Complexities, similarities, and differences in circadian regulation in the green lineage
NEW PHYTOLOGIST(2024)
摘要
The circadian clock is an internal time-keeping system that generates c. 24 h circadian rhythms. These rhythms are thought to align the phase of biological processes with the time of day in the environment. Three key properties of circadian rhythms are: free running of the rhythm without external cues, entrainment to external cycles, and temperature compensation of the period. These properties are widely conserved across organisms. Circadian rhythms in plants are thought to be generated by a transcriptional/translational feedback loop (TTFL) formed from core clock genes and proteins (Nohales & Kay, 2016). These circadian oscillations, and the circadian clock, can function even in single plant cells. Temporal information derived at the single-cell level is thought to be shared among cells, tissues, and organs, resulting in coordinated rhythms at the organismal level. In other words, circadian oscillators can produce physiological rhythms that have hierarchical organizational architectures. The mechanisms of the circadian clock have been studied extensively using the model plant Arabidopsis thaliana (Arabidopsis). However, there have been exciting developments in the investigation of circadian regulation in divergent plants ranging from green algae to crops. The knowledge obtained from various plant species is advancing understanding of the similarities and diversities of circadian clock mechanisms in the green lineage (Fig. 1). Addressing some of these questions formed part of the 33rd International Conference on Arabidopsis Research (ICAR2023), held in Japan. The conference included a session entitled, ‘Understanding circadian regulation in unpredictable environments’, organized by Antony Dodd (John Innes Centre, UK) and Tokitaka Oyama (Kyoto University, Japan). Several speakers studying various plants shared their findings to provide new insights into the questions above. The three properties of circadian rhythms described above, free running, entrainment, and temperature compensation, are crucial for understanding circadian clock systems. Free running is the property of maintaining a circadian rhythm, with a period of c. 24 h, without rhythmic environmental cues (for example, under constant light and constant temperature conditions) (Fig. 2a). Entrainment allows organisms to align their intrinsic rhythm with day–night cycles. Entrainment causes a phase shift (i.e. phase advance or phase delay), depending on the circadian phase, in response to an environmental cue (Fig. 2b). The circadian oscillator also regulates the magnitude of responses to stimuli according to the phase of the circadian clock (circadian gating). Such responses include gene expression, metabolism, and physiological outputs. Temperature compensation is the ability of the circadian clock to maintain a period of c. 24 h over a range of physiological temperatures (Fig. 2c). These three properties have been studied extensively, across life, over the past 70–80 yr (McClung, 2006). Although many clock-related genes have been identified in Arabidopsis, the molecular mechanisms underlying these three properties remain unclear. In the session at ICAR2023, Shu-Hsing Wu (Academia Sinica, Taiwan) provided a new molecular mechanism underlying the oscillation of the circadian clock. With colleagues, she investigated upstream open reading frame (uORF)-mediated translational regulation to understand why a stable circadian rhythm can be maintained within an essentially noisy process (Wu et al., 2022). The autonomous oscillation of gene expression is driven by the TTFL of circadian clock genes, so precise regulation of transcription and translation is required. However, bursts of de novo transcription and translation can produce fluctuations in mRNA and protein levels (Eldar & Elowitz, 2010). The Wu group has demonstrated that the uORFs upstream of the core clock gene TIMING OF CAB EXPRESSION 1 (TOC1) are involved in reducing such intrinsic noise. In addition, they suggested that these uORFs may effectively buffer TOC1 protein production, and discussed the possibility that this uORF-mediated regulation is a shared mechanism for maintaining the period length in other organisms. Antony Dodd presented the genome-wide circadian gating of cold responses in bread wheat (Graham et al., 2023). Low temperature is known to reduce crop yield by reducing photosynthetic capacity and causing photoinhibition (Hurry & Huner, 1992; Li et al., 2015). Therefore, with colleagues, he considered the possibility that the circadian gating of low temperature responses might affect crop performance. They found genome-wide circadian gating of low temperature responses in hexaploid bread wheat, as previously demonstrated in Arabidopsis, suggesting conserved regulatory mechanisms for temporal environmental response across angiosperms. These findings aid our understanding of circadian clock systems in crops and also provide helpful information for producing more climate change resilient crops in the future. Akari Maeda (Nagoya University, Japan) focused on temperature compensation in Arabidopsis. Previous studies reported that some mutants impair temperature compensation (Salome et al., 2010). However, the molecular mechanisms of temperature compensation are still unclear. She and her colleagues found that the quantitative control of clock protein abundance is essential for temperature compensation in Arabidopsis. They also reported that ubiquitin-dependent degradation is associated with temperature-dependent quantitative regulation of the clock protein abundance, revealing a novel molecular mechanism underlying temperature compensation. Takuya Matsuo (Kitasato University, Japan) presented the similarities and differences between the clock genes in Arabidopsis and Chlamydomonas reinhardtii (Chlamydomonas), a eukaryotic unicellular alga. He and his colleagues have identified clock genes in Chlamydomonas (Matsuo et al., 2008). They found that one of the clock proteins, RHYTHM OF CHLOROPLAST 15 (ROC15), is degraded by light stimuli, and this light-inducible degradation of ROC15 is involved in resetting the circadian clock (Niwa et al., 2013). In this session, he explained how Chlamydomonas-ELF3-like (CETL) is involved in the degradation pathway of ROC15. Interestingly, CETL has a very weak similarity to EARLY FLOWERING 3 (ELF3) in Arabidopsis (Gururaj et al., 2022). These findings are very helpful for understanding the evolution of the circadian clock genes in the green lineage. Individual plant cells possess a functional circadian clock (Davis et al., 2022). At the same time, there are differences in the period and phase among different parts of a plant. There is accumulating evidence for the coupling of circadian rhythms between cells and tissues in plants (Davis et al., 2022). However, how these circadian oscillators interact with each other remains poorly understood. Tokitaka Oyama presented an investigation of a noncell-autonomous circadian rhythm and its characteristics. Using single-cell level imaging with a duckweed species (Lemna minor), his group succeeded in monitoring dual-color bioluminescence reporters (Arabidopsis CIRCADIAN CLOCK-ASSOCIATED1::luciferase + (AtCCA1::LUC+) under the circadian clock and 35S::modified click-beetle red-color luciferase (CaMV35S::PtRLUC)) (Muranaka & Oyama, 2016; Watanabe et al., 2023). Both AtCCA1::LUC+ and CaMV35S::PtRLUC had bioluminescence rhythms with periods of c. 24 h. The amplitude of the rhythm of AtCCA1::LUC+ was reduced by overexpressing an effector of the clock (e.g. LmZTL), whereas the rhythm of CaMV35S::PtRLUC was not altered. In comparison, plasmolysis disrupted the CaMV35S::PtRLUC rhythm but did not affect AtCCA1::LUC+ rhythm. Thus, the CaMV35S::PtRLUC rhythm is noncell-autonomous and likely to be driven by symplast/apoplast mediated cellular interactions. This process could be involved in the coupling between cellular oscillators. James Locke (University of Cambridge, UK) used a mathematical approach to explain complex spatial waves of clock gene expression observed by fluorescence imaging (Greenwood et al., 2022). It was found that the interaction among oscillators possessing different periods possibly generates spatial waves. The different sensitivity to environmental stimuli between different types of tissues could be a factor for the period variations within a plant (Greenwood et al., 2022). With colleagues, he demonstrated that different sensitivity to stimuli can explain the period differences observed within plants. In addition, they demonstrated that the local coupling of clock components is necessary for forming spatial waves through a clock network model with spatial information. Finally, they analyzed the model behavior under a noisy light–dark cycle and showed that the local coupling minimized the timing errors, while the clocks in each tissue maintained phase differences (Greenwood et al., 2022). This session provided a valuable opportunity to discuss the circadian clock system with researchers studying not only Arabidopsis but also other plant species. It is possible that most of the core clock components in Arabidopsis have been identified. This provides an excellent opportunity to move on to the next mysteries of the circadian clock, which were discussed deeply during this session. Furthermore, this session taught us how advanced molecular techniques and mathematical approaches help our understanding of cell-, tissue-, and organ-specific clock properties, and the integration of temporal information from cell levels to organ levels. The clock properties discussed in this session, such as translational regulation, posttranslational regulation, gating, resetting, and coupling are all important for keeping time and responding accurately to fluctuating and unpredictable environments. In addition to the speakers, many well-established participants made this session very active and fruitful. The insights provided by this session will advance our understanding of not only the circadian clock of Arabidopsis, but also the mysteries of circadian clocks across the green lineages. The authors thank all session presenters and participants, ICAR for hosting several circadian clock sessions, and sponsors including the New Phytologist Foundation that made it possible to organize this session.
更多查看译文
关键词
Arabidopsis,Chlamydomonas,chronobiology,circadian regulation,Lemna,transcription-translation feedback loops,unpredictable environments
AI 理解论文
溯源树
样例
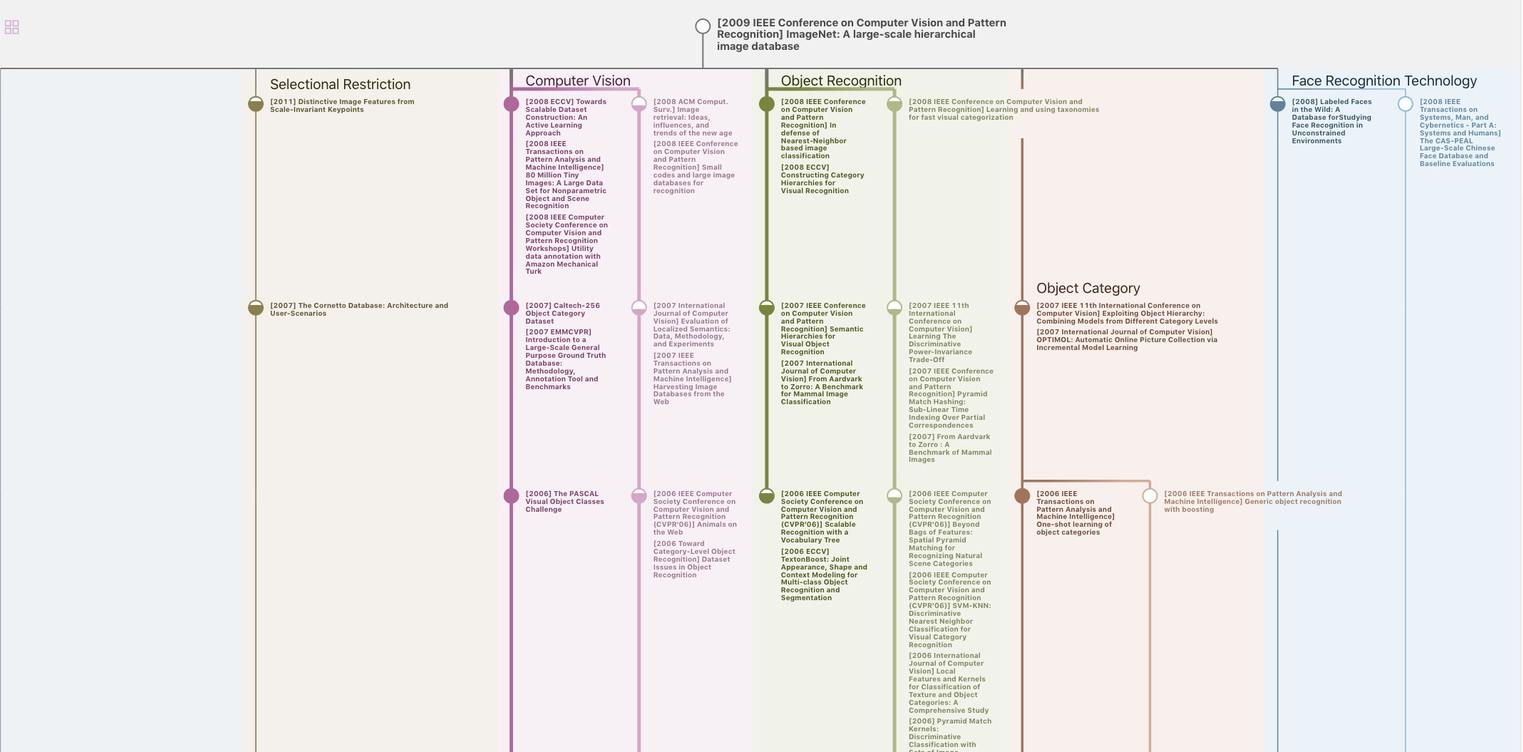
生成溯源树,研究论文发展脉络
Chat Paper
正在生成论文摘要