Comparing the effects of Dextran 70 and Hydroxyethyl starch in an intestinal storage solution
Cryobiology(2010)
摘要
Introduction Our lab has developed a novel strategy for intestinal preservation involving the intraluminal delivery of a nutrient-rich preservation solution. The aim of this study was to compare the effectiveness of two impermeant agents for use in our solution: Dextran 70 (D70; Mw = 70 kDa) and Hydroxyethyl starch (HES; Mw = 2200 kDa). Methods Rat intestines were procured, including an intravascular flush with University of Wisconsin solution followed by a ‘backtable’ intraluminal flush with: UW solution (group 1, UW), or an amino acid-based nutrient-rich preservation solution (AA solution) containing either 5% D70 (group 2, AA-D70) or HES (group 3, AA-HES). Tissue samples ( n = 6) were taken at 2, 4, 8, and 12 h cold storage; histology, energetic, end-product, and oxidative parameters were assessed. In separate groups ( n = 4), D70 and HES were fluorescently labeled with fluorescein isothiocyanate (FITC) in order to directly observe mucosal penetration of the starch and dextran. Results Over the 12 h storage time-course, direct visualization of the fluorescently labeled D70 showed penetration of the mucosal layer as early as 2 h and progressively continued to do so throughout the 12 h period. In contrast, HES did not cross the mucosal barrier and remained captive within the lumen. As time of storage progressed, grade of injury increased in all groups, however, at 4 and 12 h the AA-HES treated tissues exhibited significantly less injury compared to UW and AA-D70, P < 0.05. AA-HES group showed on moderate villus clefting (median grade 2; P < 0.05) while the AA-D70 group exhibited complete villus denudation (grade 4) and the UW group had extensive injury into the regenerative cryptal regions (grade 6). Metabolic parameters revealed a preferential maintenance of ATP and Energy Charge; increases in lactate, alanine and ammonium supported the involvement of aerobic and anaerobic pathways for energy production. Conclusion The results of this study challenge the idea that oncotic support is not a fundamental requirement of static organ storage. Furthermore, our data suggests that HES is an effective oncotic agent for use in our intraluminal nutrient-rich preservation solution, while Dextran 70 is not. Keywords Organ preservation solution Intestinal-specific Osmotic/oncotic agents Mucosal permeability Introduction Intestinal failure is the inability to maintain nutrition and fluid balance resulting in malnutrition and dehydration. Massive small bowel resection leading to short gut syndrome is the most common cause of intestinal failure [5,8] . Surgical intervention is required due to a multitude of diseases, conditions, or functional disorders [11] . The small bowel has a large reserve, however, but if more than 75% is resected, nutrient-absorption is limited and leads to a starvation-like state. Treatment modalities focus on providing nutrients, enterally or parenterally to promote intestinal ‘adaptation’ with the hopes that the patient’s gut will grow in order to return to self-sustaining function [8,33] . However, some patients do not adapt sufficiently and remain indefinitely on intravenous nutrition until complications of line infection and loss of venous access lead to patient morbidity and eventually death. The only option for these patients is intestinal transplantation [5,8,37,4,17,2] . Small bowel transplants have increased in frequency and success. In 2003, 140 transplant procedures were performed, compared to just 11 in 1990 [11] ; currently ∼200 intestinal transplants are performed worldwide yearly. For those patients receiving antibody-based pretreatment therapy and tacrolimus-based immunosuppressants, one year post-transplant survival rates have matched those of the liver (>80%). However, long-term survival rates (5 years) continue to remain low, although there is much variability depending on type of immunosuppressive protocol [11] . A major threat to successful intestinal transplantation is sepsis, accounting for 46% of mortality [11] . Systemic sepsis is the result of bacterial translocation following the disruption of intestinal barrier function, a consequence of inadequate preservation [15,1] . The University of Wisconsin (UW) solution has been recognized as one of the ‘gold standards’ for the preservation of intra-abdominal organs. But despite its efficacy with other intra-abdominal organs, simple solutions (such as 0.9% saline) has shown equivalent experimental outcomes [32,14] . Indeed, no one preservation solution has consistently proved superior over any other for intestinal storage [26,13,36] . Recognizing the urgent need for a more effective intestinal-specific preservation solution, our laboratory has been developing an amino acid-based nutrient-rich solution tailored to the metabolic requirements of the small intestine. The use of our novel preservation solution is an integral part of a strategy of intraluminal administration following a common intra-aortic flush with UW solution during multi-organ procurement. Our lab has published a number of in vitro and in vivo studies demonstrating improved metabolic parameters, tissue morphology, and functional characteristics following cold storage with this solution [26,9,20] . Although the composition of both the UW and our nutrient solution differs in many respects, each contains osmotically active molecules for controlling hypothermia-induced edema. Over the past several years, as we have been developing our nutrient-rich intraluminal preservation solution; either dextran (70 kDa) or hydroxyethyl starch has been included for the purpose of providing oncotic support [9,20,26–30] . However, molecular weight characteristics of an appropriate agent have not been established. Due to the stoppage of blood supply during cold storage, gradual deterioration of barrier function and absorptive properties of the mucosal epithelium occur. Tight junctions between epithelial cells require ATP to maintain their function, but ATP is rapidly consumed, and not produced at an effective rate during cold storage, so these protein structures begin to dilate. As the intestinal barrier function becomes compromised, some seemingly ‘high’ molecular weight compounds may be able to penetrate the mucosa to an appreciable degree. Without a true impermeant agent, the intraluminal solution cannot exert an osmotic force needed to offset tissue edema, resulting in deleterious physical injury. This physical stress will further exacerbate metabolic dysfunction initiated by the ischemic conditions of organ storage. The current study was designed to clarify issues of increased mucosal permeability during cold, ischemic storage by comparing the effects of Dextran 70 and hydroxyethyl starch in a nutrient-rich intraluminal preservation solution. Materials and methods Male Sprague–Dawley rats (200–300 g) were obtained from the University of Alberta and used as bowel donors. All experiments were conducted in accordance with the regulations and policies of the Canadian Council on Animal Care. Chemical agents were AR-grade and were purchased from Sigma Chemical (Oakville, Canada). Dextran (D70) and hydroxyethyl starch (HES) had average molecular weights of 70 and 2200 kDa, respectively. Dextran (catalogue No. D4751) was purchased from Sigma; HES was purchased from Aldrich #465143, 25,000 cps and purified as described previously to produce a purer molecular weight fraction [28] . Stability of fluorescent label and starches FITC labeling dextrans The procedure used to FITC-label D70, HES and the starch in UW solution (UW-HES) was based on the original procedure used by De Belder and Granath [6] . The molecule of interest, along with fluorescein 5-isothiocyanate (FITC), was dissolved in dimethyl sulphoxide (DMSO), pyridine, and dibutyltin dilaurate and heated to 95 °C for 2 h. The mixture was then put on ice and D70, HES or UW-HES was precipitated out with cold 100% ethanol, filtered, and dried. Evaluating stability of the fluorescent label on dextran and HES Fluorescence units per mg values for FITC-D70 and FITC-HES were determined to establish purity and efficiency of the labeling process. A mass of 10–15 mg was dissolved in Tris buffer (50 mM; pH 8.0). Appropriate dilutions were implemented and fluorescence was measured at Gain 60 on the Perkin Elmer HTS 7000 using the same excitation wavelengths as described above. Labeled D70 and HES solutions were incubated at room temperature (22 ± 2 °C) for 24 h. Samples were precipitated with cold 100% ethanol, centrifuged, diluted in Tris buffer and fluorescence measured. Evaluating the stability of the starches following the labeling procedure For HES, D70 and UW-HES, a series of molecular weight fractions were determined using centrifugal filters (Microcon Centrifugal Devices) with varying molecular weight cutoffs. A 100 mg/L solution of labeled starch and a 0.2 g/L solution of unlabeled starch were spun through 100, 50, 30, and 10 kDa MWCO filters. The filtrates of the starches were analyzed using an anthrone-based starch assay. Sample was added to anthrone and sulfuric acid, heated for 3 min at 90–95 °C, cooled on ice, plated and analyzed spectrophotometrically at 600 nm. The filtrate measured with each of the MWCOs was compared to an unfiltered sample and the percentage of starch present was determined. Permeability of bowel to D70 and HES during cold storage Surgical procedure and procurement of the small intestine All experiments were conducted in accordance with Canadian Council on Animal Care policies. Rats were fasted approximately 10–12 h. Rats were anesthetized with inhalational isoflurane (0.5–2%) in oxygen followed by an intramuscular injection of pentobarbital (65 mg/250 g; IM). A midline incision was made exposing the aorta at the level of the celiac trunk. The supraceliac aorta was clamped and 2–4 mL of University of Wisconsin (UW) solution was administered through the infrarenal aorta. The suprahepatic vena cava was transected to allow the outflow of blood and UW solution. Following the intravascular flush, experimental groups were designated according to the following intraluminal treatment: • UW: UW solution • AA-D70: nutrient solution with D70 • AA-HES: nutrient solution with HES Measurement of intraluminal Dextran 70 or HES during cold storage Separate groups of organs ( n = 4) were flushed (with 40 ml) and stored (with 30 ml) in AA-D70 and AA-HES; at the respective time points, a sample of intraluminal solution was extracted via a 23 ga needle and analyzed for starch (as glucose) [18] . Since the solutions contained other sugars (glucose and lactobionate), it was necessary to prepare a calibration curve using a series of standards of known carbohydrates for use in the final calculation of starch contents ( Fig. 1 ). Organ procurement procedure Following intravascular and intraluminal flushes, the small bowel was harvested and 16 cm long sections of ileum were measured out. The sections were filled at the proximal end with 4–5 mL nutrient-rich preservation solution (termed the ‘AA’ solution due to a large component of amino acids) containing either FITC-labeled D70 or HES (10×10 6 fluorescence units/mL) and each end was ligated with 3–0 silk. The preservation solution contained (values in brackets are mM): Glutamine (35), Lactobionate (20), Glutamate (20), Aspartate (20), Glucose (20), BES [N,N-Bis(2-hydroxyethyl)taurine] (15), Arginine (10), Glycine (10), Asparagine (10), Threonine (10), Lysine (10), Valine (10), Serine (10), Methionine (5), Leucine (5), Isoleucine (5), Histidine (5), Ornithine (5), Proline (5), Adenosine (5), Cysteine (5), β-Hydroxybutyrate (3), Tyrosine (1), Tryptophan (1), Trolox (1), 3-Aminobenzamide (1), Allopurinol (1); plus the inclusion of 5% Dextran 70 or HES. Solutions containing the respective starch were abbreviated AA-D70 or AA-HES. A control group using the University of Wisconsin (UW) solution as the intraluminal flush was included. Tissues were stored on ice in a fridge (4 °C) for a maximum of 12 h. At various times throughout a 12 h time course, short segments of bowel (2 cm) were removed from the distal end; the remaining segment of bowel was ligated to prevent escape of solution during the rest of the storage period. At the respective time point, the segment to be sampled was tied off while remaining in preservation solution. This ensured that equivalent amounts of tissue and luminal solution were removed and that the rest of the bowel remained undisturbed. A segment was fixed in alcoholic formalin for fluorescence and light microscopy at 2 (fluorescence only), 4, 8 and 12 h. Alcohol was necessary in the formalin to precipitate the starches and to maintain localization of the FITC label. Another segment at 4, 8, and 12 h was immediately frozen in nitrogen and subsequently stored at −65 °C until processing for metabolite analysis. A separate group of freshly isolated tissue ( t = 0) was analyzed for comparison to approximate in vivo levels of metabolites. For fluorescence assessment, n = 4; for metabolite analysis and H&E histology, n = 6. Histology Bowel samples were fixed in alcoholic formalin solution, processed to paraffin wax, embedded, and sections cut 4–5 μm thick. Slides were dewaxed up to 70% alcohol then mounted with Prolong Gold anti-fade reagent with DAPI (Invitrogen) and sealed with nail polish. Fluorescent microscopy was used to view FITC and DAPI fluorescence in tissue. Fluorescent images were digitally captured using AxioVision software. Ischemic injury was assessed using Park’s grading system [22] with modification as indicated in Table 1 . Sample preparation and metabolite assay Frozen samples were extracted 1:5 w/v in perchloric acid containing 1 mM EDTA. Immediately after homogenization in PCA, a 50 μl aliquot was taken and transferred to 0.15 mM NaOH (950 μl) for solubilization prior to protein measurement for standardization of metabolite levels [16] . The remainder of the acid homogenate was then centrifuged (20 min, 20,000× g ) to remove precipitated protein. Acid extracts were neutralized with 3 M KOH/0.4 M Tris/0.3 M KCl and recentrifuged (20 min, 14,000× g ). Neutralized extracts were immediately processed via standard enzyme-linked metabolite assays [23] . Spectrophotometric analysis was performed to measure the absorbance of NADH at 340 nm, providing quantification of ATP, ADP and AMP. ATP values were reported as μmol per gram protein and Energy Charge (EC) values were calculated as: EC = (ATP + 0.5ADP)/(ATP + ADP + AMP). Malondialdehyde was assessed from frozen tissue homogenized 1:10 in phosphate-buffered saline. The homogenate was then processed and fluorescence was compared to standard amounts of MDA [21] . Statistical analysis Data were reported as mean ± SE for each group. Statistical differences between groups were determined using Analysis of Variance (ANOVA), followed by SNK post hoc test; P < 0.05 was reported. Histology grades were compared with a non-parametric Kruskal–Wallis test. Results Intraluminal levels of dextran and hydroxyethyl starch throughout cold storage ( Fig. 1 ) Following intraluminal flush with AA-dextran, levels of dextran (70 kDa) contained within the lumen remained at values not significantly different than 100% of initial throughout the first 2 h storage. However, by 4 h values dropped to 86 ± 4% ( P < 0.05) and remained low and constant throughout the remainder of the 12 h time course ( P < 0.05). In contrast, levels of hydroxyethyl starch remained at values not significantly different than 100% initial. Stability of the fluorescent label and starches Stability of labeled D70 and HES The FITC label on both D70 and HES was stable; greater than 98.8% remained bound after 24 h at 22 °C. Stability of the FITC label on the HES isolated from UW solution (UW-HES) was ∼55%, with 45% of the FITC label liberating from the starch (data not shown). Molecular weight cutoff filters ( Fig. 2 ) This analysis was necessary to ensure the stability of D70 and HES for subsequent experimentation and to ensure validity of the interpretation of our results. According to the manufacturer monograft, the size of the molecules filtered is approximately 2 times greater than the filter specification (i.e. the true molecular weight exclusion for a 100 kDa filter is ∼200 kDa). Hence, discussion in this section will use filter cutoff values. Initially, we wanted to compare D70 with UW-HES, however, stability following the FITC-labeling procedure was extremely poor, indicating a considerable degradation (ranging from 24% to 50%) of the starch itself when exposed to heat ( P < 0.05 across all filter sizes). Due to the high instability of the UW-HES, it was excluded from any further experimentation. In contrast, both D70 and HES were much more stable as reflected in non-significant variations across the filter ranges. Permeability to D70 and HES during cold ischemic storage ( Fig. 3 ) During storage, D70 penetrated the tissue as early as 2 h and continued to do so throughout the 12 h storage period. Evidence of tissue damage throughout the time course was apparent. After 2 h, small amounts of epithelial lifting were observed. By 12 h, tissues showed substantial damage; epithelial lifting was complete, and the mucosa was disintegrated. Contrasting these results, the small bowel remained impermanent to HES over the duration of storage, residing within the lumen the entire time. Tissue morphology resembled that of near-normal bowel (with a minor degree of clefting) even after 12 h storage. Histologic Injury ( Table 1 and Fig. 4 ) At 4 and 12 h of cold storage, only AA-HES treatment exhibited significantly improved injury scores compared to both UW and AA-D70 groups ( P < 0.05); median grades in the AA-HES group were 0 and 2, respectively, indicating normal tissue and moderate clefting along the villus sides. At 8 h, differences between grades for all groups were not significant; median grades ranged from 2 to 3. After 12 h storage, median grades of injury in AA-D70 and UW groups were 4 and 6, respectively; these grades were indicative of completely denuded villi (grade 4) and injury to the Crypts of Lieberkühn (grade 6). Representative regions of injury depicting median grades are presented in Fig. 4 . Effect of D70 and HES on energy metabolism ( Fig. 5 ) Levels of ATP, AMP, and Energy Charge ratios exhibited a clear and distinct pattern throughout storage. The lowest levels for ATP and Energy Charge were in the UW group followed by preservation solution with D70 (AA-D70). The HES-containing solution (AA-HES) exhibited the highest levels of the three groups ( P < 0.05). Levels of the low energy adenylate, AMP, were elevated in the UW group at 4 h, but resumed initial t = 0 values by 8 h; values in AA-D70 and AA-HES were significantly less than UW group. At all time points, AA-HES values were maintained at the lowest level of the three experimental groups. Effect of D70 and HES on metabolic end-products ( Fig. 6 ) After 4 h, levels of anerobic end-product, lactate, rose by ∼50% above initial ( t = 0) P < 0.05; UW tissues exhibited no change. Values at 8 and 12 h were highest in the AA-HES group ( P < 0.05) followed by AA-D70 and UW groups. Products of amino acid metabolism, alanine and ammonium, exhibited varying degrees of accumulation with AA-HES maintaining the highest of the three groups by 8 h, P < 0.05. Effect of D70 and HES on oxidative stress ( Fig. 7 ) Levels of oxidative end-product, malondialdehyde (MDA), were highest in the UW treated group. Both AA-D70 and AA-HES groups showed excellent control of oxidative stress, as MDA levels remained low and constant throughout cold storage; P < 0.05. Discussion Dextrans are naturally occurring polymers of glucose produced by bacteria, the most well-known being of the Leuconostoc genus [31] ; hydroxyethyl starch (HES) is a semi-synthetic polymer of amylopectin [34] . Both molecules are colloids whose major clinical application has been for their volume expanding properties. In past years, the use of dextrans has been faced with considerable scrutiny due to the occurrence of anaphylactoid reactions. As a result, dextrans are now synthesised in such a way as to reduce the number of side chains, decreasing the incidence of severe anaphylactoid reactions [34] . Some reports even indicate that true anaphylactoid reactions resulting from dextrans occur with no significantly greater frequency than other colloids [24] . Others indicate that the rate of HES induced anaphylactoid reactions (0.006%) is remarkably lower than that of dextrans (0.273%) and other colloids [34] . Molecular weight (MW) affects the colloidal activity of dextrans and HES [30] . However, both of these colloids are polydisperse, meaning that a range of MW exists for any reported MW [34] . HES is particularly polydisperse, containing individual colloid molecules anywhere from a few thousand to over several million Daltons. MW can be characterized by a number of parameters including the weight average MW (MWw) or number average MW (MWn) [34] . The former directly depends on the average of all molecular weight fractions present (and not the number of molecules in each fraction) and is thus highly influenced by unrepresentative numbers of very small or large molecules [34] . On the other hand, the MWn represents a weighted average, reflecting the frequency of each individual colloid molecule [31] . By calculating the ratio of MWw-to-MWn, the polydispersity index (PDI) is given, which indicates either low or high MW variability (higher PDI values indicate wider variability in molecular weight; whereas a PDI = 1.0 indicates a pure molecular weight fraction). An additional property of HES that influences its collidal activity is the degree of substitution of the hydroxyethyl moiety [34] . Native starches are rapidly hydrolyzed in the mammalian body, but the higher the degree of hydroxyethylation of the glucosyl units at the C 2 position compared to C 6 , the slower the rate of starch degradation by endogenous enzymes [34] . When selecting a colloidal agent such as HES, these parameters describing the agent must be considered in order to avoid a significant component of low molecular weight fractions. Organ preservation solutions contain cell-impermeant molecules to counteract tissue edema during cold ischemic storage. A number of metabolic and physiologic changes accompany current storage strategies and contribute to the disruption of the mucosal barrier. Tight junctions, located near the luminal face of the epithelial cell layer, maintain an effective barrier; they consist of large dynamic proteins that are dependent on cellular energy levels [26] . Since energy-consuming processes still operate to some degree at low temperatures, energy levels rapidly decline, particularly in the face of restrictions on oxidative-phosphorylation during ischemia [26,10] . Consequently, tight junctions dilate and epithelial cells loose their ability to sustain a barrier, increasing the flux of intraluminal macromolecules across the mucosa [35] . Transepithelial flux of enteric bacteria is of particular concern due to direct implications for life-threatening sepsis. At a molecular level, the Na+/K+ pump maintains transmembrane ion gradients, such that extracellular Na+ levels are high and K+ levels are low (intracellular concentrations are reversed) [3] . ATP, produced primarily through oxidative phosphorylation, provides the energy required by the pump. Under normal conditions, energy levels are sustained, and Na+ is preferentially excluded from the intracellular space. The extracellular Na+ creates an osmotic pressure which counters the osmotic pressure exerted by impermeable anions and proteins. The force created by these intracellular sources has been calculated as 110–140 mOsm/kg. However, under conditions of cold ischemic storage, this delicate balance is disrupted. Collectively, hypothermia coupled with declining ATP levels suppress Na+/K+ pump function, thereby decreasing the cell membrane potential. In response to this charge disruption, Na+ and Cl − flow down their concentration gradients into the cell interior. As water follows the flux of ions, the cell begins to swell. If allowed to continue indefinitely, a loss of cell function and lysis of the cell membrane would occur. In order to offset the generated imbalance in osmotic forces, cell-impermeable agents can be utilized to maintain osmotic equilibrium. Clearly the maintenance of cell/tissue viability involves energetic and osmotic components, where either component influences the other. In the current communication, a metabolic attempt to generate ATP from both anerobic and aerobic processes was apparent. Within the first 4 h, all groups (including UW which contained no amino acid substrates) exhibited an accumulation of alanine and ammonium that would suggest catabolism of endogenous amino acids. However, at subsequent times (8 and 12 h) alanine was preferentially produced in tissues treated with the nutrient-rich solution (AA solution) supplemented with 5% hydroxyethyl starch, implicating the conversion of preservation solution amino acids. The relative contribution of anaerobic processes (i.e. glycolysis) was also greatest in the hydroxyethyl starch containing solution. These findings reflect the involvement of both aerobic and anaerobic metabolism in an attempt to replenish dwindling energy reserves. However, it is difficult to determine relative contributions of anaerobic versus aerobic metabolism to ATP synthesis since the rates of end-product transport out of the intestine during cold ischemia, either into the vasculature or into the lumen, are currently unknown. In order for a preservation solution to be effective, it must provide an external osmotic force sufficient to counteract intracellular forces; thus, preventing shifts in water leading to edema. Without an intraluminal flush and storage solution, it is difficult to provide adequate osmotic support to the mucosa, although there would be some contribution via the intravascular route. In the past, intravascular preservation solutions have used low molecular weight compounds such as mannitol, lactobionate, raffinose, and gluconate as effective osmotic impermeants. However, in the realm of an intraluminal preservation solution, the agents must remain impermeant to the mucosa. The exact molecular weight that defines a molecule as ‘impermeant’ has not been elucidated for an intraluminal preservation solution during cold storage. Over the last few years, we have included Dextran 70, lactobionate, and HES in our solution as arbitrary choices based on previous literature for primarily vascular impermeants [25,29,30] ; however, these choices are not supported by any direct evidence. Throughout the literature, the permeability of dextran (Mw = 4400 kDa) has been used as a reliable indicator of the effects of intestinal ischemia on mucosal permeability in in vivo models [12] . Ekstrom et al. demonstrated that FITC-labeled dextran (70 kDa) does not enter the systemic circulation or even enterocytes after orogastric administration albeit in healthy rats [7] . Furthermore, Nejdfors established an inverse relationship between mucosal permeability and molecular weight in multiple species, however larger molecules >70 kDa were not explored in the realm of intestinal ischemia [19] . Our current communication supports a negative correlation between molecular weight and permeability to the mucosal barrier during cold ischemia; and implicates the need for a high molecular weight molecule (>70 kDa) when employing an intraluminal preservation solution during organ storage. During the cold storage time-course, in which D70 and HES were incorporated into the intraluminal preservation solution, direct visualization with fluorescent micrographs revealed that D70 fully penetrated the intestinal barrier as early as 2 h. Moreover, these tissues had developed extensive damage, with epithelial clefts developing after 2 h, and complete denudation of the villi by 12 h. In contrast, grafts stored in the presence of HES exhibited an intact mucosal barrier and retained an intact morphology throughout all time-points. Our lab has utilized this high Mw (2200 kDa) starch (in AA-HES solution) while investigating intestinal ischemia–reperfusion and found positive effects on several metabolic aspects and a reduction in hemorrhagic events during reperfusion [27,28] . However, one of the concerns in the development of a novel preservation solution is its viscosity and whether elevated viscosity due to large amounts of osmotic and/or oncotic agents will influence perfusion pressures and flow rates to the detriment of the endothelium and parenchyma of the organ. The AA-HES solution has a measured viscosity of 3.93 ± 0.01 cP compared to 2.69 ± 0.01 cP for UW solution at 25 °C; viscosity at 4 °C is 9.24 ± 0.10 cP (AA-HES) versus 5.78 ± 0.08 cP (UW). Although solution viscosity is a significant concern for intravascular delivery, there is negligible risk of developing high pressures due to the increased viscosity alone during the administration of a preservation solution via the intraluminal route. In terms of practical application, the elevated viscosity does not preclude the effective intraluminal delivery of AA-HES solution even at reduced temperatures (4 °C). Although our study focuses on the importance of an intraluminal preservation solution tailored to intestinal metabolism, preservation of the vasculature remains an important aspect of organ viability. Damage to the endothelium can elicit inflammatory/immune responses leading to injury to the entire organ, so both vascular and intraluminal strategies are vital to organ viability. We have developed a strategy of intraluminal preservation solution administration based on the fact that the confines of clinical practice dictate that a common intra-aortic flush with a standard intravascular preservation solution is performed as part of a multi-organ procurement procedure. Once all relevant organs have been flushed intravascularly to clear the blood and initiate tissue cooling, the intestine is removed, thereby permitting further treatment of the intestine on the ‘back table’. In order for any change to current protocols to be instituted in the clinic, the proposed alteration must first, be safe and second, result in a substantial improvement in clinical outcomes; it is becoming apparent that our strategy of satisfies both criteria. Future studies will focus on translating the findings in this study to a small animal transplant model in order to demonstrate direct clinical applicability. To summarize, the permeability characteristics of the small intestine change markedly under ischemic conditions, permitting even relatively high molecular weight molecules such as D70 to cross the mucosal barrier during cold ischemia. In contrast, a high molecular weight HES remained impermeant throughout 12 h cold storage and contributed to the preferential maintenance of energetic parameters and mucosal morphology. The generation of ATP from both anaerobic and aerobic pathways was implicated. The results presented in this study support the use of HES (Mw = 2200 kDa) as an effective impermeant for an intraluminal preservation solution, however, the exact molecular weight limit remains to be elucidated. Acknowledgment Special thanks to Mr. Jacek Studzinski and Mrs. Geneva Hurd for excellent technical assistance. References [1] K. Abu-Elmagd J. Reyes G. Bond G. Mazariegos T. Wu N. Murase R. Sindhi D. Martin J. Colangelo M. Zak D. Janson M. Ezzelarab I. Dvorchik M. Parizhskaya M. Deutsch A. Demetris J. Fung T.E. Starzl Clinical intestinal transplantation: a decade of experience at a single center Ann. Surg. 234 2001 404 416 [2] S. Asfar R. Zhong D. Grant Small bowel transplantation Surg. Clin. North Am. 74 1994 1197 1210 [3] F.O. Belzer J.H. Southard Principles of solid-organ preservation by cold storage Transplantation 45 1988 673 676 [4] D.L. Bigam D.R. Grant Small bowel transplantation R.N. Ratnaike Small Bowel Disorders 2000 Oxford University Press Inc. New York 508 517 [5] I.W. Booth A.D. Lander Short bowel syndrome Baillieres Clin. Gastroenterol. 12 1998 739 773 [6] A.N. De Belder K. Granath Preparation and properties of fluorescein labeled dextrans Carbohyd. Res. 30 1973 375 378 [7] G.M. Ekstrom B.R. Westrom E. Telemo B.W. Karlsson The uptake of fluorescein-conjugated dextran 70, 000 by the small intestinal epithelium of the young rat and pig in relation to macromolecular transmission into the blood J. Developmental. Physiol. 10 1988 227 233 [8] A. Frenzer Short bowel syndrome R.N. Ratnaike Small Bowel Disorders 2000 Oxford University Press Inc. New York 492 507 [9] Y. Fujimoto D.W. Olson K.L. Madsen J. Zeng L.D. Jewell N.M. Kneteman D.L. Bigam T.A. Churchill Defining the role for a tailored luminal solution for small bowel preservation Am. J. Transplant. 2 2002 229 236 [10] B.J. Fuller The effects on cooling on mammalian cells B. Fuller B. Grout Clinical Applications of Cryobiology 1991 CRC London [11] D. Grant K. Abu-Elmagd J. Reyes A. Tzakis A. Langnas T. Fishbein O. Goulet D. Farmer 2003 report of the intestinal transplant registry: a new era has dawned Ann. Surg. 241 2005 607 613 [12] K. Inoue M. Oyamada S. Mitsufuji T. Okanoue T. Takamatsu Different changes in the expression of multiple kinds of tight-junction proteins during ischemia-reperfusion Injury of the rat ileum Acta Histochem. Cytochem. 39 2006 35 45 [13] N.V. Jamieson R. Sundberg S. Lindell K. Claesson J. Moen P.K. Vreugdenhil D.G. Wight J.H. Southard F.O. Belzer Preservation of the canine liver for 24–48 hours using simple cold storage with UW solution Transplantation 46 1988 517 [14] Y. Kokudo T. Furuya Comparison of University of Wisconsin, Euro-Collins, and lactated Ringer’s solutions in rat small bowel preservation for orthotopic small bowel transplantation Transplant. Proc. 26 1994 1492 1493 [15] A.N. Langnas Advances in small intestine transplantation Transplantation 77 2004 S75 S78 [16] O.H. Lowry N.J. Rosebrough A.L. Farr R.J. Randall Protein measurement with the Folin phenol reagent J. Biol. Chem. 193 1951 265 275 [17] A.D. Mayer Small bowel transplantation Baillieres Clin. Gastroenterol. 8 1994 561 580 [18] D.L. Morris Quantitative determination of carbohydrates with Dreywood’s anthrone reagent Science 107 1948 254 255 [19] P. Nejdfors M. Ekelund B. Jeppsson B.R. Westrom Mucosal in vitro permeability in the intestinal tract of the pig, the rat and man: species- and region-related differences Scand. J. Gastroenterol. 5 2000 501 507 [20] D.W. Olson H. Jijon K.L. Madsen M. Al-Saghier J. Zeng L.D. Jewell D.L. Bigam T.A. Churchill Human small bowel storage: the role for luminal preservation solutions Transplantation 76 2003 709 714 [21] H. Ohkawa N. Ohishi K. Yagi Assay for lipid peroxides in animal tissues by thiobarbituric acid reaction Anal. Biochem. 95 1979 351 358 [22] P.O. Park U. Haglund G.B. Bulkley K. Falt The sequence of development of intestinal tissue injury after strangulation ischemia and reperfusion Surgery 107 1990 574 580 [23] J.V. Passonneau O.H. Lowry Enzymatic Analysis: A Practical Guide 1993 Humana Press Totowa, NJ, USA [24] J. Paull A prospective study of dextran-induced anaphylactoid reactions in 5, 745 patients Anaesth. Intensive. Care 15 1987 163 167 [25] R.J. Ploeg D. Goosens P. Vreugdenhil Successful 72-hour cold storage kidney preservation with UW solution Trans. Proc. 20 1998 935 [26] P. Salehi J.Z. Zhu E.G. Castillo J. Avila J. Lakey T.A. Churchill Preserving the mucosal barrier during small bowel storage Transplantation 76 2003 911 917 [27] P. Salehi D. Bigam J.B. Ewaschuk K.L. Madsen G.T. Sigurdson L.D. Jewell T.A. Churchill Alleviating intestinal ischemia-reperfusion injury in an in vivo large animal model: developing an organ-specific preservation solution Transplantation 85 2008 878 884 [28] P. Salehi J. Walker K. Madsen G.T. Sigurdson B.L. Strand B.E. Christensen L.D. Jewell T.A. Churchill Relationship between energetic stress and pro-apoptotic/cytoprotective kinase mechanisms in intestinal preservation Surgery 141 2007 795 803 [29] P. Salehi K. Madsen J. Zhu E. Castillo J. Avila J.R. Lakey T.A. Churchill Alleviating ischemia-reperfusion injury in small bowel Am. J. Transplant. 4 2004 728 737 [30] P. Salehi L.F. Zhu G.T. Sigurdson L.D. Jewell T.A. Churchill Nutrient-related issues affecting successful experimental orthotopic small bowel transplantation Transplantation 80 2005 1261 1268 [31] J.B. Salmon M.G. Mythen Pharmacology and physiology of colloids Blood Rev. 7 1993 114 120 [32] E. Schweizer A. Gassel E. Deltz P. Schroeder Morphologic and histologic alterations after small bowel transplantation – a comparison of different solutions Transplant. Proc. 24 1992 1087 [33] L.K. Shanbhogue J.C. Molenaar Short bowel syndrome: metabolic and surgical management Br. J. Surg. 81 1994 486 499 [34] J. Treib J.F. Baron M.T. Grauer R.G. Strauss An international view of hydroxyethyl starches Intensive Care Med. 25 1999 258 268 [35] T. Tsukamoto S.K. Nigam Tight junction proteins form large complexes and associate with the cytoskeleton in an ATP depletion model for reversible junction assembly Biol. Chem. 272 1997 16133 16139 [36] J.A. Wahlberg R. Love L. Landegaard J.H. Southard F.O. Belzer 72-hour preservation of the canine pancreas Transpantation 43 1987 5 8 [37] J.M. Woodward D. Mayer The unique challenge of small intestinal transplantation Br. J. Hosp. Med. 56 1996 285 290
更多查看译文
关键词
Organ preservation solution,Intestinal-specific,Osmotic/oncotic agents,Mucosal permeability
AI 理解论文
溯源树
样例
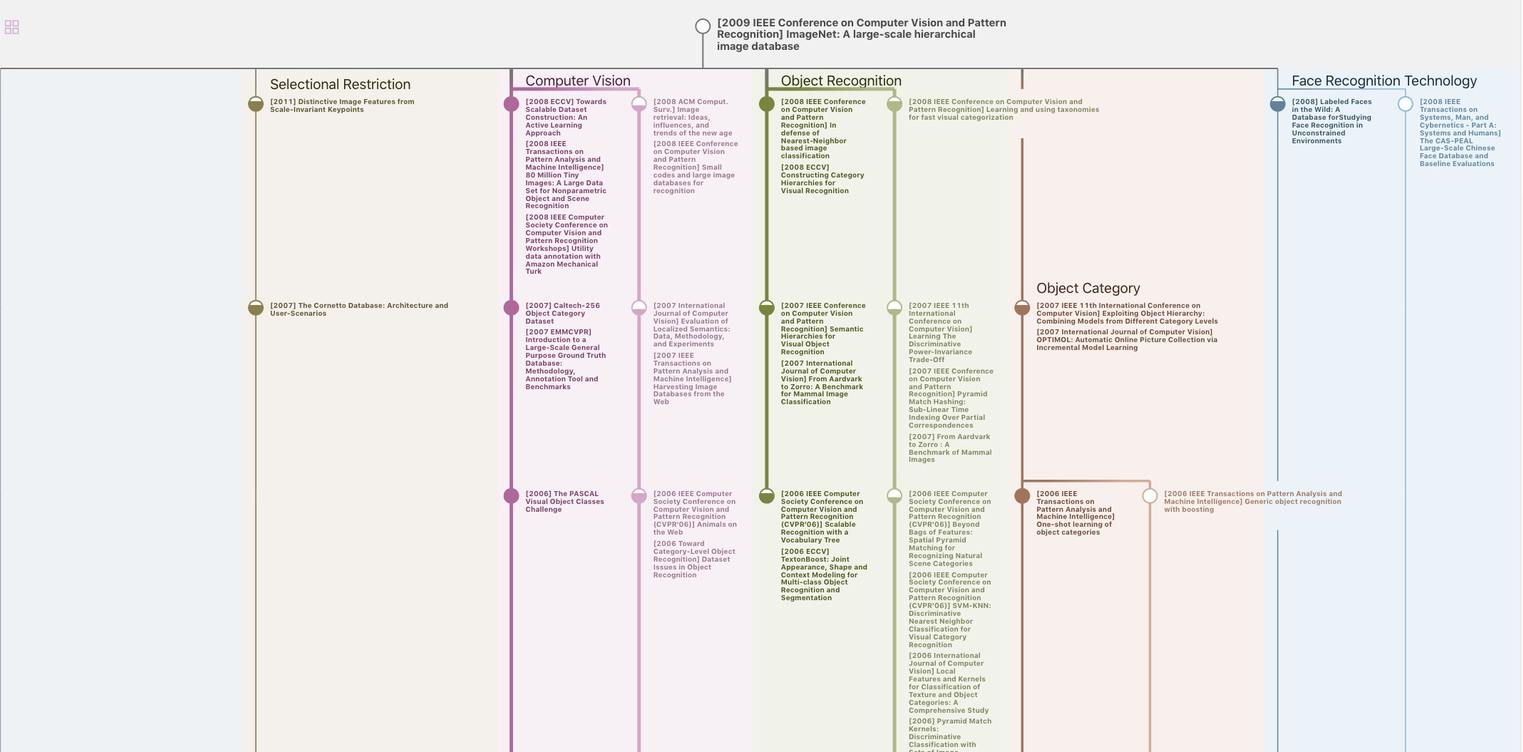
生成溯源树,研究论文发展脉络
Chat Paper
正在生成论文摘要