The quality of red cells stored under blood bank conditions: Is donor age just a number?
Transfusion(2023)
摘要
Red cell concentrates are the single most transfused cellular blood product, and millions of units are collected annually to help those in need. Yet, shortages remain, and one of the ways to enlarge the pool of eligible donors is by allowing older and younger donors to donate. In many jurisdictions, traditionally, the age range to donate blood was between 18 and 65. Nowadays, people as young as 16 can donate, as well as those in their 70s and 80s.1 And with the general increasing wellbeing of older people, this upper limit could altogether disappear, just as long as there is no harm to the donor, and the blood products remain of adequate clinical efficacy. Notably, there is no upper age limit for blood donation in the United States. However, there is not much information on the relationship between the quality of blood products and the age of the donors. After collection, a unit of whole blood is processed into a red cell concentrate, and this is stored until the unit is needed for a patient, with a maximum storage time of five to six weeks at refrigerated temperatures. The storage conditions in the blood bank are entirely different as compared to the environment of the human body: red cells are suspended in an additive solution rather than in plasma, they remain stationary in a plastic container rather than circulate in the human body, in the plastic container there is no interaction with other (blood) cells or organs, and the red cells are stored at 1–6°C rather than at body temperature. Although specialized storage media and conditions are developed to optimize red cell quality during storage, the gradual process of red cell aging cannot be completely halted. The collective changes that are associated with gradual deterioration of red cell quality is what is known as the storage lesion, which is a process that can be strikingly different between donors.2, 3 Various red cell quality parameters are known to be subject to large inter-donor variations, such as in ATP content, hemolysis, and percent recovery after transfusion.4 For example, red cells from certain donors are repeatedly associated with recoveries around 50%, while others are consistently upwards of 90%. If donors with an inherent low red cell recovery are included in a study to register a new blood product, then the new product may fail to meet predefined acceptance criteria. However, once approved, red cell recoveries are not determined on an individual basis, and a failing unit will have no consequences at best, or require an additional transfusion at worst. There are some general explanations as to why this is the case. Hemoglobin concentration, for example, is sex-related,5 and therefore explains why red cell concentrates from female donors have on average a lower hemoglobin content than those obtained from men. Sex hormones influence mechanical red cell fragility.6 Red cell fragility can be measured with a laboratory test to simulate shear stress, where a red cell suspension is incubated on a rocker with a stainless-steel ball bearing for 1 h, and the resulting hemolysis is the read-out. Red cells from premenopausal women tend to show lower levels of shear stress-induced hemolysis than age-matched men7 or postmenopausal women.6 Other factors that induce inter-donor differences include smoking, sickle cell trait, glucose-6-phosphate dehydrogenase deficiency, to name a few, as reviewed elsewhere.3 The age of the donors is also a factor of note, and this was described in the literature only recently.8 In the REDS-III RBC-Omics study cohort, which contains donors from 18 to about 90 years old, an association was found between oxidative hemolysis and donor age (and sex).8 The oxidative hemolysis in vitro test measures the percentage of hemolysis following the addition of an oxidant that produces free radicals. The investigators found a higher oxidative hemolysis in red cells from male as compared with female donors, decreasing for both sexes with increasing age. They also found similar sex and age associations for hemolysis induced by storage (storage-induced hemolysis) and hemolysis induced after incubation with a hypotonic solution (osmotic-stress hemolysis). D'Alessandro et al.9 found, in the same REDS-III RBC-omics cohort, comparable differences in oxidative hemolysis, which was higher in donors aged 18–30 years of age versus those over 60. In this edition of TRANSFUSION, Cloutier together with colleagues from the BEST Collaborative now extend that age bracket.10 They studied red cell concentrates from 75 teenage donors (16–19 years old), and compared these with ethnicity-matched donors that were 75 years of age or over. Here, they also found an increased susceptibility of red cells from teenage donors to oxidative hemolysis. However, unlike Kanias et al.,8 storage-induced hemolysis and osmotic hemolysis showed no differences. The elegance of the study lies in the age cohorts, that is, at the extremes of what is currently allowed. Many, if not all studies, focus on the average donor population with the majority of donors in their 40s or 50s, and certainly limit their observations to donors within the traditional cohort from 18 to 65. When looking at the extremes of the age spectrum of eligible blood donors, new information can be obtained. Other key findings of the study include that older donors have fewer but larger (higher MCV) red cells, so that total hemoglobin is not different between the age groups. Inflammatory markers, as a possible consequence of the storage lesion, showed no differences. The study was not designed or intended to explain why aging affects the in vitro quality of stored red blood cells. Various theories exist that try to explain the process of aging. It could be a genetic process where telomeres shorten with age.11 However, how shortened telomeres can explain in vitro differences in anucleate red cells, is uncertain. The observed differences could be programmed in our genes, but the biological meaning for the observed differences in red cells remains elusive. A third explanation is that of the oxidative stress theory.12 This theory states that organisms age due to the damage incurred because of free radicals over time. Reactive oxygen species (ROS) are formed during metabolic processes inside the cell. ROS are toxic, and so a system is in place with antioxidants to neutralize the ROS. It has been hypothesized that oxidative stress increases with age, which results in oxidized lipids, proteins, sugars, and nucleic acids, ultimately resulting in cellular dysfunction. Inal et al.13 showed in age groups ranging from 0.2 to 69 years of age that superoxide dismutase in red cells decreased with age, while catalase and glutathione peroxidase increased, suggesting an adaptive approach against the formation of hydrogen peroxide. In that same study, lipid peroxidation, measured as malondialdehyde concentration in plasma, was highest in the oldest age cohort.13 This is in line with the finding that the plasma membrane redox system in red cells increases with age.14 They found an age-related decrease in plasma antioxidant capacity. Furthermore, in older donors, there are more reticulocytes.15 Red cells are cleared more rapidly at older age, potentially because of binding of IgG to the band 3 protein, resulting in removal of the red cells from the circulation,16, 17 which could explain the higher percentage of reticulocytes in older donors. There is evidence for increased oxidative stress with age, including less reduced and more oxidized glutathione in red cells,18 likely caused by declining antioxidant enzyme activities with increasing age.19 A summary of the various changes with age is given in Table 1. How do we align these findings with those of Cloutier et al.10? Oxidative damage increases with increasing age, as can be derived from the increased lipid peroxidation.13, 18 Glutathione peroxidase increases with age,13 but the glutathione concentration itself is lower at older age.9, 18 It may be that, due to the increased oxidation, the red cells sensitive to oxidative hemolysis are already cleared from circulation in vivo, explaining why in the older donors, the sensitivity in the oxidative hemolysis assay is lower than in younger donors. The removal of red cells sensitive to oxidative hemolysis could also explain why older donors have more reticulocytes15 and have a higher MCV,10 as younger red cells are larger. In addition, older donors have an altered glycolysis, with a higher activation of the pentose phosphate pathway, and higher methionine metabolism.9 The pentose phosphate pathway generates NADPH, that can be used for the reduction of glutathione, to counteract the effects of the oxidative stress. Methionine plays a role in activation of antioxidant enzymes, including methionine sulfoxide reductase A, and the synthesis of glutathione.20 There are other explanations for the findings of Cloutier. One is donor selection. Older donors are likely to be recurring donors, and may have a different iron metabolism, or more reticulocytes, because they donate more often. Older donors may have undiagnosed or subclinical medical conditions explaining these results. Lifestyle factors, including smoking and diet, could be another possibility. Therefore, more studies are needed to elucidate why red cells of younger donors have a higher sensitivity to oxidative stress. The study was done as a multisite study in three large blood centers. On one hand, this substantiates the results as three sites independently, in their own donor cohort, show the same differences in oxidative hemolysis. However, a challenge of these multisite studies is interlaboratory variation. For example, are there truly differences in teenage male donor ferritin levels between Vitalant and Hema-Quebec? Is this merely a measuring error? Or is it because of the different ages between the cohorts (on average 16.4 years old at Vitalant and 18.8 in Hema-Quebec)? As Vinkenoog et al.21 showed, there is a steep increase in ferritin levels between age cohorts 18–24 and 25–34, particularly for males (from median 96 to 136 ng/mL), so this difference could well be real, but follow up studies could shed further light on this. Finally, are the findings of the effect of donor age on red cell quality important? There are studies that link donor age to clinical outcomes. In a large cohort study of over 30,000 patients receiving more than 80,000 red cell transfusions, Chassé et al.22 found a statistically significant 8% increase in death if the patients received blood from donors aged 17–19.9 years old, and 6% when the donors were between 20 and 29.9 years old, compared with those receiving red cells from donors from 40 to 49.9 years of age. Red cell transfusions from donors aged 30 or over showed no increased risk of mortality. Patel et al.23 studied the donor age effect in very low birthweight infants with a composite primary endpoint of death, necrotizing enterocolitis, retinopathy of prematurity, and bronchopulmonary dysplasia. They found an association between donor sex and age, with a relative risk of 0.29 if the donor was female compared with male, and there was a differentiation based on age: the risk decreased from 0.41 if the age of the female donor was in the first quartile (up to 38.6 years) to 0.18 if the female donor age was in the third quartile (older than 57.6 years). In other words, the risk of meeting the primary endpoint in these infants decreased with transfusions of red cells from older, female donors. However, others refute these findings. Vasan et al.24 found no effect of the age of blood donors on the 30 day and 1 year mortality. Edgren et al.25 initially found an association when their data were unadjusted, with a 12% increase in death if the donor was aged under 20, and a 25% increase if the donor was over 70, with no effect on mortality in the other age cohorts in between. However, when adjusted for the number of red cell transfusions, the effect disappeared entirely. They explained the finding of Chassé by residual confounding. With this conflicting evidence, and only a partial explanation why there are differences between the various studies, the effect of donor age on clinical outcomes remains uncertain, and again, more studies are needed. Last, the investigators only studied a limited set of quality parameters, and it could be that there are clinically relevant age-related differences in other parameters, such as ATP content, oxygen dissociation, or recovery after transfusion, and potentially specific blood bank-related procedures, such as sensitivity to lesions induced by irradiation or pathogen inactivation. The upper age for donor eligibility can be extended to a very old age, as long as the donor is healthy and does not experience adverse effects. With increasing age, the observed differences could be exacerbated, certainly in the context of a blood bank-stored red cell concentrate, and warrants further research to make sure that the blood products from these very old donors are of sufficient clinical efficacy, and do not induce adverse effects in the recipient. In summary, the intriguing findings of Cloutier et al.10 show that donor age is important for the in vitro quality of red cells stored under blood bank conditions. Various differences, related to oxidative stress and red cell hemopoiesis, are observed, but the underlying biological explanation is unknown, and requires further study. The clinical impact is currently uncertain, and with extension of the eligible donor age, could gain more importance. The authors have disclosed no conflicts of interest.
更多查看译文
关键词
red cells,donor age,blood bank conditions
AI 理解论文
溯源树
样例
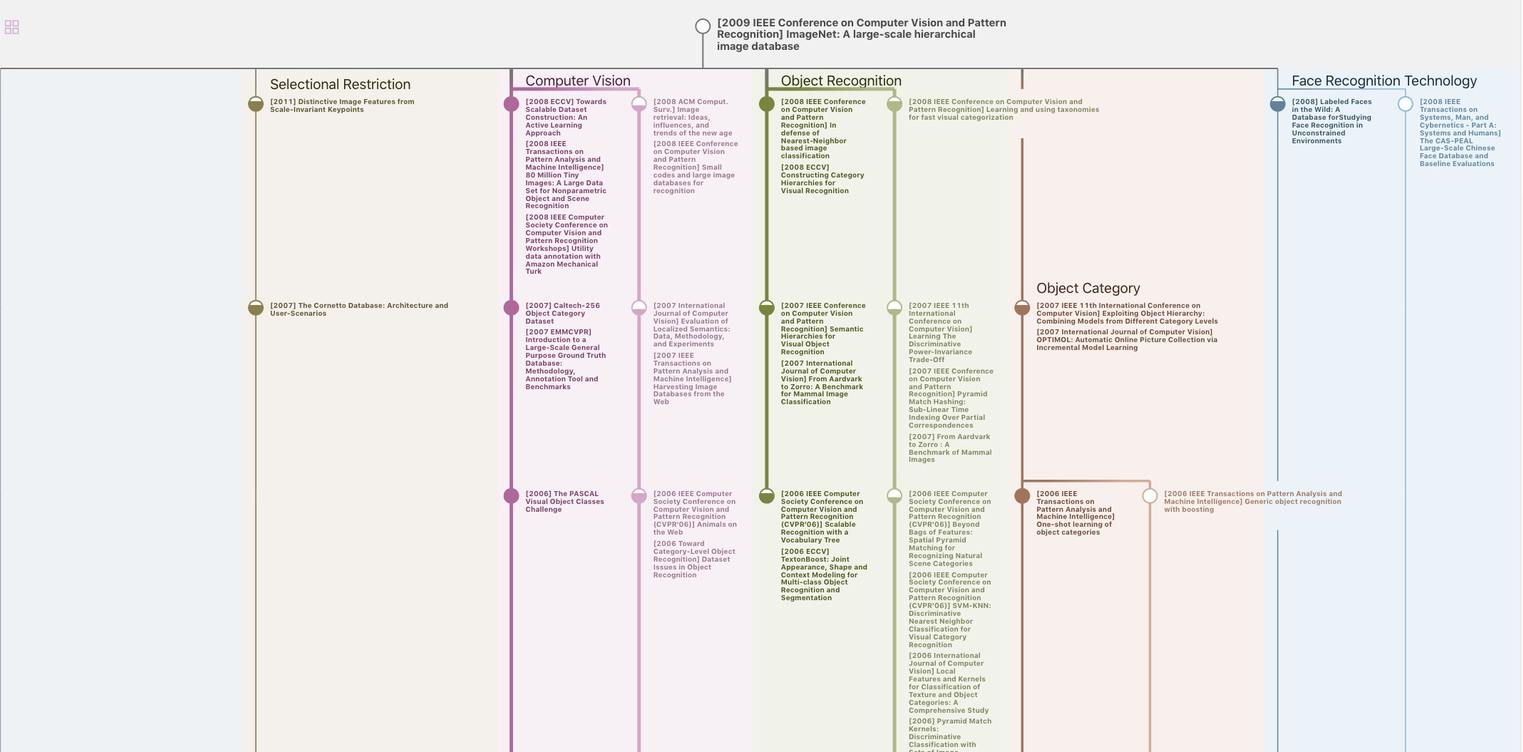
生成溯源树,研究论文发展脉络
Chat Paper
正在生成论文摘要