Feasibility and safety of G-CSF administration to induce bone marrow-derived cells mobilization in patients with end stage liver disease
Journal of Hepatology(2006)
摘要
Methods Eight patients with severe liver cirrhosis (Child–Pugh score B-C, spleen diameter less than 170 mm) were included. They were treated with G-CSF (5 μg/kg b.i.d for three consecutive days) to mobilize BMC, evaluated as circulating CD34+ve cells (flow cytometry) and myeloid CFU-GM progenitors (in vitro colony growth assay). Co-expression in CD34+ve cells markers of differentiation (Thy1, CD133, CXCR4, c1qRp) were investigated on CD34+ve cells by double direct immunofluorescence. Data from 40 healthy haematopoietic stem cell donors were used as controls. Results Mobilization of CD34+ve cells occurred in all patients. It was paralleled by expansion of circulating CFU-GM progenitors. Circulating CD34+ve cells co-expressed epithelial and stem cell markers in both cirrhotics and volunteer stem cell donors. G-CSF was well tolerated, no adverse event occurred, a significant reversible increase of splenic longitudinal diameter was observed. Conclusions (i) G-CSF mobilization of BMC co-expressing epithelial and stem markers occurred in all cirrhotic patients; (ii) splenomegaly up to 170 mm does not prevent safe BMC mobilization following G-CSF in patients with end stage liver disease; (iii) mobilized BMC may represent an easy immature cell source potentially useful for novel approaches for liver regeneration. Keywords Bone marrow-derived stem cell Liver disease G-CSF Abbreviations BMC bone marrow-derived cells G-CSF granulocyte-colony stimulating factor PB peripheral blood US ultrasound WBC white blood cell 1 Introduction Bone marrow-derived cells (BMC) can trans-differentiate into a variety of adult cell types including hepatocytes [1–5] . In response to acute or chronic liver damage, BMC can spontaneously populate liver and differentiate into hepatic cells [6,7] . Human BMC express multiple epithelial and liver markers when transplanted into rats with damaged liver [8] and, after peripheral blood stem cell transplantation, BMC repopulate liver and differentiate into epithelial and hepatic cells [7] . In particular, BMC can significantly contribute to hepatic regeneration after acute or subacute liver necrosis, when mature hepatocytes are inhibited in cellular duplication [9,10] . However some studies describe a fusion of BMC into liver cells as mechanism underlying liver tissue regeneration after injury, and the factual capacity of BMC to trans-differentiate or to fuse into liver cells is still a great controversial issue [5,11,12] . These observations have stimulated emerging interest in the potential use of BMC to repair severely damaged liver. Unfortunately, at basal conditions, a very low rate of BMC is involved into liver regeneration [13] while a massive BMC mobilization in peripheral blood (PB) circulation might lodge in the severely damaged liver. A non-invasive and potentially effective approach to obtain large quantities of BMC is their mobilization into PB by cytokines [14,15] . Granulocyte-colony stimulating factor (G-CSF), routinely used in haematological malignancies for BMC transplantation purposes, has potent mobilization capacity in donors and chronic haematological patients [16,17] . Moreover some studies suggest that cytokine-induced mobilization could also promote tissue repair in chronic or acute liver disease. G-CSF treatment significantly improved survival and liver histology in chemically injured mice, predominantly by promoting endogenous repair mechanisms [18] . Conversely splenomegaly, associated with liver cirrhosis and portal hypertension, might result in a potential restriction to BMC mobilization. Cell sequestration by the enlarged spleen can lessen or even abolish PB circulation of mobilized BMC [19] and liver failure might somehow affect the distinctive capacity of immature marrow cells to be released in PB in response to cytokine stimulation [20] . Moreover, splenic rupture is an uncommon though well-known complication, occasionally reported in a few patients and healthy donors undergoing BMC mobilization with G-CSF [21,22] . Thus, splenomegaly in patients with cirrhosis can considerably magnify the risk of splenic rupture following cytokine stimulation. To address these issues, a pilot study has been performed evaluating G-CSF-induced mobilization in selected patients with end stage liver cirrhosis. The aims of this study were to define the feasibility and safety of BMC mobilization and to characterize the phenotype of mobilized cells following G-CSF administration. 2 Patients and methods 2.1 Patients Between February 2004 and March 2005, eight patients with advanced liver cirrhosis entered this phase I/II study. Eligibility criteria included age between 18 and 80 years and advanced liver cirrhosis of any origin with a Child–Pugh score ⩾9 and a Meld score >10. Criteria of exclusion were the following: enlisted for liver transplantation, spleen diameter larger than 170 mm, diagnosis of hepatocellular carcinoma or other cancers, digestive haemorrhage due to portal hypertension in the last 7 days, recent diagnosis of portal vein thrombosis, severe renal or cardiac dysfunction, acute infection or disseminate intravascular coagulation. Demographic and clinical features are summarized in Table 1 . Five patients had alcoholic and three cryptogenic cirrhosis. Chronic alcohol assumption had been interrupted at least 12 months before enrollment. All patients had one or more episodes of liver decompensation in the previous 3 months (porto-systemic encephalopathy, abdominal ascites or variceal bleeding). Data from 40 adult healthy volunteers undergoing G-CSF mobilization at analogous dosage for allogenic haematopoietic stem cell transplantation were taken as controls. The protocol was approved by the Regional Ethical Committee. The study was carried out according to the Declaration of Helsinki. All patients gave formal written informed consent before entering the study protocol. 2.2 Mobilization protocol BMC mobilization was induced by G-CSF (Myelostim ® ), given subcutaneously at a dose of 5 μg/kg every 12 h for three consecutive days. All patients were hospitalised in our Liver Unit 1 day before starting on G-CSF and discharged 3 days after G-CSF discontinuation. Patients were monitored daily by physical examination and laboratory tests. In addition, ultrasound (US) scan and a US-Doppler were performed daily from day 0 to day 6, in order to evaluate changes of spleen diameter and of portal flow (vein thrombosis). Discharged patients were monitored monthly by clinical and laboratory investigations and US scan. 2.3 Assessment of BMC mobilization BMC mobilization was monitored by daily evaluation of circulating CD34+ve cells and in vitro clonogenic cells (CFU-GM assay) during cytokine administration and for 3 days thereafter. In addition, circulating CD34+ve cells were further characterized for the expression of various differentiation markers such as CD90, CD93, CD133, CD184 [23–32] , see below. 2.3.1 Evaluation of circulating CD34+ve cells Mobilized BMC were assessed as CD34+ve cells by flow cytometry. The assay was performed on freshly isolated cells. Briefly, 100 μL of whole blood was incubated with 20 μL of anti-CD34 PE monoclonal antibody (BD Biosciences, San Jose, CA) for 20 min at room temperature in the dark. Three microlitres of ammonium chloride lysing solution was added and incubated for 15 min for red blood cell lyses. Cells were then centrifuged at 1500 rpm for 5 min, washed in phosphate-buffered saline (PBS), and re-suspended in PBS for acquisition. The acquisition was performed by a FACSCalibur flow cytometer (BD Biosciences) and data analyzed using Cell Quest Software (BD Biosciences). A minimum of 60,000 events for each sample was acquired and analyzed. The number of CD34+ve cells per microliter was obtained by multiplying the percentage of CD34+ve cells by the number of white blood cells (WBC) in 1/μL of blood. 2.3.2 In vitro colony growth assay Circulating myeloid progenitors (CFU-GM) were evaluated daily, peripheral blood mononuclear low density (LD) cells were separated on a Ficoll–Hypaque gradient (density 1.077, Nygard, Oslo, Norway), then re-suspended in Iscove’s modified Dulbecco’s medium (IMDM). LD separated cells were cultured at different concentrations in triplicate dishes containing 1 mL of methylcellulose-based medium (HCC-4100; Stem Cell Technologies, Vancouver, Canada) and human recombinant cytokines (rhSCF, rhGM-CSF, rhIL3, rhG-CSF) +/− erythropoietin (Stem Cell, Vancouver, BC, cult™ GFH 4534). Cultures were incubated at 37 °C in a fully humidified 5% CO 2 atmosphere and evaluated after 14–18 days. The number of CFU-GM is expressed in milliliters of peripheral blood cells. 2.3.3 Immunophenotypic characterization of circulating CD34+ve cells Circulating CD34+ve were characterized by double immunofluorescence staining of cells using anti-CD34/PE or anti-CD34/FITC (BD Biosciences) and the appropriate amount of the following monoclonal antibodies: anti-CD133 (anti-AC133) [23,24] , PE (Miltenyi Biotec, GmbH), anti-CD90 PE (anti-Thy1) [25,26] and purified anti-CD93 (anti-C1qR) [27] (BD Biosciences Pharmingen, San Diego, CA), anti-CD184 FITC (anti-CXCR4) [28–32] (Medical and Biological Laboratories, Japan), goat anti-mouse Ig FITC (BD Biosciences). The cells were simultaneously stained with CD34 (FITC or PE) and one of the above-mentioned antibodies for 20 min at room temperature. The cells were then washed and re-suspended in PBS for acquisition and analysis. CD93 staining of CD34+ve cells was performed by a double indirect immunofluorescence: first, the cells were stained with purified anti-CD93 for 20 min, then washed and incubated with goat anti-mouse FITC for 10 min; after another wash with PBS, the cells were then incubated with anti-CD34 PE for 15 min. A minimum of 150,000 cells were acquired and analyzed in double staining technique, allowing the evaluation of specific antigen expression on the surface of the CD34+ve circulating cells. 2.4 Statistical analysis Categorical data are presented as absolute values and percentages, whereas continuous data are summarized as median and range or mean and SD. The Wilcoxon test was used for comparison of continuous variables. A value of p < 0.05 was regarded as significant. 3 Results 3.1 Procedure feasibility and early clinical outcome Out of 21 patients initially evaluated for protocol eligibility, eight patients were included in the study. Major causes of exclusion were: previous unknown hepatocellular carcinoma (five cases), spleen diameter superior to 170 mm (five cases), extra hepatic carcinoma (one case), severe cardiopulmonary disease (one case), refusal to sign informed consent (one case). Of the eight eligible patients, one underwent two mobilization procedures at 6 months interval. Overall, nine cycles of BMC mobilization were performed and evaluated. In all the mobilization procedures, G-CSF was well tolerated and no severe adverse events were observed. During cytokine stimulation, liver function enzymes remained stable and levels of α-fetoprotein did not significantly increase. A significant increase in longitudinal splenic major diameter was documented, median basal value was 150 mm (range 108–170 mm) and it increased to 180 mm at peak day (range: 128–194 mm) ( p < 0.05). Splenic diameter reverted to pre-treatment value within 3 days after G-CSF discontinuation ( Fig. 1 A). No thrombosis of portal system was observed during treatment and in the following follow-up. 3.2 BMC mobilization White blood cell (WBC) counts significantly increased, with peak values between day 3 and 4 ( p < 0.05). Counts then reverted to pre-treatment values within 3–5 days after G-CSF discontinuation as shown in Fig. 1 B. 3.2.1 CD34+ve cell assessment In all nine mobilization procedures, a significant increase of PB CD34+ve cells was observed following G-CSF administration. As shown in Fig. 2 A, the median pre-treatment value of PB CD34+ve cells was 1.43/μL (range 0.7–2.22/μL). Following G-CSF stimulation, a median peak value of 19.33/μL PB CD34+ve cells (range 6.7 and 29.14/μL) was recorded at day 3 ( p < 0.05). Peripheral CD34+ve cells reverted to the pre-treatment value within 3–4 days from G-CSF suspension. The patient receiving two cycles of G-CSF obtained a higher count of CD34+ve circulating cells during the second procedure (CD34+ve/μL peak: 7.88/μL vs 28.78/μL). Data from 40 healthy adult volunteers undergoing G-CSF mobilization were compared with those from cirrhotic patients. At day 3 of G-CSF stimulation there was a median peak value of 36.89/μL PB CD34+ve cells (range 2.6–130.06/μL), which means a significantly higher mobilization compared to cirrhotic patients ( p < 0.05). 3.2.2 CFU-GM assessment The CFU-GM colony assay proved the mobilization of BMC with in vitro clonogenic capacity. As shown in Fig. 2 B, the median peak value of circulating CFU-GM was 2843/mL (range 583–5415/mL) at day 4 since G-CSF starting, indicating a significant increase compared to pre-treatment values (median CFU-GM/mL: 116.5, range 37.9–212) ( p < 0.05). 3.2.3 Immunophenotypic characterization of circulating CD34+ve cells Immunophenotypic characterization for early stem and epithelial markers was performed on basal circulating CD34+ve cells and on day 3 of mobilization. All epithelial markers were expressed both in pre-treatment and mobilized CD34+ve cells at variable extents. Before and during G-CSF administration the percentage of cells co-expressing CD34 and epithelial markers such as CD90, CD93 was constant. Cells co-expressing CD34 and CD133 significantly increased after G-CSF stimulation ( p = 0.018) whereas cells co-expressing CD184 decreased ( p = 0.05) ( Table 2 ). No significant differences in the pattern of surface antigen co-expression by circulating CD34+ve cells were observed between cirrhotic patients and healthy donors (data not shown). 3.3 Clinical follow-up At a median follow-up of 8 months (range 5–17 months) all patients are alive. No severe adverse events have been reported at this time. In addition, no patient developed hepatocellular carcinoma nor any increase in α-fetoprotein levels. Liver function has been carefully monitored. Child–Pugh score improved by 2 or more points in four patients, worsened in one patient (from a Child–Pugh C10–C12), while it was unchanged (or improved by less than 2 points) in three patients. Overall, the MELD score improved from a median pre-treatment value of 17.5 (range 11–20) to 14.5 (range 9–20) at end of follow-up. Clinical amelioration was observed in six patients with reduction of encephalopathy episodes in two patients and ascites in four patients. One patient underwent a second mobilization course after 6 months. After the first mobilization treatment Child–Pugh score improved from C11 to B9 and following the second treatment the score further improved to a stable B8. Of note, patients with Child–Pugh and MELD score amelioration showed a higher mobilization rate of CD34+ve cells at peak day (median 26.5 CD34+ve cells/μL) compared to those with no change or worsening score (median 11.6 CD34+ve cells/μ) ( p < 0.01). 4 Discussion This pilot study was aimed to verify the feasibility and safety of BMC mobilization in patients with advanced liver cirrhosis and to characterize the phenotype of mobilized cells following G-CSF administration. Variable amounts of CD34+ve cells were mobilized in all patients in spite of their severe liver dysfunction. The procedure was feasible and safe suggesting that G-CSF mobilization can be considered as a novel approach for liver regeneration purposes. In haematological field, G-CSF is the most widely employed cytokine for BMC mobilization and collection [14,17] . Its potent mobilizing activity and high tolerability are well known [33–35] . G-CSF has been already safely used in patients receiving liver transplantation with the aim to reduce sepsis [36] or to improve neutrophil function in acute liver failure [37,38] . Considering the simplicity and the non-invasiveness of the mobilizing procedure, a pilot study has been performed in order to verify whether G-CSF administration could be safely used in cirrhotic patients with organ failure with the aim of inducing effective BMC mobilization. To support the use of G-CSF in patients with cirrhosis, a recent study reports that G-CSF accelerates recovery and improves survival in mice with acute or chronic liver injury [18] . Patients enrolled in this prospective trial had advanced liver disease and were not eligible for orthotopic liver transplant. Spleen enlargement was not an exclusion criterion if maximal spleen diameter was within 170 mm. Overall, the mobilization protocol applied to approximately 40% of evaluated patients. In our patients group the mobilization procedure showed to be safe and well tolerated. No severe adverse events occurred and in no case treatment had to be discontinued. During G-CSF stimulation serum liver enzymes and α-fetoprotein levels remained unchanged. Upper abdominal US scan measured a transient and reversible increase of spleen diameter in all patients. This was carefully monitored for the risk of splenic rupture [21,22] . All patients displayed a marked increase of circulating BMC, assessed as CD34+ve cells as well as in vitro clonogenic cells, in response to G-CSF administration. However the extent of BMC mobilization in our cirrhotic patients was significantly lower compared to that recorded in healthy donors. This lower rate of circulating CD34+ve cells could be ascribed to spleen enlargement which exerts an abnormal white cell sequestration [19] . In addition, liver failure may imply direct or indirect impairment of BM function, and this is particularly true in chronic post-alcoholic liver failure [39] . Finally, adult healthy donors are generally younger than our patients and receive an additional stimulus to BMC mobilization by the leucapheretic procedures. Taken together, all these aspects may explain the reduced BMC mobilization observed in our cirrhotic series. The feasibility and safety of the procedure allowed us to consider multiple cycles of G-CSF stimulation for boosting the BMC mobilization. Indeed, one patient who had a marked clinical improvement following the first G-CSF cycle underwent a second mobilization round after 6 months interval. The second G-CSF stimulation induced a significant increase of CD34+ve mobilization. This finding suggests that repeated courses of cytokine-induced BMC mobilization could be considered for future protocols. Signs of clinical improvement were noticed in about 50% of studied patients. No histological analysis could be performed due to the high risk of liver biopsy in cirrhotic patients. However, the patients with Child–Pugh and MELD score amelioration after G-CSF administration were those with a higher CD34+ve cells mobilization. Phenotype analysis showed that epithelial and early stem cell markers are expressed on the cell surface of mobilized CD34+ve cells in variable percentages. In detail, CD34+ve cells were assayed for the co-expression of the following surface antigens: (i) C1qR (CD93), known as complement receptor, and co-expressed by a rare stem cell population with both haematopoietic and hepatic differentiation properties [27] ; (ii) CXCR4 (CD184), receptor for SDF-1 (stromal cell-derived factor-1), which is considered an essential molecule in the mobilization and homing process of CD34+ve cells [28–32] ; (iii) AC 133 (CD133), which is expressed on both epithelial and haematopoietic stem cells, including liver progenitors detectable in PB after massive hepatic necrosis [23,24] ; (iv) Thy 1 (CD90), an early stem cell marker, co-expressed on human liver-residing stem cells [25,26] . Overall, the level of expression of epithelial markers on mobilized CD34+ve cells was found comparable in the study group and in healthy donor controls. Interestingly, in cirrhotic patients, expression of CD133 on CD34+ve cells significantly increased at peak day after G-CSF administration. CD133+ve cells could have a crucial role considering that their intraportal administration seemed to contribute to liver regeneration after partial hepatectomy in humans [40] and that they sensibly increase in the serum after partial hepatectomy in living liver donors [41] . In addition enriched CD133+ve cells generate an adherent cell population expressing CK8, α-fetoprotein and human albumin [41] . These findings suggest that CD133+ve/CD34+ve cells have potential hepatic differentiation capacity and our study showed that treatment with G-CSF can contribute to mobilize this particular cellular phenotype. In conclusion, our study shows the feasibility of BMC mobilization induced by G-CSF in end stage cirrhotic patients. The procedure is safe also in patients with advanced liver failure and splenomegaly. A significant increase in CD34+ve cells was observed in all patients, although the rate of BMC mobilization was lower compared to that recorded in healthy adult volunteers. No significant differences in terms of functional in vitro growth capacity and phenotypic characteristics of mobilized CD34+ve cells were observed between study patients and healthy controls. This procedure can be exploited in therapeutic strategies aimed at employing BMC for the regeneration of severely damaged liver. Mobilized BMC can be easily collected, immature cells can then be isolated, manipulated in vitro in order to initiate hepatic differentiation and then re-injected into the patient. Alternatively, we can consider that the large quantities of circulating BMC following G-CSF might favor the homing of immature cells in the damaged liver where they may possibly contribute to organ regeneration. Acknowledgements This study was supported by grants from “Ministero della Salute 2003, Progetto di ricerca finalizzata – Nuove modalità di valutazione del ricevente e del donatore per un miglioramento della qualità dei trapianti – No. 396, 17/12/2002” by “Regione Piemonte – Progetti di Ricerca Finalizzata”. G-CSF (Myelostim 34) was kindly provided by Italfarmaco. References [1] B.E. Petersen W.C. Bowen K.D. Patrene W.M. Mars A.K. Sullivan N. Murase Bone marrow as a potential source of hepatic oval cells Science 284 1999 1168 1170 [2] E. Lagasse H. Connors M. Al-Dhalimy M. Reitsma M. Dohse L. Osborne Purified hematopoietic stem cells can differentiate into hepatocytes in vivo Nat Med 6 2000 1229 1234 [3] N.D. Theise S. Badve R. Saxena O. Henegariu S. Sell J.M. Crawford Derivation of hepatocytes from bone marrow cells in mice after radiation-induced myeloablation Hepatology 31 2000 235 240 [4] N.D. Theise M. Nimmakayalu R. Gardner P.B. Illei G. Morgan L. Teperman Liver from bone marrow in humans Hepatology 32 2000 11 16 [5] Y. Jiang B.N. Jahagirdar R.L. Reinhardt R.E. Schwartz C.D. Keene X.R. Ortiz-Gonzalez Pluripotency of mesenchymal stem cells derived from adult marrow Nature 418 2002 41 49 [Epub 2002 Jun 20] [6] I. Ng K.L. Chan W.H. Shek J.M.F. Lee D.Y.T. Fong C.M. Lo High frequency of chimerism in transplanted livers Hepatology 38 2003 989 998 [7] M. Korbling R.L. Katz A. Khanna A.C. Ruifrok G. Rondon M. Albitar Hepatocytes and epithelial cells of donor origin in recipients of peripheral-blood stem cells N Engl J Med 346 2002 738 746 [8] S. Terai I. Sakaida N. Yamamoto K. Omori T. Watanabe S. Ohata An in vivo model for monitoring trans-differentiation of bone marrow cells into functional hepatocytes J Biochem (Tokyo) 134 2003 551 558 [9] S. Masson D.J. Harrison J.N. Plevris P.N. Newsome Potential of hematopoietic stem cell therapy in hepatology: a critical review Stem Cells 22 2004 897 907 [10] M.H. Dahlke F.C. Popp F.H. Bahlmann H. Aselmann M.D. Jager M. Neipp Liver regeneration in a retrorsine/CCl 4 -induced acute liver failure model: do bone marrow-derived cells contribute? J Hepatol 39 2003 365 373 [11] G. Vassilopoulos P.R. Wang D.W. Russell Transplanted bone marrow regenerates liver by cell fusion Nature 422 2003 901 904 [12] C.J. Vessey P.M. de la Hall Hepatic stem cells: a review Pathology 33 2001 130 141 [13] F. Fogt K. Beyser C. Poremba U. Khettry J. Ruschoff Recipient-derived hepatocytes in liver transplant: a rare event in sex-mismatched transplants Hepatology 36 2002 1 [14] A.M. Gianni S. Siena M. Bregni C. Tarella A.C. Stem A. Pileri Granulocyte–macrophage colony-stimulating factor to harvest circulating haematopoietic stem cells for autotransplantation Lancet 2 1989 580 585 [15] C. Tarella D. Ferrero M. Bregni S. Siena E. Gallo A. Pileri Peripheral blood expansion of early progenitor cells after high-dose cyclophosphamide and rhGM-CSF Eur J Cancer 27 1991 22 27 [16] W.P. Sheridan C.G. Begley C. Juttner Effect of peripheral blood progenitor cells mobilized by filgrastim (G-CSF) on platelet recovery after high-dose chemotherapy Lancet i 1992 640 644 [17] C. Tarella F. Zallio D. Caracciolo Hemopoietic progenitor cell mobilization and harvest following an intensive chemotherapy debulkying in indolent lymphoma patients Stem Cells 17 1999 55 61 [18] E. Yannaki E. Athanasiou A. Xagorari V. Constantinou I. Bastis P. Kaloyannidis G-CSF-primed hematopoietic stem cells or G-CSF per se accelerate recovery and improve survival after liver injury, predominantly by promoting endogenous repair programs Exp Hematol 33 2005 108 119 [19] P.J. Toghill S. Green Splenic influences on the blood in chronic liver disease Q J Med 48 1979 613 625 [20] C. Di Capli A.C. Piscaglia S. Giuliante F. Rutella G. Bonanno M.A. Zocco No evidence of hematopoietic stem cell mobilization in patients submitted to hepatectomy or in patients with acute on chronic liver failure Transplant Proc 37 2005 2563 2566 [21] H. Balaguer A. Galmes G. Ventayol J. Bargay J. Besalduch Splenic rupture after granulocyte-colony-stimulating factor mobilization in a peripheral blood progenitor cell donor Transfusion 44 2004 1260 1261 [22] D.P. O’Malley M. Whalen P.M. Banks Spontaneous splenic rupture with fatal outcome following G-CSF administration for myelodysplastic syndrome Am J Hematol 73 2003 294 295 [23] R. Handgretinger P.R. Gordon T. Leimig X. Chen H.J. Buhring D. Niethammer Biology and plasticity of CD133 + hematopoietic stem cells Ann N Y Acad Sci 996 2003 141 151 [24] A.J. Potgens U. Schmitz P. Kaufmann H.G. Frank Monoclonal antibody CD133-2 (AC141) against hematopoietic stem cell antigen CD133 shows crossreactivity with cytokeratin 18 J Histochem Cytochem 50 2002 1131 1134 [25] H.C. Fiegel J.J. Park M.V. Lioznov A. Martin S. Jaeschke-Melli P.M. Kaufmann Characterization of cell types during rat liver development Hepatology 37 2003 148 154 [26] T. Ishii K. Yasuchika H. Fujii T. Hoppo S. Baba M. Naito In vitro differentiation and maturation of mouse embryonic stem cells into hepatocytes Exp Cell Res 309 2005 68 77 [27] G.H. Danet J.L. Luongo G. Butler M.M. Lu A.J. Tenner M.C. Simon C1qRp defines a new human stem cell population with hematopoietic and hepatic potential Proc Natl Acad Sci U S A 99 2002 10441 10445 [28] O. Kollet S. Shivtiel Y.Q. Chen J. Suriawinata S.N. Thung M.D. Dabeva HGF, SDF-1, and MMP-9 are involved in stress-induced human CD34+ve stem cell recruitment to the liver J Clin Invest 112 2003 160 169 [29] M. Kucia J. Ratajczak R. Reca A. Janowska-Wieczorek M.Z. Ratajczak Tissue-specific muscle, neural and liver stem/progenitor cells reside in the bone marrow, respond to an SDF-1 gradient and are mobilized into peripheral blood during stress and tissue injury Blood Cells Mol Dis 32 2004 52 57 [30] M.Z. Ratajczak M. Kucia R. Reca M. Majka A. Janowska-Wieczorek J. Ratajczak Stem cell plasticity revisited: CXCR4-positive cells expressing mRNA for early muscle, liver and neural cells ‘hide out’ in the bone marrow Leukemia 18 2004 29 40 [31] M. Kucia K. Jankowski R. Reca M. Wysoczynski L. Bandura D.J. Allendorf CXCR4-SDF-1 signalling, locomotion, chemotaxis and adhesion J Mol Histol 35 2004 233 245 [32] H.M. Hatch D. Zheng M.L. Jorgensen B.E. Petersen SDF-1alpha/CXCR4: a mechanism for hepatic oval cell activation and bone marrow stem cell recruitment to the injured liver of rats Cloning Stem Cells 4 2002 339 351 [33] R.E. Champlin N. Schmitz M.M. Horowitz Blood stem cells compared with bone marrow as a source of hematopoietic cells for allogeneic transplantation Blood 95 2000 3702 3709 [34] C. Tarella P. Gavarotti D. Caracciolo P. Corradino C. Cherasco C. Castellino Haematological support of high-dose sequential chemotherapy: clinical evidence for reduction of toxicity and high response rate in poor risk lymphomas Ann Oncol 6 1995 3 8 [35] N. Schmitz D.C. Linch P. Dreger A.H. Goldstone M.A. Boogaerts A. Ferrant Randomised trial of filgrastim-mobilised peripheral blood progenitor cell transplantation versus autologous bone-marrow transplantation in lymphoma patients Lancet 347 1996 353 357 [36] D.J. Winston P.F. Foster K.A. Somberg R.W. Busuttil M.F. Levy P.A. Sheiner Randomized placebo-controlled, double blind, multicenter trial of efficacy and safety of granulocyte colony stimulating factor in liver transplant recipients Transplantation 68 1999 1298 1304 [37] M. Rolando N. Clapperton J. Wade G. Penetsos G. Mufti R. Williams Granulocyte colony-stimulating factor improves function of neutrophils from patients with acute liver failure Eur J Gastroenterol Hepatol 12 2000 1135 1140 [38] M. Rolando N. Clapperton J. Wade J. Wndom Administering granulocyte colony-stimulating factor to acute liver failure patients corrects neutrophil defects Eur J Gastroenterol Hepatol 12 2000 1323 1328 [39] A. Panasiuk A. Kemona Bone marrow failure and hematological abnormalities in alcoholic liver cirrhosis Rocz Akad Med Bialymst 46 2001 100 105 [40] J.S. am Esch W.T. Knoefel M. Klein A. Ghodsizad G. Fuerest L.W. Poll Portal application of autologous CD133 + bone marrow cells to the liver: a novel concept to support hepatic regeneration Stem Cells 23 2005 463 470 [41] U.M. Gheling M. Willems M. Dandri J. Petersen M. Berna M. Thill Partial hepatectomy induces mobilization of a unique population of haematopoietic progenitor cells in human healthy liver donors J Hepatol 43 2005 845 853
更多查看译文
关键词
Bone marrow-derived stem cell,Liver disease,G-CSF
AI 理解论文
溯源树
样例
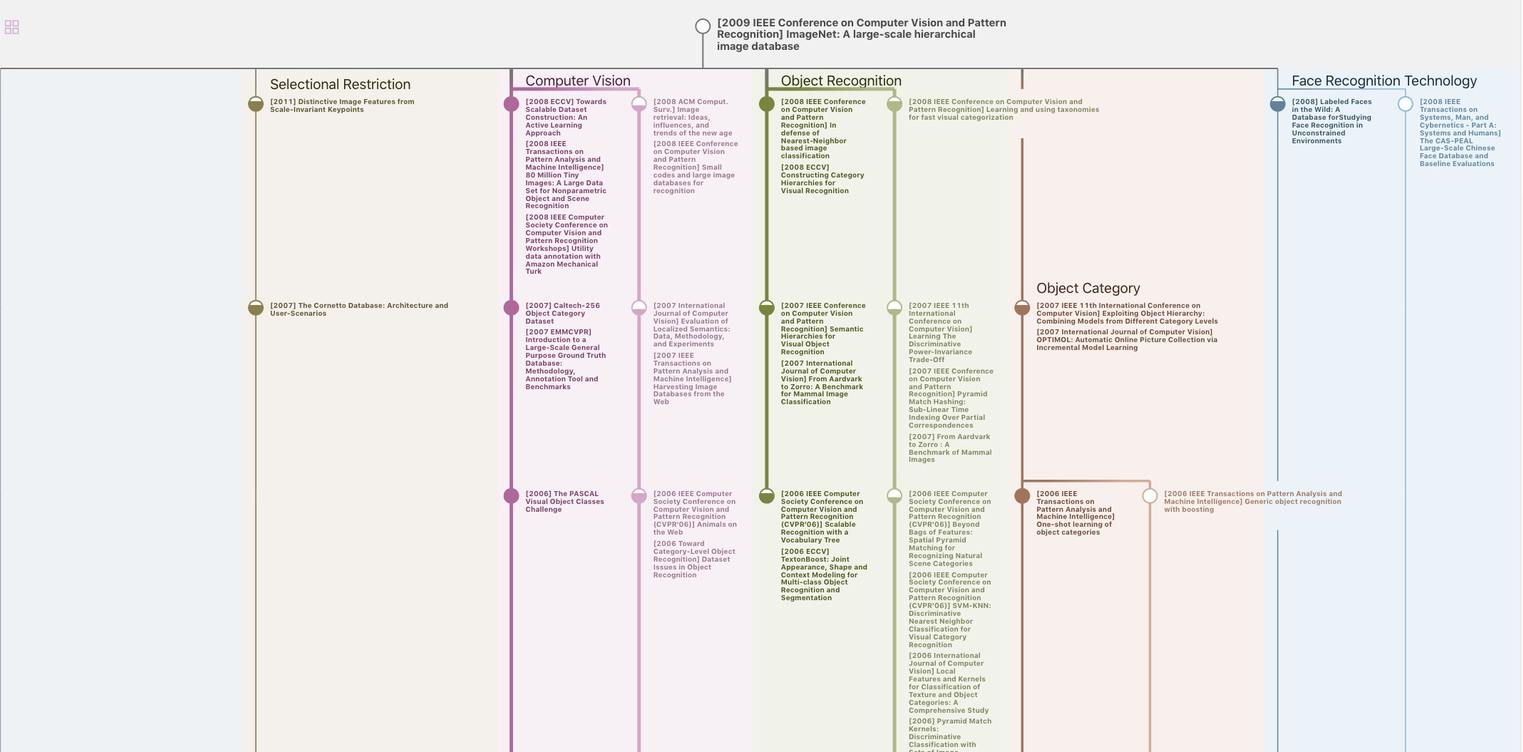
生成溯源树,研究论文发展脉络
Chat Paper
正在生成论文摘要