean subjects using computed tomography (CT). The controversy in the results might be attributed to the low sensitivity of CT for detection of low levels of liver fat content [43] . In obese subjects, the results of exercise training without weight loss on liver fat content are also contradictory. In the studies of Heijden and co-workers [20] and Johnson and co-workers [20] , physical training decreased liver fat content, whereas no changes were observed in the studies of Shojaee-Moradie and co-workers [23] or Devries and co-workers [21] after the intervention period. However, the effects of physical training on hepatic lipid metabolism might be different in overweight and lean subjects due to the differences in lipid metabolism. The results of the current study confirm previous findings that a significant relationship exists between liver fat content and intra-abdominal fat mass [14,44] . It is important to emphasize that the selection of identical twins in our study minimizes the effects of different genetic backgrounds, representing a common confounder in cross-sectional studies. Therefore, the group differences shown herein can be attributed to the sole impact of lifestyle. In this study, a higher association was observed between liver fat content and intra- and retroperitoneal fat masses than with the abdominal subcutaneous fat mass. This is in agreement with the suggestion that liver fat content may be related to metabolic activity rather than only to the mass of different adipose tissue depots [44,45] . Currently, there are no published studies providing clear pathways regarding the effects of physical activity on liver fat content. Our previous findings show a reduced hepatic FFA uptake in the group with higher physical activity, and a significant correlation between the decrease in FFA uptake and liver fat content in obese individuals undergoing weight loss [46] . The widely published data indicate that liver fat content is a reliable (most likely indirect) marker of whole body insulin sensitivity. Altogether, the above evidence suggests that the FFA load to the liver, likely regulated by adipose tissue lipolysis, and its suppression by insulin, plays a major role in the differences observed between our study groups. However, further studies are needed to elucidate which metabolic markers are the key players in this process. Our results of pancreatic fat content are comparable with the previous reports in healthy subjects [5,11] . The main difference of the current study with the previous studies is that we evaluated 1 H MRS for measurement of pancreatic fat in pigs with the organ still in situ , thus more closely resembling the challenges of 1 H MRS in humans. Ectopic pancreatic fat in humans has been shown to increase with age, weight, and BMI, in different pathological conditions measured in vitro and using Dixon MR imaging [8,9,47,48] . In the present study, higher physical activity and fitness did not significantly affect the pancreatic fat content, and no association was found between pancreatic fat content and VO 2max . This suggests that pancreatic fat is probably not as sensitive to changes in physical activity as other tissues, such as the liver. On the other hand, the subjects in our study were healthy and relatively fit, and it remains to be studied whether exercise training could restore pancreatic fat content in obese patients. We also hypothesised that pancreatic fat content would be correlated with intra-abdominal fat masses. However, only slight trends were observed with intra- and retroperitoneal fat masses. On the other hand, pancreatic fat associated significantly with hepatic fat content. Further, our results are in agreement with previous findings that the fat content is higher in the pancreas than in the liver, measured using Dixon MR imaging [8] . We also studied the association between pancreatic fat content and FFA uptake in different tissues, measured using PET and [ 18 F] FTHA in our earlier studies with the same subjects [16,32] . Pancreatic fat content associated positively with hepatic, myocardial, and abdominal subcutaneous adipose tissue FFA uptake. A trend was also observed between pancreatic fat content and visceral adipose tissue FFA uptake. Unfortunately, pancreatic FFA uptake was not measured in our previous study. No association was found between hepatic fat content and hepatic, myocardial, or adipose tissue FFA uptake, though group comparisons had shown that hepatic FFA uptake was lower in the more fit group [16] . The weakness in the correlation of individual data could be due to the fact that FFA uptake was measured under fasting conditions, whereas fat accumulation may occur predominantly in the post-prandial period. However, more targeted studies are needed to evaluate the mechanisms or possible confounding factors in the relationship of physical activity and liver and pancreas fat content. Ectopic fat accumulation in muscle and liver is related to insulin resistance [1–4] , and it has also been suggested that lipid accumulation in the pancreas impairs the insulin secretion of the β-cells in the islets of Langerhans [3] . Recently, Tushuizen and co-workers [5] showed a negative correlation between pancreatic fat and β-cell function parameters in healthy obese subjects. Such correlation appeared to be in great part driven by individuals with a pronounced insulin resistance. We could not find a significant correlation between the insulinogenic index and pancreatic fat content, which may be due to the lack of severe insulin resistance in our subjects. Our present results confirm an association between hepatic and pancreatic fat contents and insulin sensitivity and resistance parameters. The positive correlation of HOMA-B and pancreatic fat content may be explained by the strong influence of insulin levels over this indirect index of beta cell function. In fact, normalization for insulin resistance eliminated the significance. In the current study, pancreatic fat content associated negatively with adiponectin and positively with gammaglutamyltransferase levels, which have previously been connected to hepatic and visceral fat, insulin sensitivity and type 2 diabetes [21,41,49–51] . To our knowledge, the association of pancreatic fat content with adiponectin and gammaglutamyltransferase has not been reported previously. We conclude that pancreatic fat correlates with hepatic fat content and insulin resistance. In healthy males, hepatic fat content seems to be lower in more physically active subjects as compared to less active subjects. However, further studies with larger patient populations are needed to elucidate the effects and mechanisms of physical fitness on pancreatic and liver fat content. Conflict of interest The authors who have taken part in this study declared that they do not have anything to disclose regarding funding or conflict of interest with respect to this manuscript. Acknowledgements The authors wish to thank the personnel of the Turku PET Centre for their assistance during the study. The study was conducted within the Finnish Centre of Excellence in Molecular Imaging in Cardiovascular and Metabolic Research supported by the Academy of Finland, the University of Turku, Turku University Hospital, and Åbo Academy University. The study was financially supported by the Academy of Finland ( 206970 and 204240 ), the Academy of Finland Centre of Excellence in Complex Disease Genetics, the Ministry of Education ( 143/722/2002 , 51/722/2003 , and 40/627/2005 ), the Juho Vainio Foundation, the Turku University Foundation, the Finnish Cultural Foundation, the South Western Finland Cultural Foundation, the Finnish Sports Institute Foundation, the Finnish Sports Research Foundation, and the HEPADIP (LSHM-CT-2005-018734). The FinnTwin16 study has been supported by the National Institute on Alcohol Abuse and Alcoholism (AA08315 & AA12502), the Academy of Finland (44069 & 100499) and the European Union Fifth Framework Program (QLG2-CT-2002-01254). References [1] N.A. van Herpen V.B. Schrauwen-Hinderling Lipid accumulation in non-adipose tissue and lipotoxicity Physiol Behav 94 2008 231 241 [2] J. Szendroedi M. Roden Ectopic lipids and organ function Curr Opin Lipidol 20 2009 50 56 [3] K.E. Pinnick S.C. Collins C. Londos D. Gauguier A. Clark B.A. Fielding Pancreatic ectopic fat is characterized by adipocyte infiltration and altered lipid composition Obesity (Silver Spring) 16 2008 522 530 [4] S.E. Kahn R.L. Hull K.M. Utzschneider Mechanisms linking obesity to insulin resistance and type 2 diabetes Nature 444 2006 840 846 [5] M.E. Tushuizen M.C. Bunck P.J. Pouwels S. Bontemps J.H. van Waesberghe R.K. Schindhelm Pancreatic fat content and beta-cell function in men with and without type 2 diabetes Diabetes Care 30 2007 2916 2921 [6] Y. Lee H. Hirose M. Ohneda J.H. Johnson J.D. McGarry R.H. Unger Beta-cell lipotoxicity in the pathogenesis of non-insulin-dependent diabetes mellitus of obese rats: impairment in adipocyte-beta-cell relationships Proc Natl Acad Sci USA 91 1994 10878 10882 [7] J. Matsui Y. Terauchi N. Kubota I. Takamoto K. Eto T. Yamashita Pioglitazone reduces islet triglyceride content and restores impaired glucose-stimulated insulin secretion in heterozygous peroxisome proliferator-activated receptor-gamma-deficient mice on a high-fat diet Diabetes 53 2004 2844 2854 [8] A. Kovanlikaya S.D. Mittelman A. Ward M.E. Geffner F. Dorey V. Gilsanz Obesity and fat quantification in lean tissues using three-point Dixon MR imaging Pediatr Radiol 35 2005 601 607 [9] H. Raeder I.S. Haldorsen L. Ersland R. Gruner T. Taxt O. Sovik Pancreatic lipomatosis is a structural marker in nondiabetic children with mutations in carboxyl-ester lipase Diabetes 56 2007 444 449 [10] N.F. Schwenzer J. Machann P. Martirosian N. Stefan C. Schraml A. Fritsche Quantification of pancreatic lipomatosis and liver steatosis by MRI: comparison of in/opposed-phase and spectral-spatial excitation techniques Invest Radiol 43 2008 330 337 [11] I. Lingvay V. Esser J.L. Legendre A.L. Price K.M. Wertz B. Adams-Huet Noninvasive quantification of pancreatic fat in humans J Clin Endocrinol Metab 94 2009 4070 4076 [12] A. Kotronen A. Seppälä-Lindroos R. Bergholm H. Yki-Järvinen Tissue specificity of insulin resistance in humans: fat in the liver rather than muscle is associated with features of the metabolic syndrome Diabetologia 51 2008 130 138 [13] A. Kotronen J. Westerbacka R. Bergholm K.H. Pietiläinen H. Yki-Järvinen Liver fat in the metabolic syndrome J Clin Endocrinol Metab 92 2007 3490 3497 [14] C. Thamer J. Machann M. Haap N. Stefan E. Heller B. Schnodt Intrahepatic lipids are predicted by visceral adipose tissue mass in healthy subjects Diabetes Care 27 2004 2726 2729 [15] D.E. Kelley T.M. McKolanis R.A. Hegazi L.H. Kuller S.C. Kalhan Fatty liver in type 2 diabetes mellitus: relation to regional adiposity, fatty acids, and insulin resistance Am J Physiol Endocrinol Metab 285 2003 E906 E916 [16] J.C. Hannukainen P. Nuutila B. Ronald J. Kaprio U.M. Kujala T. Janatuinen Increased physical activity decreases hepatic free fatty acid uptake: a study in human monozygotic twins J Physiol 578 2007 347 358 [17] B.A. Irving C.K. Davis D.W. Brock J.Y. Weltman D. Swift E.J. Barrett Effect of exercise training intensity on abdominal visceral fat and body composition Med Sci Sports Exerc 40 2008 1863 1872 [18] T. Leskinen S. Sipilä M. Alen S. Cheng K.H. Pietiläinen J.P. Usenius Leisure-time physical activity and high-risk fat: a longitudinal population-based twin study Int J Obes (Lond) 33 2009 1211 1218 [19] N.A. Johnson T. Sachinwalla D.W. Walton K. Smith A. Armstrong M.W. Thompson Aerobic exercise training reduces hepatic and visceral lipids in obese individuals without weight loss Hepatology 50 2009 1105 1112 [20] G.J. van der Heijden Z.J. Wang Z.D. Chu P.J. Sauer M.W. Haymond L.M. Rodriguez A 12-Week Aerobic Exercise Program Reduces Hepatic Fat Accumulation and Insulin Resistance in Obese, Hispanic Adolescents Obesity (Silver Spring) 18 2010 384 390 [21] M.C. Devries I.A. Samjoo M.J. Hamadeh M.A. Tarnopolsky Effect of endurance exercise on hepatic lipid content, enzymes, and adiposity in men and women Obesity (Silver Spring) 16 2008 2281 2288 [22] G. Perseghin G. Lattuada F. De Cobelli F. Ragogna G. Ntali A. Esposito Habitual physical activity is associated with intrahepatic fat content in humans Diabetes Care 30 2007 683 688 [23] F. Shojaee-Moradie K.C. Baynes C. Pentecost J.D. Bell E.L. Thomas N.C. Jackson Exercise training reduces fatty acid availability and improves the insulin sensitivity of glucose metabolism Diabetologia 50 2007 404 413 [24] J.C. Hannukainen U.M. Kujala J. Toikka O.J. Heinonen J. Kapanen T. Vahlberg Cardiac structure and function in monozygotic twin pairs discordant for physical fitness J Appl Physiol 99 2005 535 541 [25] J.A. Baecke J. Burema J.E. Frijters A short questionnaire for the measurement of habitual physical activity in epidemiological studies Am J Clin Nutr 5 1982 936 942 [26] J. Folch M. Lees G.H.S. Stanley A simple method for the isolation and purification of total lipides from animal tissues J Biol Chem 226 1957 497 509 [27] J.G. Hamilton K. Comai Rapid separation of neutral lipids, free fatty-acids and polar lipids using prepacked silica sep-pak columns Lipids 23 1988 1146 1149 [28] W.W. Christie A simple procedure for rapid transmethylation of glycerolipids and cholesteryl esters Journal of Lipid Research 23 1982 1072 1075 [29] C. Thomsen U. Becker K. Winkler P. Christoffersen M. Jensen O. Henriksen Quantification of liver fat using magnetic-resonance spectroscopy Magn Reson Imag 12 1994 487 495 [30] R.J. Borra S. Salo K. Dean R. Lautamäki P. Nuutila M. Komu Nonalcoholic fatty liver disease: rapid evaluation of liver fat content with in-phase and out-of-phase MR imaging Radiology 250 2009 130 136 [31] N. Abate A. Garg R. Coleman S.M. Grundy R.M. Peshock Prediction of total subcutaneous abdominal, intraperitoneal, and retroperitoneal adipose tissue masses in men by a single axial magnetic resonance imaging slice Am J Clin Nutr 65 1997 403 408 [32] J.C. Hannukainen K.K. Kalliokoski R.J. Borra A.P. Viljanen T. Janatuinen U.M. Kujala Higher Free Fatty Acid Uptake in Visceral Than in Abdominal Subcutaneous Fat Tissue in Men Obesity (Silver Spring) 18 2010 261 265 [33] I. Vauhkonen L. Niskanen E. Vanninen S. Kainulainen M. Uusitupa M. Laakso Defects in insulin secretion and insulin action in non-insulin-dependent diabetes mellitus are inherited – metabolic studies on offspring of diabetic probands Journal of Clinical Investigation 101 1998 86 96 [34] D.R. Matthews J.P. Hosker A.S. Rudenski B.A. Naylor D.F. Treacher R.C. Turner Homeostasis model assessment: insulin resistance and beta-cell function from fasting plasma glucose and insulin concentrations in man Diabetologia 28 1985 412 419 [35] M. Matsuda R.A. DeFronzo Insulin sensitivity indices obtained from oral glucose tolerance testing – Comparison with the euglycemic insulin clamp Diabetes Care 22 1999 1462 1470 [36] M. Ahotupa M. Ruutu E. Mäntylä Simple methods of quantifying oxidation products and antioxidant potential of low density lipoproteins Clin Biochem 29 1996 139 144 [37] M. Ahotupa J. Marniemi T. Lehtimäki K. Talvinen O.T. Raitakari T. Vasankari Baseline diene conjugation in LDL lipids as a direct measure of in vivo LDL oxidation Clin Biochem 31 1998 257 261 [38] S. Eda J. Kaufmann W. Roos S. Pohl Development of a new microparticle-enhanced turbidimetric assay for C-reactive protein with superior features in analytical sensitivity and dynamic range J Clin Lab Anal 12 1998 137 144 [39] R. Lautamäki T. Rönnemaa R. Huupponen T. Lehtimäki P. Iozzo K.E. Airaksinen Low serum adiponectin is associated with high circulating oxidized low-density lipoprotein in patients with type 2 diabetes mellitus and coronary artery disease Metabolism 56 2007 881 886 [40] D.H. Kim A.P. Burgess M. Li P.L. Tsenovoy F. Addabbo J.A. McClung Heme oxygenase-mediated increases in adiponectin decrease fat content and inflammatory cytokines tumor necrosis factor-alpha and interleukin-6 in Zucker rats and reduce adipogenesis in human mesenchymal stem cells J Pharmacol Exp Ther 325 2008 833 840 [41] M. Bajaj S. Suraamornkul P. Piper L.J. Hardies L. Glass E. Cersosimo Decreased plasma adiponectin concentrations are closely related to hepatic fat content and hepatic insulin resistance in pioglitazone-treated type 2 diabetic patients J Clin Endocrinol Metab 89 2004 200 206 [42] I.J. Perry S.G. Wannamethee A.G. Shaper Prospective study of serum gamma-glutamyltransferase and risk of NIDDM Diabetes Care 21 1998 732 737 [43] P. Charatcharoenwitthaya K.D. Lindor Role of radiologic modalities in the management of non-alcoholic steatohepatitis Clin Liver Dis 11 2007 37 54 [44] E.L. Thomas G. Hamilton N. Patel R. O’Dwyer C.J. Dore R.D. Goldin Hepatic triglyceride content and its relation to body adiposity: a magnetic resonance imaging and proton magnetic resonance spectroscopy study Gut 54 2005 122 127 [45] J.G. Kral F. Schaffner R.N. Pierson Jr. J. Wang Body fat topography as an independent predictor of fatty liver Metabolism 42 1993 548 551 [46] A.P. Viljanen P. Iozzo R. Borra M. Kankaanpaa A. Karmi R. Lautamaki Effect of weight loss on liver free fatty acid uptake and hepatic insulin resistance J Clin Endocrinol Metab 94 2009 50 55 [47] P. Schmitz-Moormann P.M. Pittner W. Heinze Lipomatosis of the pancreas. A morphometrical investigation Pathol Res Pract 173 1981 45 53 [48] T.S. Olsen Lipomatosis of the pancreas in autopsy material and its relation to age and overweight Acta Pathol Microbiol Scand A 86A 1978 367 373 [49] G. Perseghin G. Lattuada F. De Cobelli A. Esposito E. Belloni T. Canu Serum retinol-binding protein-4, leptin, and adiponectin concentrations are related to ectopic fat accumulation J Clin Endocrinol Metab 92 2007 4883 4888 [50] C. Koebnick C.K. Roberts G.Q. Shaibi L.A. Kelly C.J. Lane C.M. Toledo-Corral Adiponectin and leptin are independently associated with insulin sensitivity, but not with insulin secretion or beta-cell function in overweight Hispanic adolescents Horm Metab Res 40 2008 708 712 [51] E. Bugianesi U. Pagotto R. Manini E. Vanni A. Gastaldelli R. de Iasio Plasma adiponectin in nonalcoholic fatty liver is related to hepatic insulin resistance and hepatic fat content, not to liver disease severity J Clin Endocrinol Metab 90 2005 3498 3504
更多查看译文
关键词
Magnetic resonance spectroscopy,Physical activity,Monozygotic twins
AI 理解论文
溯源树
样例
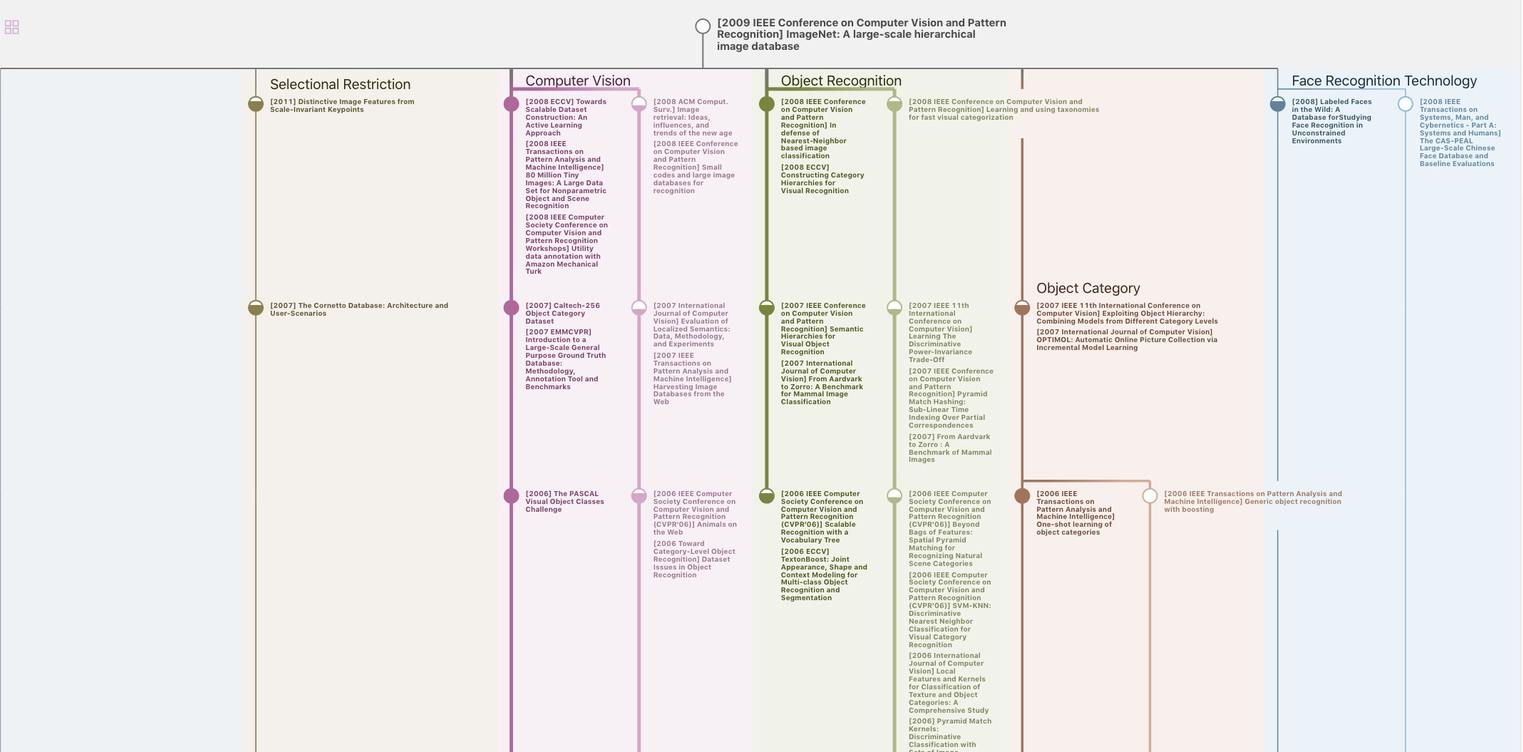
生成溯源树,研究论文发展脉络
Chat Paper
正在生成论文摘要