Determining Kidney Obstructions by Renogram Data Modeling
Elsevier eBooks(2023)
摘要
In this chapter, we are presenting our work on Renography Modeling, to (i) develop quantifiable means for differentiating between normal kidney, mildly, and severely obstructed kidney, and (ii) obtain a measure of urine flow rate index for this purpose. In renography, a tracer is introduced into the blood circulation. The kidney extracts this tracer from the blood circulation in the glomeruli, and for a transient period of time in a normal person, the tracer is found within the renal parenchyma. From the renal parenchyma, it is excreted into the collecting system of the kidney (mainly the renal pelvis), from where it travels out of the kidney into the urinary bladder. Our aim is to model the behavior of the tracer from input into renal parenchyma to output from renal pelvis, by using mathematical modeling in the form of a differential equation and develop (i) quantifiable measures as well as (ii) urine flow rate, to differentiate between normal kidney and slightly obstructed or heavily obstructed kidney. If a tracer is injected into the blood circulation and the amount of tracer is measured by radioactive means in the renal outflow, then Figure 10.11 illustrates the curves in a normal kidney and in an obstructed kidney. Now the tracer flow and the kidney structure can be divided into two control volumes, (1) chamber 1 of renal parenchyma, and (2) chamber 2 of renal pelvis, as shown in Figure 10.13. In this figure, we are displaying how the tracer I(t) is input into the renal parenchyma, where it has concentration C1. Then the tracer along with the blood, as FC1, travels into the renal pelvis compartment, where it gets concentration C2. From there, it exits into urine as U(t)C2. Then we put down the differential equations for flow rate of tracer amounts in chambers 1 and 2. Finally, we get the governing differential Equation (10.22, in the chapter), as follows:(10.22)V2C..+βC˙+γC=(FV1)Iwhere(10.23)(FV2V1+U)=β(FUV1)=γ In this DEq, V1: volume of chamber 1, renal parenchyma in dL; V2: volume of chamber 2, renal pelvis in dL; I(t): tracer input function in Counts/sec; F(t): blood flow from chamber 1 to chamber 2 in dL/sec; U(t): urine outflow in dL/sec. This DEq has three types of solutions (1) underdamped, associated with normal kidney (2) overdamped, associated with obstructed kidney having a prolonged renal transit time of tracer, and (3) critically damped, associated with partially obstructed kidney. For the underdamped case, by using G2(t) = C(t)V2, the solution of the governing DEq for the amount of tracer is represented as:(10.28)G2(t)=kV2A1e−DtsinA1twhere k=FV1V2; A1=γV2−β24V22 ; D=β2V2 The parameters A1, D and kV2 can be determined by matching the Equation (10.28) (solution of Equation 10.22) with the renogram data. The value of the normalized urine flow rate U/V2 index can then be found from the following algebraic equations:(10.29)A12=γV2−D2(10.30)γV2=FV1UV2V2V2=(kV2)UV2 For the overdamped case, the solution of the governing DEq is given by:(10.32)G2(t)=kV2A2e−DtsinhA2twhere k=FV1V2, A22=β24V22−γV2, D=β2V2, and the parameters of A2, D and kV2 can be determined by matching Equation (10.32) with the renogram data. Then, the value of the normalized urine flow rate U/V2 index can be evaluated by using Equations (10.29) and (10.30). For the critically damped case, the solution of the governing DEq is given by:(10.34)G2(t)=kV2te−Dtwhere k=FV1V2 ; D=β2V2; and the parameters A1, D and kV2 can be determined by matching the solution Equation (10.34) with the renogram data. The value of U/V2 can then be calculated by using:(10.35)D2=UV2kV2 In order to match the three cases of model solutions to the tracer data, it is better for us to match the solution in terms of G2(t)/G(max), so that we would not need to be concerned with units for the tracer. Now for matching this normalized renogram date, the corresponding model solution equations are (1) Equation (10.28)/G(max) for the underdamped case, (2) Equation (10.32)/G(max) for the overdamped case, and (3) Equation (10.34)/G(max) for the critically damped case. These model solution equations are then compared to and matched with the normalized renogram by using MATLAB. The function of curve fitting in MATLAB is applied to fit the clinical data with the derived model solutions by using compartmental analysis. Each sample renogram data is fitted by all the 3 system solutions, that is, underdamped, overdamped and critically damped. The curve fittings of Patient 1’s right kidney are displayed in Figure 10.15. As shown in the Table 10.2, the best curves used to represent both the kidneys of Patient 1 are for the overdamped system. The parametric values of the model solution that best fits the renogram data are determined by MATLAB. Then the magnitudes of the normalized urine flow rate index U/V2 are calculated from the algebraic Equations (10.29) and (10.30). In this way, we can assess all the patients, determine which of the three types of solutions best fit the normalized renogram data, and use the appropriate equations to evaluate the model parameters and calculate their urine flow rate index U/V2. For the novel index U/V2 representing normalized urine flow rate, the higher values of U/V2 indicate faster flow and hence no obstruction or normal kidney. The lower values of U/V2 indicate slower flow implying obstructions in the flow. Table 10.5 shows higher values of U/V2 corresponding to the normal kidneys, medium values of U/V2 corresponding to slightly obstructed kidneys and the lower values associated with heavily obstructed kidneys. Hence the developed mathematical model representing the transport of the tracer through the kidney is valid, by which this urine flow rate U/V2 index can be used as a clinical index in differentiating kidney obstruction.
更多查看译文
关键词
kidney obstructions,renogram data modeling
AI 理解论文
溯源树
样例
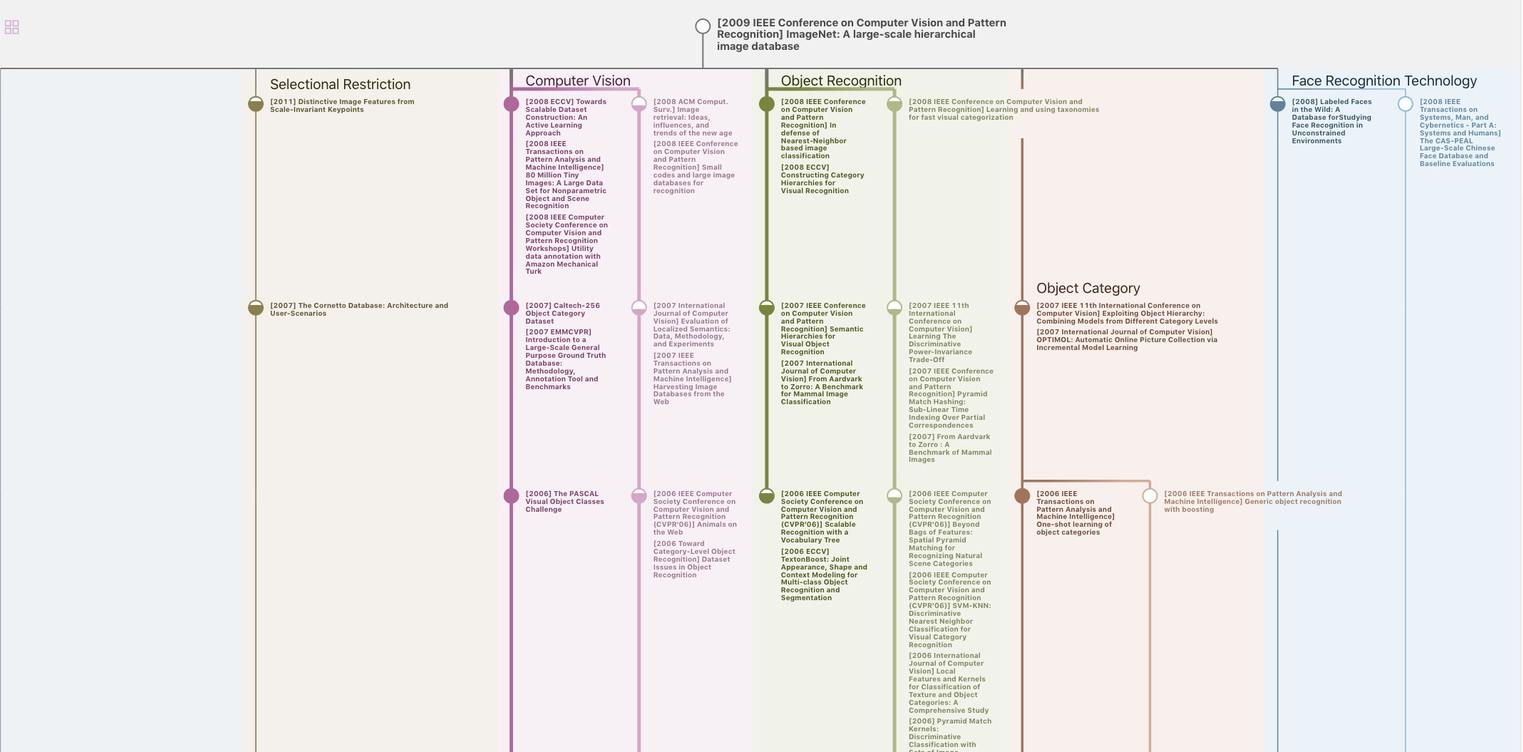
生成溯源树,研究论文发展脉络
Chat Paper
正在生成论文摘要