Plateau and Transpulmonary Pressure With Elevated Intra-Abdominal Pressure or Atelectasis
Journal of Surgical Research(2010)
摘要
Methods In Yorkshire pigs, esophageal pressure (Pes) was measured with a balloon catheter as a surrogate for Ppl under two experimental conditions: (1) high IAP group ( n = 5), where IAP was elevated by CO 2 insufflation in 5mm Hg steps from 0 to 30mm Hg; and (2) Atelectasis group ( n = 5), where a double lumen endotracheal tube allowed clamping and degassing of either lung by O 2 absorption. Lung collapse was estimated by increases in pulmonary shunt fraction. Results High IAP: Sequential increments in IAP caused a linear increase in Pplat (r 2 = 0.754, P < 0.0001). Ptp did not increase (r 2 = 0.014, P = 0.404) with IAP due to the concomitant increase in Pes (r 2 = 0.726, P < 0.0001). Partial Lung Collapse: There was no significant difference in Pplat between the atelectatic (21.83 ± 0.63cm H 2 O) and inflated lung (22.06 ± 0.61 cmH 2 O, P < 0.05). Partial lung collapse caused a significant decrease in Pes (11.32 ± 1.11mm Hg) compared with inflation (15.89 ± 0.72mm Hg, P < 0.05) resulting in a significant increase in Ptp (inflated = 5.97 ± 0.72mm Hg; collapsed = 10.55 ± 1.53mm Hg, P < 0.05). Conclusions Use of Pplat to set ventilation may under-ventilate patients with intra-abdominal hypertension and over-distend the lungs of patients with atelectasis. Thus, Ptp must be used to accurately set mechanical ventilation in the critically ill. Key Words transpulmonary pressure intra-abdominal pressure atelectasis ventilator induced lung injury Introduction Severe trauma, hemorrhage, and sepsis in critically ill patients can result in systemic inflammation [1, 2] which, if untreated, can lead to multiple organ dysfunction syndrome (MODS) [3–5] . One of the first organs to fail as a result of this inflammation is the lung, with the development of acute lung injury and, most severely, the acute respiratory distress syndrome (ARDS) [6] . Currently, the only treatment for ARDS is supportive mechanical ventilation [7] ; however, improperly implemented mechanical ventilation can increase ARDS-induced morbidity and mortality in the form of ventilator induced lung injury (VILI) [8] . To avert this outcome, the landmark study by the ARDS Network (ARDSnet) demonstrated that low tidal volume (Vt) and limits on airway plateau pressures (Pplat) can significantly reduce VILI-induced mortality in ARDS patients [9] . One shortcoming of this paradigm, however, is that the direct determinant of lung over-distension and VILI is the transpulmonary pressure (Ptp), which is defined as the plateau pressure minus the pleural pressure (Pplat – Ppl) [10, 11] . It follows therefore that Ptp can show evidence of pronounced lung distension not revealed by routine Vt and Pplat monitoring. It is well known that intra-abdominal hypertension (IAH) is a serious complication common in critically ill patients [12, 13] that may significantly increase morbidity and mortality [14] . Abdominal compartment syndrome (ACS) is the pathologic consequence of IAH-induced end-organ dysfunction [15] . The lung is subject to the negative impact of IAH [16] , as high intra-abdominal pressure compresses the thoracic cavity, significantly reducing chest wall compliance (Ccw) and increasing pleural pressure. Due to this elevated pleural pressure, Ptp in patients with ACS may be much lower for any given Pplat and, consequently, the patient may be under-ventilated. This poses a problem for the clinician because using Ptp values to guide mechanical ventilation could result in a Pplat considered unsafe by ARDSnet standards ( > 30 cmH 2 O) when the lung, in fact, was being ventilated safely. Lung collapse secondary to surfactant deactivation is also a common occurrence in the critically ill patient with ARDS [17] . Lung collapse increases the negative intrathoracic pressure causing a decrease in pleural pressure [18] , which may lead to an increase in Ptp for a given Pplat. Under these circumstances, VILI could occur at a Pplat considered to be safe by ARDSnet standards ( < 30 cm H 2 O) [9] . Without knowledge of Ptp, the patient with lung collapse cannot be optimally ventilated. The goal of this study was to assess the use of a clinically available esophageal balloon catheter to determine Ptp, using the esophageal pressure (Pes) as a surrogate for pleural pressure. We hypothesized that changes in Pes with increased intra-abdominal pressure (IAP) and atelectasis would result in distending pressures that were not reflected by Pplat. Real-time awareness of Ptp alerts clinicians of the need to reduce or prevent VILI under circumstances that are not included in the protective vigilance offered by ARDSnet standards. Materials and Methods Juvenile male Yorkshire pigs ( n = 10) weighing 35–45 kg were pre-anesthetized with ketamine (20 mg/kg, IM) and xylazine (2 mg/kg, IM) 10–15 min before intubation. Propofol (10 mg/mL at a rate of 25–90 mL/h depending on need) was delivered intravenously (IV) via a Gemini Volumetric Infusion Pump (model PC-2TX; Imed Corp., San Diego, CA) for continuous anesthesia. The animals were ventilated with an AVEA ventilator (Viasys Health Care, Yorba Linda, CA) with 21% (Group 1) or 100% (Group 2) oxygen. Baseline ventilator settings were tidal volume 8 mL/kg, PEEP 5 cmH 2 O, and respiratory rate 15 breaths/min. Respiratory rate was adjusted to maintain arterial carbon dioxide (PaCO 2 ) between 35 and 45 cm H 2 O. Esophageal pressure (Pes) was measured with a Bicore monitor connected directly with the AVEA and a nasogastric esophageal balloon catheter (SmartCath Nasogastric Pressure Monitoring Tug Set; Viasys Health Care, Palm Springs, CA) positioned by pressure tracing (i.e., obvious cardiac inflections during expiration and a parallel rise and fall in Pes with airway pressure) as described by Talmor et al. [11] . A left carotid arteriotomy was performed and a PiCCO Pulsiocath 5 French catheter was inserted for blood gas measurements (Gem Premier-3000; Instrumentation Laboratory Inc., Lexington, MA), systemic arterial blood pressure monitoring, and calculation of continuous cardiac output and extra vascular lung water (EVLW) (Pulsion Medical Inc., East Brunswick, NJ). A double lumen catheter (MAC two-lumen central venous access 7 or 8 French; Arrow International, Reading, PA) with Swan-Ganz inducer port was placed into the right internal jugular vein for fluid, anesthesia, and drug infusion. A seven French flow-directed Swan-Ganz thermodilution catheter (Edwards Life Science, LLC, Irvine CA) was passed through the inducer into the pulmonary artery for mixed venous blood gas and O 2 content sampling along with continuous cardiac output (CO) determinations (Vigilance; Edwards Life Science, LLC, Irvine, CA). Blood pressures were measured using transducers (open heart monitoring kit number 46068-48; Hospira Inc, Lake Forest, IL) leveled with the right atrium and recorded on a Hewlett-Packard monitor (model 56, Palo Alto, CA) with pressure (model M-1006BT), oxygen saturation (model M-1029A), and ECG (model M-1002A) amplifiers. An incision was made in the right groin and the bladder was accessed in a retroperitoneal fashion to prevent leakage of CO 2 during insufflation. A small incision was made in the bladder and a Foley catheter with an inline pressure port (infection control 350 mL urine meter Foley tray; Bardex I. C. Complete Care; C. R. Bard Inc., Covington GA) was inserted and connected to a pressure transducer leveled to the mid-axillary line on the animal and to a Hewlett-Packard monitor (model 56) and amplifier (model M-1006BT) for measurement of bladder pressure. A Veress needle (Stryker Endoscopy, Inc.) was inserted midline in the abdomen (Group I only) and connected to a CO 2 insufflator (16 L flow insufflator; Stryker Endoscopy, Inc.). The insufflator injected CO 2 at a volume sufficient to maintain the preset pressure (0–30 in 5 mm Hg increments) constant. Protocol Group I (High IAP, n = 5) The animals were placed on the AVEA ventilator with 21% O 2 . Following instrumentation, baseline hemodynamics [arterial (MAP), pulmonary (PAP), pulmonary artery wedge (PAW), and central venous (CVP) pressures (mm Hg)], lung function [mean (Pmean), peak (Ppeak), and plateau (Pplat) pressures; esophageal pressure (Pes) at Pplat (PesPlat) and PEEP (PesPEEP), and the difference between these two pressures (dPes); Transpulmonary (Ptp) pressures (cmH 2 O) at both Pplat (PtpPplat) and PEEP (PtpPEEP); static (Cstat), dynamic (Cdyn) and chest wall (Ccw) compliance, (mL/cmH 2 O)], blood gas (pH, PO 2 , PCO 2 ), and bladder (Pbladder, mm Hg) pressures were recorded. Pbladder was used as a surrogate for intra-abdominal pressure (IAP) and measured by injecting 20 mL of saline into the bladder via the Foley catheter. We allowed a 1-min stabilization period before recording the measurement. The IAP was increased in 5 mm Hg increments with the insufflator ( Fig. 1 ). As soon as the IAP stabilized (1-3 min) at the preset value, 10 additional min were given for all pressures to stabilize and all measurements, pressures and blood gases were again recorded. This process was repeated until an IAP of 30 mm Hg was obtained ( Fig. 1 ). Following the above measurements, the CO 2 was allowed to escape from the abdomen and IAP was reduced to 0 mm Hg while the Ccw also returned to normal. Vt was then increased until the Pplat was identical to that caused by 30 mm Hg of IAP and all pressures were again measured ( Fig. 1 ). Vt was reduced to baseline and PEEP was increased until the Pplat was identical that caused by 30 mm Hg of IAP and all parameters were measured ( Fig. 1 ). Group 2 (Atelectasis, n = 5) The animals were mechanically ventilated on the AVEA ventilator with 100% O 2 and surgically prepared as in Group 1 animals, except that a double lumen endotracheal tube (Tyco Healthcare, Pleasanton, CA) was inserted through a tracheostomy. To confirm proper placement of the endotracheal tube both lumens of the tube were separately occluded and breath sounds were confirmed in the contralateral lung fields but absent in the ipslateral fields. Baseline measurements were recorded (see above) and then one lung was randomly chosen and the endotracheal tube lumen to that lung was clamped and the lung allowed to degas. The Vt was reduced to the remaining lung in an attempt to keep Pplat at baseline levels. After a 10-min stabilization period, all lung function measurements were recorded, and a blood sample was drawn from the carotid and pulmonary artery for pulmonary shunt calculation. Pulmonary shunt was calculated with the following eqs: Pa O 2 = Fi O 2 ( 760 − 47 ) − 1.2 ( PaC O 2 ) Cn O 2 = ( 1.34 × Hgb × Sn O 2 / 100 ) + ( 0.003 × Pa O 2 ) Qs / Qt = ( Cc O 2 − Ca O 2 ) / Cc O 2 − Cv O 2 ) (PaO 2 = arterial partial pressure of oxygen; FiO 2 is the fraction of inspired oxygen; PaCO 2 = partial pressure of carbon dioxide; CnO 2 is the content of oxygen, n = venous, capillary, or arterial blood; Hgb = hemoglobin; SnO 2 = saturation of oxygen; and Qs/Qt = pulmonary shunt fraction). After all measurements were recorded the clamp was removed and the collapsed lung reinflated. After a 15-min stabilization period, the opposite lung was clamped and degassed according to the same protocol. This procedure was repeated six times (i.e., the right and left lungs were each degassed three times) before the experiment was terminated. All animals from both groups were euthanized at the completion of the studies by a pentobarbital overdose (150 mg/kg). Statistics Linear regressions with goodness of fit (r 2 ) with P value were performed to analyze relationships between parameters. A one-way ANOVA with a Newman-Keuls multiple comparison test was used to assess significance in Group I. An unpaired t -test was used to compare parameters between the normal and atelectatic lung in Group II using GraphPad Prism, GraphPad Software, San Diego, CA, www.graphpad.com . Significance was assumed when the probability of the null hypothesis being true was less than 5% ( P < 0.05). All values are reported as mean ± SEM. Vertebrate Animals Experiments described in this study were performed in adherence with the National Institutes of Health guidelines for the use of experimental animals in research. The Institutional Animal Care and Use Committee at Orlando Regional Medical Center approved the protocol. Results Group I The changes in all lung function parameters with increasing IAP are presented in Table 1 . Rising IAP caused an increase in PesPlat that balanced out the rise in Pplat without a significant increase in PtpPplat ( Table 1 ). PesPEEP and PtpPEEP were not affected by rising IAP ( Table 1 ). Peritoneal pressure (IAP) was accurately increased and maintained with the insufflator, and Pbladder was tightly correlated with IAP ( Fig. 2 ). As expected, increasing IAP caused an increase in Pplat with a concomitant decrease in Ccw ( Fig. 3 ). More importantly, increasing IAP correlated strongly with PesPlat, which prevented a concurrent rise in PtpPlat ( Fig. 4 ). The dynamic component of Pes was highly correlated with changing IAP whereas the static was not ( Fig. 5 ). We also compared the impact of increasing Pplat by first increasing Vt and then PEEP until a level of Pplat was obtained similar to that at IAP 30 mm Hg. This was done with an IAP of 0 mm Hg so that Pes and Ccw were normal. Increasing Pplat with normal Pes and Ccw caused a significant increase in Ptp compared with the same Pplat with high Pes and low Ccw ( Fig. 6 ). Group II The amount of collapsed lung was estimated by the increase in pulmonary shunt fraction caused by lung clamping. Lung degassing caused a significant decrease in the Pes and a resulting increase in the PtpPplat at the same Pplat without a change in Ccw ( Fig. 7 A-D ). However, the amount of collapse (% shunt) did not correlate with the change in Pes or PtpPlat ( Fig. 8 A and B). Discussion It has been shown in a Phase III clinical trial that low tidal volume and plateau pressure can reduce mortality in ARDS patients [9] . However, such ventilation strategy is based on the premise that both chest wall compliance and pleural pressures are normal. The pressure that distends the lung is not simply the plateau pressure, rather, it is the plateau pressure minus the pleural (Ppl) pressure, and this is termed transpulmonary pressure (Ptp). Patients that develop MODS with ALI/ARDS are likely to also develop IAH or ACS, which may significantly increase the Ppl and reduce Ptp at any given Pplat. It seems logical, therefore, to manage the ventilator strategy of ARDS patients using Ptp rather than Pplat. This notion has been recently tested clinically in ARDS and acute lung injury patients, showing significantly improved oxygenation and compliance when Pes was used to estimate Ptp, compared with patients treated with the current standard of care (ARDSnet protocol) [11] . Our study demonstrated that the measurement of esophageal pressure with clinically available equipment accurately determined Ptp and allowed the identification of the known physiologic relationships between Pplat, Ccw, Ppl, and Ptp. Rising IAP causes the push of abdominal contents against the diaphragm, manifested as a rise in Pplat and a fall in Ccw. Increasing IAP also increased esophageal pressure (the surrogate measurement of Ppl), however, transpulmonary pressure did not correlate with increasing IAP. This is noteworthy because as Pplat rose, so did Ppl and, thus, Ptp did not increase significantly. PtpPEEP was negative throughout the study, consistent with a recent clinical study [11] and represents lung collapse secondary to insufficient PEEP. It is important to note that several factors seen clinically can augment the changes seen in this study. Abdominal ascites is less distensible than the gas used, which may lead to larger elevations in IAP and, thus, Pplat and Ptp. Body wall edema can decrease the compliance of the thorax and abdomen, also causing larger elevations in pressure. Another concept that was supported by esophageal pressure monitoring was the difference between dynamic and static Pes [19] . It has recently been shown that differentiating between static and dynamic components of esophageal pressure may be important to clinical assessment of patients with ACS [19] . Valenza et al. defined static Pes as Pes at PEEP (PesPEEP) and dynamic Pes as the difference between Pes at end-expiratory and end-inspiratory pressure (dPes) [19] . They demonstrated that the dynamic component of esophageal pressure (dPes) was highly correlated with IAP, and that PesPEEP was not [19] . Our data support these findings, as we demonstrated that the significant increase in dPes was highly correlated with increasing IAP, whereas there was no significant change in PesPEEP or correlation with the increasing IAP ( Fig. 5 ). In the second Phase of Group I, the Vt and PEEP were individually increased to a similar Pplat as that obtained at an IAP of 30. This phase of the study was designed to ascertain if the Ptp would change as the Pplat changed in the presence of normal Ccw and Ppl. We found that at a Pplat slightly lower than that generated with high IAP ( Fig. 6 ), elevating Vt or PEEP in the presence of normal Ccw significantly increased Ptp. The significance of this observation is that Pplat is a poor indicator of lung distension in patients with a stiff chest wall. Our focus on esophageal pressure allowed us to follow established physiologic tenets of lung inflation and demonstrate the importance of chest wall compliance in lung distension. This study addresses questions of essence to proper ventilation in the critically ill patient. Malbrain et al. [12] observed that intra-abdominal hypertension is common among critically ill patients [12] , which suggests that the pressure driving lung distension is being inaccurately measured in many patients in the ICU. In the ALVEOLI trial, there was no significant clinical benefit from increased PEEP in patients with ARDS [20] , even though many other studies have shown that PEEP is protective [21, 22] . It is possible that the negative results from the ALVEOLI study were due to application of PEEP without regard to chest wall compliance and transpulmonary pressure. Knowledge of the patient's pleural pressure allows accurate calculation of the transpulmonary pressure, which would avoid both over- and under-distension of the lung. Avoiding over-distension would reduce VILI caused by volutrauma, and avoiding under-distension would lead to better oxygenation and ventilation of the patient. Although the literature describing the impact of atelectasis on pleural pressure is scant, it seems intuitive that lung collapse should cause a decrease in Ppl by increasing the negative pressure in the chest, and in this study we have demonstrated this to be true. The reduction in Ppl effected an increase in PtpPplat at the same Pplat, suggesting that PtpPplat values provide an accurate measurement of the lung distending pressure in the presence of significant atelectasis. Surfactant deactivation resulting in atelectasis is a hallmark of ARDS. Since patients with ARDS are at high risk for secondary VILI, the possibility exists that plateau pressure may not be a good measure of the lung distending pressure and, notably, that volutrauma may occur at lung pressures considered safe [9] . The amount of lung collapse did not closely correlate with the PesPlat or the PtpPlat. This could be due to the limitations of using % shunt as a parameter to represent amount of collapse. Physiologic mechanisms exist to prevent blood from flowing through atelectatic and non-ventilated regions of the lung; alveolar hypoxia resulting from atelectasis causes pulmonary vasoconstriction preventing ventilation-perfusion mismatching and arterial hypoxia [23] . This vasoconstriction occurs to varying levels in individual animals, resulting in wide range of intrapulmonary shunting. The % shunt could therefore inaccurately estimate the amount of lung collapse. It is known, though, that larger areas of collapse cause a less effective vascular response, resulting in more intrapulmonary shunting [23] . In conclusion, we were able to successfully determine real-time esophageal pressure during ventilatory maneuvers in a large animal model using clinically available equipment. Our focus on physiologic relationships between IAP, Pplat, Ccw, Pes, and Ptp using esophageal pressure catheters demonstrated their value in assuring safe ventilation in the critical care patient. This study also demonstrated that using Pplat to estimate the distending pressure of the lung is highly inaccurate in the presence of a stiff chest wall or with atelectasis. From this we can infer that esophageal pressure should be routinely measured in critically ill patients at risk for IAH or atelectasis to prevent either over- or under-distension of the lung while attempting protective mechanical ventilation. Acknowledgments The authors thank Edward Sivak, M.D. for his comments and criticisms of this manuscript. This study was funded in part by a grant from Viasys Healthcare Inc. References 1 N. Matsuda Y. Hattori Systemic inflammatory response syndrome (SIRS): Molecular pathophysiology and gene therapy J Pharmacol Sci 101 2006 189 2 F. Jaimes J. Garces J. Cuervo The systemic inflammatory response syndrome (SIRS) to identify infected patients in the emergency room Intensive Care Med 29 2003 1368 3 A.E. Baue MOF, MODS, and Sirs: What is in a name or an acronym? Shock 26 2006 438 4 A.E. Baue Sepsis, systemic inflammatory response syndrome, multiple organ dysfunction syndrome, and multiple organ failure: Are trauma surgeons lumpers or splitters? J Trauma 55 2003 997 5 A.E. Baue Sepsis, multi-organ dysfunction syndrome (MODS) and multiple organ failure (MOF). Prevention is better than treatment Minerva Anestesiol 65 1999 477 discussion 481 6 S. Fujishima H. Morisaki A. Ishizaka Neutrophil elastase and systemic inflammatory response syndrome in the initiation and development of acute lung injury among critically ill patients Biomed Pharmacother 62 2008 333 7 O. Gajic F. Frutos-Vivar A. Esteban Ventilator settings as a risk factor for acute respiratory distress syndrome in mechanically ventilated patients Intensive Care Med 31 2005 922 8 L.N. Tremblay A.S. Slutsky Ventilator-induced lung injury: From the bench to the bedside Intensive Care Med 32 2006 24 9 A.R.D.S. Network Ventilation with lower tidal volumes as compared with traditional tidal volumes for acute lung injury and the acute respiratory distress syndrome N Engl J Med 342 2000 1301 10 D.R. Hess L.M. Bigatello The chest wall in acute lung injury/acute respiratory distress syndrome Curr Opin Crit Care 14 2008 94 11 D. Talmor T. Sarge A. Malhotra Mechanical ventilation guided by esophageal pressure in acute lung injury N Engl J Med 359 2008 2095 12 M.L. Malbrain D. Chiumello P. Pelosi Prevalence of intra-abdominal hypertension in critically ill patients: A multicenter epidemiological study Intensive Care Med 30 2004 822 13 E.J. Kimball M.D. Rollins M.C. Mone Survey of intensive care physicians on the recognition and management of intra-abdominal hypertension and abdominal compartment syndrome Crit Care Med 34 2006 2340 14 R. Peralta H. Hojman Abdominal compartment syndrome Int Anesthesiol Clin 39 2001 75 15 M.L. Malbrain M.L. Cheatham A. Kirkpatrick Results from the International Conference of Experts on Intra-abdominal Hypertension and Abdominal Compartment Syndrome. I. Definitions Intensive Care Med 32 2006 1722 16 P. Pelosi M. Quintel M.L. Malbrain Effect of intra-abdominal pressure on respiratory mechanics Acta Clin Belg 1(Suppl 1) 2007 S78 17 P.E. Cogo G.M. Toffolo C. Ori Surfactant disaturated-phosphatidylcholine kinetics in acute respiratory distress syndrome by stable isotopes and a two compartment model Respir Res 8 2007 13 18 G.T. Ford C.A. Bradley N.R. Anthonisen Forces involved in lobar atelectasis in intact dogs J Appl Physiol 48 1980 29 19 F. Valenza G. Chevallard G.A. Porro Static and dynamic components of esophageal and central venous pressure during intra-abdominal hypertension Crit Care Med 35 2007 1575 20 R.G. Brower P.N. Lanken N. MacIntyre Higher versus lower positive end-expiratory pressures in patients with the acute respiratory distress syndrome N Engl J Med 351 2004 327 21 M.B. Amato C.S. Barbas D.M. Medeiros Effect of a protective-ventilation strategy on mortality in the acute respiratory distress syndrome N Engl J Med 338 1998 347 22 V.M. Ranieri P.M. Suter C. Tortorella Effect of mechanical ventilation on inflammatory mediators in patients with acute respiratory distress syndrome: A randomized controlled trial JAMA 282 1999 54 23 D.G. Peroni A.L. Boner Atelectasis: Mechanisms, diagnosis and management Paediatr Respir Rev 1 2000 274
更多查看译文
关键词
transpulmonary pressure,intra-abdominal pressure,atelectasis,ventilator induced lung injury
AI 理解论文
溯源树
样例
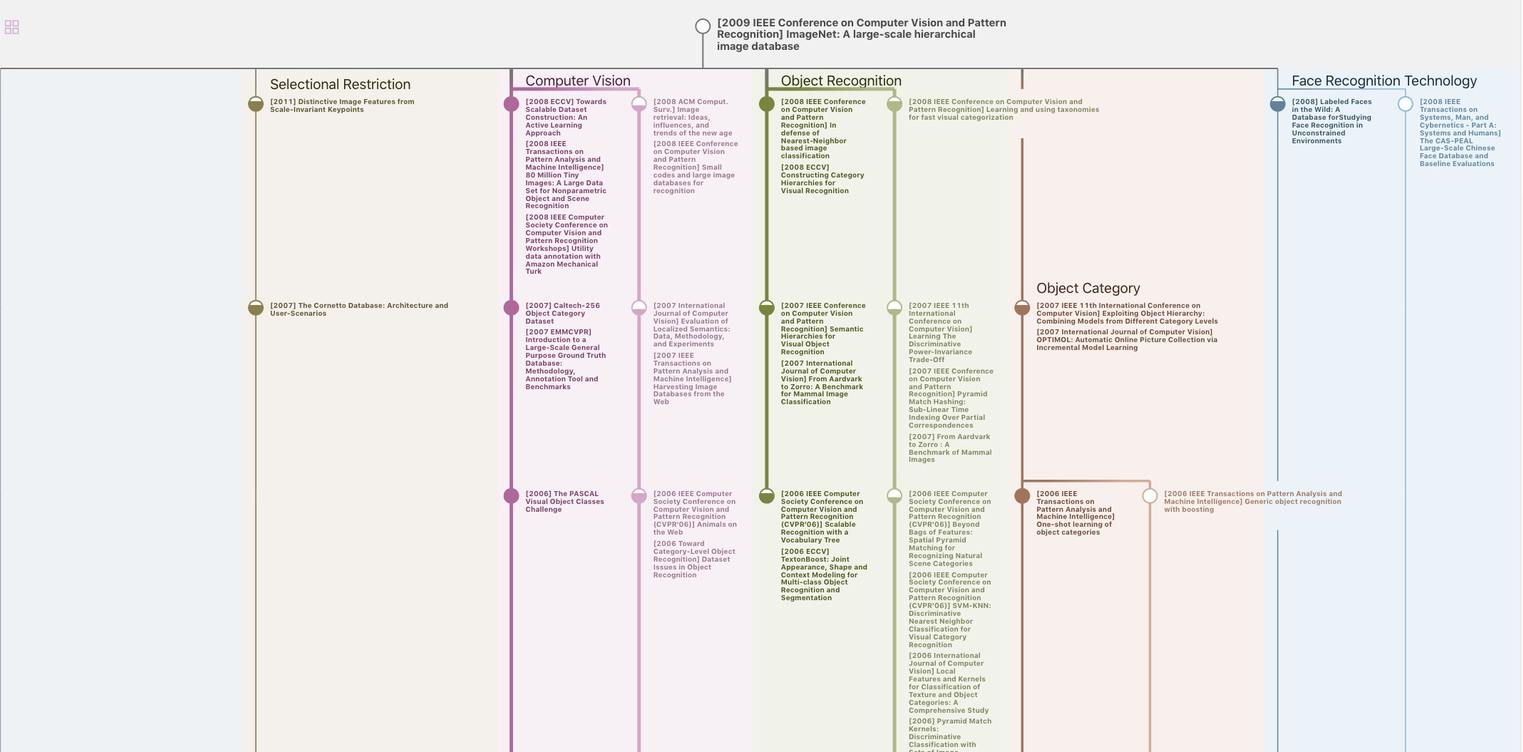
生成溯源树,研究论文发展脉络
Chat Paper
正在生成论文摘要