Author response: Pericentrin-mediated SAS-6 recruitment promotes centriole assembly
crossref(2019)
摘要
Article Figures and data Abstract Introduction Results Discussion Materials and methods Data availability References Decision letter Author response Article and author information Metrics Abstract The centrosome is composed of two centrioles surrounded by a microtubule-nucleating pericentriolar material (PCM). Although centrioles are known to regulate PCM assembly, it is less known whether and how the PCM contributes to centriole assembly. Here we investigate the interaction between centriole components and the PCM by taking advantage of fission yeast, which has a centriole-free, PCM-containing centrosome, the SPB. Surprisingly, we observed that several ectopically-expressed animal centriole components such as SAS-6 are recruited to the SPB. We revealed that a conserved PCM component, Pcp1/pericentrin, interacts with and recruits SAS-6. This interaction is conserved and important for centriole assembly, particularly its elongation. We further explored how yeasts kept this interaction even after centriole loss and showed that the conserved calmodulin-binding region of Pcp1/pericentrin is critical for SAS-6 interaction. Our work suggests that the PCM not only recruits and concentrates microtubule-nucleators, but also the centriole assembly machinery, promoting biogenesis close by. https://doi.org/10.7554/eLife.41418.001 Introduction The centrosome is the major microtubule organizing center found in animals, being composed of two microtubule-based cylinders, the centrioles, which are surrounded by an electron-dense proteinaceous pericentriolar material (PCM) that nucleates microtubules. The centriole can also function as a basal body, nucleating cilia formation. Centrioles are normally formed in coordination with the cell cycle, one new centriole forming close to each existing one, within a PCM cloud (Bettencourt-Dias and Glover, 2007; Fu et al., 2015). The first structure of the centriole to be assembled is the cartwheel, a ninefold symmetrical structure, composed of SAS-6, CEP135/Bld10, STIL/Ana2/SAS-5, amongst others (Kitagawa et al., 2011; Lin et al., 2013; Nakazawa et al., 2007; Tang et al., 2011; van Breugel et al., 2011). This is followed by centriole elongation through the deposition of centriolar microtubules which is dependent on components such as CPAP/SAS-4 (Kohlmaier et al., 2009; Schmidt et al., 2009; Tang et al., 2009). Remarkably, SAS-6 and CEP135/Bld10 can self-assemble in vitro to generate a ninefold symmetrical cartwheel (Guichard et al., 2017). In vivo, active PLK4 is known to recruit and phosphorylate STIL, which then recruits SAS-6 (Arquint et al., 2012; Arquint et al., 2015; Moyer et al., 2015; Ohta et al., 2014). In a process called ‘centriole-to-centrosome conversion’, the centriole recruits centriole-PCM linkers, such as CEP192/SPD2 and CEP152/asterless, which contribute to both centriole biogenesis and PCM recruitment (Cizmecioglu et al., 2010; Dzhindzhev et al., 2010; Hatch et al., 2010; Sonnen et al., 2013). Finally, CDK5RAP2/CNN and pericentrin are recruited, which then recruit and activate γ-tubulin and create an environment favorable for concentrating tubulin, leading to the formation of a proficient matrix for microtubule nucleation and organization (Delaval and Doxsey, 2010; Feng et al., 2017; Megraw et al., 2011; Woodruff et al., 2017). The cascade of phosphorylation and interaction events between centriole components leading to centriole biogenesis is an intricate succession of positive feedback interactions. That circuit leads to amplification of an original signal present at the centriole, such as the presence of active PLK4, or its substrate STIL, hence perpetuating centriole biogenesis there (Arquint and Nigg, 2016; Lopes et al., 2015; Moyer et al., 2015). Additionally, the already existing ‘older’ centriole is surrounded by PCM, which could help to localize and concentrate centriole components during centriole assembly, reinforcing centriole formation close by. In support of this idea, when centrioles are eliminated in human cells by laser ablation, they form de novo within a PCM cloud (Khodjakov et al., 2002). Moreover, exaggeration of the PCM cloud by overexpressing a PCM component, pericentrin, in S-phase-arrested CHO cells, induces the formation of numerous daughter centrioles (Loncarek et al., 2008). Although pericentrin is not shown to be implicated in centriole biogenesis per se to date, dissociation of another pericentrin-related protein, AKAP450, from the centrosome, interferes with centriole duplication in human cells (Keryer et al., 2003). Moreover, PCM components, γ-tubulin and Spd-5, a functional analogue of Drosophila CNN and Hs Cdk5rap2, contribute to centriole assembly in C. elegans (Dammermann et al., 2004). Downregulation of the PCM in Drosophila, has been shown to lead to: i) fragmented and short centrioles, in the case of pericentrin (PLP) (Martinez-Campos et al., 2004; Roque et al., 2018), ii) disengaged centrioles, in the case of CNN (Lucas and Raff, 2007; Megraw et al., 2001), and iii) centriole disassembly, in the case of the removal of all PCM components (Pimenta-Marques et al., 2016). It is thus likely that the PCM plays critical roles in assembling and/or maintaining centriole structures. Despite the possible importance of the PCM, it is difficult to study its roles on centrioles due to confounding effects of the many centriole-centriole component interactions that exist in centriole-containing animal cells. To define the contribution of PCM in regulating centriole components, we explored a system that has no endogenous centriole components, taking advantage of the diverged centrosomes observed in nature. While centrioles are ancestral in eukaryotes, they were lost in several branches of the eukaryotic tree, concomitantly with the loss of the flagellar structure (Carvalho-Santos et al., 2010). Instead of a canonical centrosome, yeasts have a spindle pole body (SPB), a layered structure composed of a centriole-less scaffold that similarly recruits γ-tubulin and other PCM components and nucleates microtubules (Cavanaugh and Jaspersen, 2017). The timing and regulation of SPB biogenesis are similar to the one observed in animal centrosomes (Lim et al., 2009; Kilmartin, 2014; Rüthnick and Schiebel, 2016). It is likely that the animal centrosome and yeast SPB evolved from a common ancestral structure that had centrioles (Figure 1A), as early-diverged basal fungi such as chytrids (e.g. Rhizophydium spherotheca) have a centriole-containing centrosome (Powell, 1980). By expressing animal centriole components in fission yeast, we observed that the SPB recruits them. We further demonstrate that the SPB conserved PCM component Pcp1/pericentrin recruits the centriole component SAS-6. We further validated this interaction in animals and show it is important for centriole elongation. Our work reveals an important role for pericentrin in recruiting centriole components and regulating centriole structure in animals. Figure 1 Download asset Open asset Evolution of the morphology and protein content of animal and fungi centrosomes. (A) The structure of the centrosome in mitosis in most animals, chytrids (flagellated fungi) and fission yeast. Animals and chytrids have a centriole/basal body and a canonical centrosome composed of a pair of centrioles surrounded by PCM, which anchors and nucleates microtubules. Fission yeast lacks a centriole but has a spindle pole body (SPB) inserted in the nuclear envelope. The SPB nucleates microtubules from the PCM-like structure, inside the nucleus. Parsimoniously, it is likely that the common ancestor of animals and fungi had a centriole-containing centrosome with a PCM structure (model shown). (B) Phylogenetic distribution of centrosome components in opisthokonts (animals, fungi and choanoflagellates). We searched for orthologues of components of the human centrosome localizing to centrioles, centriole-PCM linkers and PCM, and the fission yeast SPB components. Black circles represent the presence of orthologues that were identified by the bidirectional best hit approach to the human or fission yeast proteins, respectively; gray circle represents the presence of a putative orthologue identified by constructing phylogenetic trees; blue circles indicate that previous studies showed the presence of a protein with short conserved motifs (Kilmartin, 2003; Samejima et al., 2010; Lin et al., 2014) although we failed to identify it by the computational methods highlighted above; white circles indicate no detectable orthologue. https://doi.org/10.7554/eLife.41418.002 Figure 1—source data 1 List of the predicted orthologues of the human centrosome and S. pombe SPB components in animal and fungi species. https://doi.org/10.7554/eLife.41418.003 Download elife-41418-fig1-data1-v1.xlsx Results We first investigated the conservation of centrosome components, searching for orthologues of the known animal proteins comprising: components of centrioles that are required for centriole biogenesis (SAS-6, STIL/Ana2/SAS-5, CPAP/SAS-4, CEP135/Bld10 and CEP295/Ana1), linkers of the centriole to the PCM, which are bound to the centriole and are required for PCM recruitment (CEP152 and CEP192), and the PCM itself, which is involved in γ-tubulin recruitment and anchoring (pericentrin, Cdk5rap2 and γ-tubulin itself). In addition, to better understand when the SPB originated, we also searched for orthologs of the fission yeast SPB components: the core scaffold proteins (Ppc89, Sid4, Cdc11, Cut12 and Cam1; Bestul et al., 2017; Chang and Gould, 2000; Krapp et al., 2001; Moser et al., 1997; Rosenberg et al., 2006) and the half-bridge proteins (Sfi1 and Cdc31; Kilmartin, 2003; Paoletti et al., 2003), which are required for SPB duplication. Consistent with previous studies (Carvalho-Santos et al., 2010; Hodges et al., 2010), the proteins required for centriole biogenesis in animals were not identified in the fungal genomes, with exception of chytrids, which have centrioles (Figure 1B). Centriole-PCM connectors were not found in both chytrids and yeasts. In contrast, when investigating the PCM composition, pericentrin and Cdk5rap2 were found in all fungal species, although the budding yeast Spc110 and Spc72 only share short conserved motifs with pericentrin and Cdk5rap2, respectively (Lin et al., 2014). Regarding the SPB components, we found that Cam1 and Cdc31 are both highly spread across opisthokonts, which may reflect a conserved module or, alternatively an MTOC-independent conserved function of these proteins. On the other hand, we could only find proteins such as Ppc89 and Sid4 in yeasts, but not in chytrids, suggesting that some of the yeast-specific structural building blocks of the SPB appeared after branching into yeasts (Figure 1B). Altogether, these results suggest the yeast centrosome is very different from the animal canonical centrosome, not having centriole and centriole-PCM adaptors, and being composed of several yeast-specific SPB components. Our results suggest that different proteins are involved in assembling different modules of the centrosome and that they can be lost when that module is lost, leading to a divergence of the remaining structures. Importantly, while centriole components were lost, the PCM module is conserved in animals and fungi in terms of composition and function, establishing fission yeast as an interesting system to study the interaction between components of the centriole and the PCM. We thus expressed key animal centriole components in fission yeast and asked whether they would interact with the PCM at the SPB or other yeast microtubule organizing centers. Animal centriole components localize to the fission yeast SPB We used individual Drosophila centriole components as they are well-characterized and tested their localization when expressed in fission yeast. We chose five critical components, SAS-6, CEP135/Bld10, CPAP/SAS-4, STIL/Ana2/SAS-5 and PLK4 for the test. All genes coding for these proteins are absent from the yeast genomes (Figure 1B). Fission yeast SPBs are easily recognizable under light microscopy with fluorescent protein-tagged SPB marker proteins, such as Sid4 and Sfi1 (Chang and Gould, 2000; Kilmartin, 2003), which show distinct localization as a clear focus. Therefore, we examined if the animal centriole proteins could recognize and thus localize to the SPB. GFP or YFP-tagged Drosophila centriole proteins were heterologously expressed under control of the constitutive atb2 promoter (Matsuyama et al., 2008) or the inducible nmt1 promoter (Maundrell, 1990) in fission yeast. Despite the one billion years separating yeasts from animals (Douzery et al., 2004; Parfrey et al., 2011), SAS-6-GFP, Bld10-GFP and YFP-SAS-4 co-localized with fission yeast Sid4 to the SPB (Figure 2A and B). In addition to the localization on the SPB, YFP-SAS-4 signal was also weakly observed along interphase cytoplasmic microtubules, likely reflecting its microtubule-binding capacity (Gopalakrishnan et al., 2011). In contrast, GFP-Plk4 and YFP-Ana2 did not localize to the SPB and existed as foci in the cytoplasm (Figure 2A and B). We confirmed the expression of the fusion proteins with the expected sizes (Figure 2—figure supplement 1A). Cells expressing centriole proteins which localize to the SPB grew as well as control cells, which have no centriole protein (Figure 2—figure supplement 1B), suggesting their expression does not impair yeast growth. Figure 2 with 1 supplement see all Download asset Open asset Drosophila centriole proteins localize to the centrosome of fission yeast. (A) SAS-6, Bld10 and SAS-4 localize to the SPBs, while Ana2 and Plk4 do not (see Materials and methods for details on expression constructs). Scale bar, 5 μm. (B) Quantification of cells with GFP or YFP-tagged centriole proteins localized on the SPB. Data are the average of three experiments ± s.d. (N > 50, GFP/YFP-positive cells). (C) Physical interaction between the centriole proteins and fission yeast Pcp1. Protein extract was prepared from fission yeast cells expressing HA-tagged Pcp1 and either SAS-6-GFP, or Bld10-GFP, or YFP-SAS-4 or GFP. The GFP-tagged proteins were immunoprecipitated with anti-GFP antibody. Immunoprecipitates and inputs (4%) were analyzed by western blotting using the indicated antibodies. Red open and filled arrowheads indicate the bands of Pcp1-HA and GFP/YFP fusion proteins, respectively. https://doi.org/10.7554/eLife.41418.004 Figure 2—source data 1 The source data to plot the graph in Figure 2B. https://doi.org/10.7554/eLife.41418.006 Download elife-41418-fig2-data1-v1.xlsx The surprising result that SAS-6, Bld10 and SAS-4 independently localize to the SPB, suggests that one or more fission yeast SPB component can recruit them. These results indicate that SPBs and canonical centrosomes have diverged less than what would be expected from their diverse morphology and divergent protein composition. Fission yeast pericentrin, Pcp1, interacts with centriole proteins Given the conservation of PCM components (Figure 1B), we wondered whether fission yeast PCM components localized on the SPB could be recruiting the Drosophila centriole components. We first examined the interaction between the centriole proteins (SAS-6, Bld10 and SAS-4) and Pcp1, the fission yeast pericentrin ortholog, which recruits the γ-tubulin ring complex (γ-TuRC) to regulate mitotic spindle formation (Fong et al., 2010). In animals, pericentrin is a key component of the PCM, extending with its C-terminus at the centriole wall into the PCM (Lawo et al., 2012; Mennella et al., 2012). Surprisingly, we found that SAS-6, Bld10 and SAS-4, all interacted with Pcp1 as revealed by co-immunoprecipitation (Figure 2C). Fission yeast Pcp1 is required for SAS-6 recruitment Next, we examined if Pcp1 is required for localization of SAS-6, Bld10 and SAS-4 on the SPBs using a temperature-sensitive mutant of Pcp1 (pcp1-14), in which the amount of Pcp1 protein is already reduced, and further reduced when grown at the restrictive temperature (Tang et al., 2014). These cells also arrest in mitosis when at the restrictive temperature. To compare the signal intensity in cells at the same cell cycle stage, mitosis, we introduced the cut7-446 allele both in wild-type and pcp1-14 background. Cut7 is a mitotic kinesin, its mutation fails in interdigitating the mitotic spindle and causes the cells to arrest in early mitosis (Hagan and Yanagida, 1990). Hereafter, we refer to the strains with cut7-446 and pcp1-14 cut7-446 alleles, as the control and pcp1 mutant, respectively. The intensity of SAS-6-GFP per SPB was significantly increased in control cells when arrested in prometaphase (Figure 3A and B). We observed reduced SAS-6-GFP intensity in the pcp1 mutant both at the permissive and restrictive temperatures (Figure 3A and B). This indicates that Pcp1 is required for SAS-6 recruitment to the SPB. Unlike SAS-6, Bld10-GFP intensity was not reduced, but slightly increased in the pcp1 mutant (Figure 3C and D). It is possible that Bld10 is recruited to the SPB by another SPB component(s) which is upregulated in the pcp1 mutant. Although the intensity of SAS-4 was reduced in the pcp1 mutant (Figure 3E and F), we found that the total protein of YFP-SAS-4 was also lower in the pcp1 mutant while that of SAS-6-GFP and Bld10-GFP was comparable in control and pcp1 mutant lysates (Figure 3G). We think that YFP-SAS-4 might be stabilised by Pcp1 in fission yeast cells, and therefore we cannot conclude whether Pcp1 is required for YFP-SAS-4 localization on the SPB. Since SAS-6 is such a critical component in centriole assembly, and its localization is determined by Pcp1, we decided to explore further how SAS-6 is recruited to the SPB by Pcp1. Figure 3 Download asset Open asset Fission yeast pericentrin-like protein Pcp1 is required to recruit SAS-6 to the SPB. (A, C, E) SAS-6-GFP, Bld10-GFP and YFP-SAS-4 intensities on the SPB in the pcp1 mutant in asynchronous and prometaphase-arrested cells. The cut7-446 (labeled ‘control’) and cut7-446 pcp1-14 (labeled ‘pcp1 mutant’) strains expressing SAS-6-GFP and Sid4-tdTomato were incubated at the restrictive temperature (36°C) for three hours to block cells in prometaphase due to the cut7-446 mutation (temperature-sensitive allele of a mitotic kinesin, which causes failure in mitotic spindle formation). Representative images of the cells collected before shifting the temperature (interphase, Int) and three hours after the shift to 36°C (prometaphase, PM, restrictive temperature) in control and pcp1 mutant are shown. DNA was stained with DAPI. Arrowheads indicate the signal on the SPB. Note that we also observed aggregation of SAS-6-GFP and YFP-SAS-4 in the cytoplasm both in the control and pcp1 mutant in all the cells exposed to the restrictive temperature (indicated with an asterisk), and never at the normal and permissive culture condition. We think that aggregate formation might stem from the cut7-446 genetic background and/or the heat stress. Scale bar, 5 μm. (B, D, F) Quantification of the intensity of the centriole proteins per SPB in the indicated conditions. Means ± s.d. are shown in red (N > 100 SPBs, ns-not significant, *p<0.05, **p<0.001, ***p<0.0001, Mann-Whitney U test). (G) Western blotting analysis of protein extracts prepared from the indicated conditions. https://doi.org/10.7554/eLife.41418.007 Figure 3—source data 1 The source data to plot the graphs in Figure 3B,D,F. https://doi.org/10.7554/eLife.41418.008 Download elife-41418-fig3-data1-v1.xlsx It is known in Drosophila and human cells that phosphorylation and interaction of PLK4 with STIL/Ana2, facilitates STIL recruitment to the centriole and its interaction and recruitment of SAS-6 (Arquint et al., 2012; Moyer et al., 2015; Ohta et al., 2014; Vulprecht et al., 2012). However, neither Plk4 nor STIL are present in the fission yeast genome (Figure 1B), suggesting that Pcp1/pericentrin is part of an additional and ancestral molecular pathway for SAS-6 recruitment. SAS-6 interacts with the conserved region of Pcp1, and Pcp1 is sufficient to recruit SAS-6 We reasoned that if the interaction between SAS-6 and Pcp1/pericentrin is an ancient and conserved connection, they should interact through an evolutionarily conserved domain in Pcp1. Subsequently, we determined which part of Pcp1 is required for its interaction with SAS-6. Full-length and truncation mutants of Pcp1 (N, M and C) were co-expressed with SAS-6-GFP (Figure 4A). Only full-length Pcp1 and its C-terminal region containing the conserved PACT domain interacted strongly with SAS-6 (Figure 4B). Given that the Pcp1-M fragment was weakly expressed, we cannot exclude completely the possibility that it also interacts with SAS-6-GFP (Figure 4B). The PACT domain localizes to the centriole wall in animals and is required for MTOC targeting both in animal and fungi (Gillingham and Munro, 2000). Figure 4 with 3 supplements see all Download asset Open asset SAS-6 interacts with Pcp1 through the conserved carboxyl-terminal region, and Pcp1 is sufficient for SAS-6 localization. (A) Schematic illustration of the truncation constructs of Pcp1. (B) Pcp1 interacts with SAS-6 through the conserved carboxyl-terminal region. mCherry-tagged full-length or truncation mutants of Pcp1 were expressed in cells constitutively expressing SAS-6-GFP. Immunoprecipitation was performed and analyzed similarly as in Figure 2B. Red arrowheads indicate bands with the expected size of each fusion protein. The asterisk indicates non-specific bands. (C, D) Pcp1 is sufficient to recruit SAS-6. Overexpression of Pcp1 leads to the formation of Pcp1 containing cytoplasmic foci (schematic illustration, (C). Pcp1-mCherry was overexpressed under control of nmt41 promoter in the strain expressing SAS-6-GFP and GFP alone. Sfi1-CFP is shown (SPB marker). Arrowheads indicate the SAS-6-GFP signal on the Pcp1-cytoplasmic foci. Scale bar, 5 μm. (E) Quantification of the cells with colocalized Pcp1-mCherry and GFP foci. Data are the average of three experiments ± s.d. (N > 50, Pcp1-mCherry positive cells). https://doi.org/10.7554/eLife.41418.009 Figure 4—source data 1 The source data to plot the graph in Figure 4E. https://doi.org/10.7554/eLife.41418.016 Download elife-41418-fig4-data1-v1.xlsx We next asked whether Pcp1 could recruit SAS-6 ectopically. It is known that Pcp1 overexpression forms multiple Pcp1-containing foci in the cytoplasm (Flory et al., 2002). We thus examined if the cytoplasmic Pcp1 foci recruit SAS-6 (illustrated in Figure 4C). Overexpressed mCherry-tagged Pcp1 recruited SAS-6-GFP, but not GFP (control), to such foci (Figure 4D and E), indicating that Pcp1 can ectopically recruit SAS-6. Though epifluorescence micrographs suggest SAS-6 co-localizes with Sid4 and Pcp1 (Figures 2 and 4), due to the resolution limit of a conventional optical microscope (~200 nm), we failed to conclude precisely where SAS-6 localizes to. To further determine the precise localization of SAS-6, we analyzed the relative position of SAS-6-GFP with respect to core SPB components Pcp1-tdTomato or Sid4-tdTomato (Figure 4—figure supplement 1A) by structured illumination microscopy (SIM). The foci of Pcp1-tdTomato and Sid4-tdTomato within the duplicated SPBs were distinguishably separated (60 ± 10 nm) (Figure 4—figure supplement 1B and C). Importantly, SAS-6-GFP center of mass localizes at ~50 nm distance from both Pcp1-tdTomato and Sid4-tdTomato center of mass. Given that the diameter and height of SPB is 180 nm and 90 nm respectively (Ding et al., 1997), our data suggest that ectopically expressed SAS-6-GFP localizes to the core of the SPB. Regulation of SAS-6 localization to the SPB To further understand how SAS-6 is recruited to the SPB, we asked which of its domains are required for that localization. It has been reported that the coiled-coil region of human SAS-6 is sufficient for its localization to the animal centriole. This process is mediated by its interaction with other centriole components, such as STIL and CEP135 (Keller et al., 2014; Moyer et al., 2015). We expressed full-length SAS-6 and truncation mutants N-terminal (aa 1–176) and C-terminal (aa 177–472) and asked which co-localized with the SPB marker Sid4 (Figure 4—figure supplement 2A). Similar to what happens in animals, the C-terminal coiled-coil region of SAS-6 is required for its localization at the SPB (Figure 4—figure supplement 2B,C and D). To approach the timing of SAS-6 targeting to the SPB, we asked when it would be targeted during the cell cycle. Firstly, we confirmed that SAS-6 expression under atb2 promoter was constant throughout the cell cycle in synchronized cells (Figure 4—figure supplement 3A and B). We observed that SAS-6 accumulated at the SPB before entering mitosis, similar to its described regulation in animals, and also similar to the reported recruitment of Pcp1 to the SPB at that cell cycle stage in fission yeast (Keller et al., 2014; Strnad et al., 2007; Wälde and King, 2014). Conservation of the Pcp1/pericentrin - SAS-6 interaction Our experiments suggest that there are conserved interactions between centrioles and pericentrin, whose evolution is constrained. They further suggest that pericentrin has an important role in recruiting centriole components. To test our prediction, we examined in animal cells whether pericentrin interacts with SAS-6 and helps to recruit it to the centriole. The pericentrin family varies in protein length but contains the conserved PACT domain in the C-terminal region (Figure 5A). Since we observed that fission yeast Pcp1 interacts with SAS-6 through the PACT-domain containing region, we tested whether SAS-6 interacts with the PACT domain of Drosophila pericentrin ortholog, pericentrin-like protein (PLP). EGFP-tagged SAS-6 and HA-tagged PLP fragment containing the conserved PACT domain were co-expressed in Drosophila melanogaster tissue cultured cells (D.Mel cells). Consistent with the results obtained in fission yeast, SAS-6 interacts with the PACT domain (Figure 5B). To verify whether this interaction is direct, we validated this result in vitro, by performing in vitro binding assays using purified GST, GST-tagged SAS-6 and His-tagged PACT. GST-SAS-6 was specifically bound to His-PACT, indicating a direct interaction between these two proteins (Figure 5C). Figure 5 Download asset Open asset The Drosophila pericentrin (PLP) conserved domain –PACT- interacts with SAS-6, and its overexpression causes centriole amplification. (A) Schematic illustration of human pericentrin, Drosophila PLP and S. pombe Pcp1. (B) Physical interaction between SAS-6-EGFP and the conserved Drosophila PACT domain. Protein extract was prepared from Drosophila tissue culture cells (D.Mel cells) expressing HA-tagged PACT, and SAS-6-EGFP or EGFP. The GFP-tagged proteins were immunoprecipitated with anti-GFP antibody. Immunoprecipitates and inputs (20%) were analyzed by western blotting using the indicated antibodies. (C) Direct binding between SAS-6 and PACT. The in vitro binding assay was performed using purified GST or GST-fused SAS-6 and His-tagged PLP PACT. Note that we loaded 100% for each sample on each lane after pull-down to compare the efficiency of direct binding (bound vs input). Quantification of His-PACT bound to the GST-fusion protein is shown below the panel (data are the average of three experiments ± s.d) (D) Cells overexpressing EGFP-PACT or EGFP were arrested in mitosis by colchicine treatment for six hours and stained with the antibodies against Bld10 (centriole marker), phospho-H3 (mitotic marker) and SAS-6. Scale bar, 5 μm. (E) Quantification of SAS-6 intensity per centriole in cells overexpressing EGFP-PACT or EGFP arrested in mitosis. Means ± s.d. are shown in red (**p<0.001, Mann-Whitney U test). Results are representative of three independent experiments (N > 40 centrioles for each condition). (F) Cells overexpressing EGFP-PACT or EGFP were stained for two centriole markers (SAS-4 and Bld10, SAS-4 and Ana1) to count centriole number. Scale bar, 5 μm. (G) Quantification of centriole number per cell (N > 50, EGFP-positive cells). Data are the average of three experiments ± s.d. (*p<0.05, Mann-Whitney U test). Note that although control Drosophila tissue culture cells already show cells with underduplicated and over-duplicated centrioles as published before (Bettencourt-Dias et al., 2005), the expression of PACT leads to a significant amplification of centrioles. https://doi.org/10.7554/eLife.41418.017 Figure 5—source data 1 The source data to plot the graphs in Figure 5E and G. https://doi.org/10.7554/eLife.41418.018 Download elife-41418-fig5-data1-v1.xlsx The conserved SAS-6-pericentrin interaction plays a role in centriole assembly Pericentrin is highly expressed and correlates with the levels of centrosome aberrations in acute myeloid leukemia (AML) (Krämer et al., 2005; Neben et al., 2004). Moreover, overexpression of pericentrin in S-phase-arrested CHO cells induces the formation of numerous daughter centrioles (Loncarek et al., 2008). We thus wondered whether those effects were mediated by excessive SAS-6 recruitment since SAS-6 overexpression leads to supernumerary centriole formati
更多查看译文
关键词
centriole assembly,pericentrin-mediated
AI 理解论文
溯源树
样例
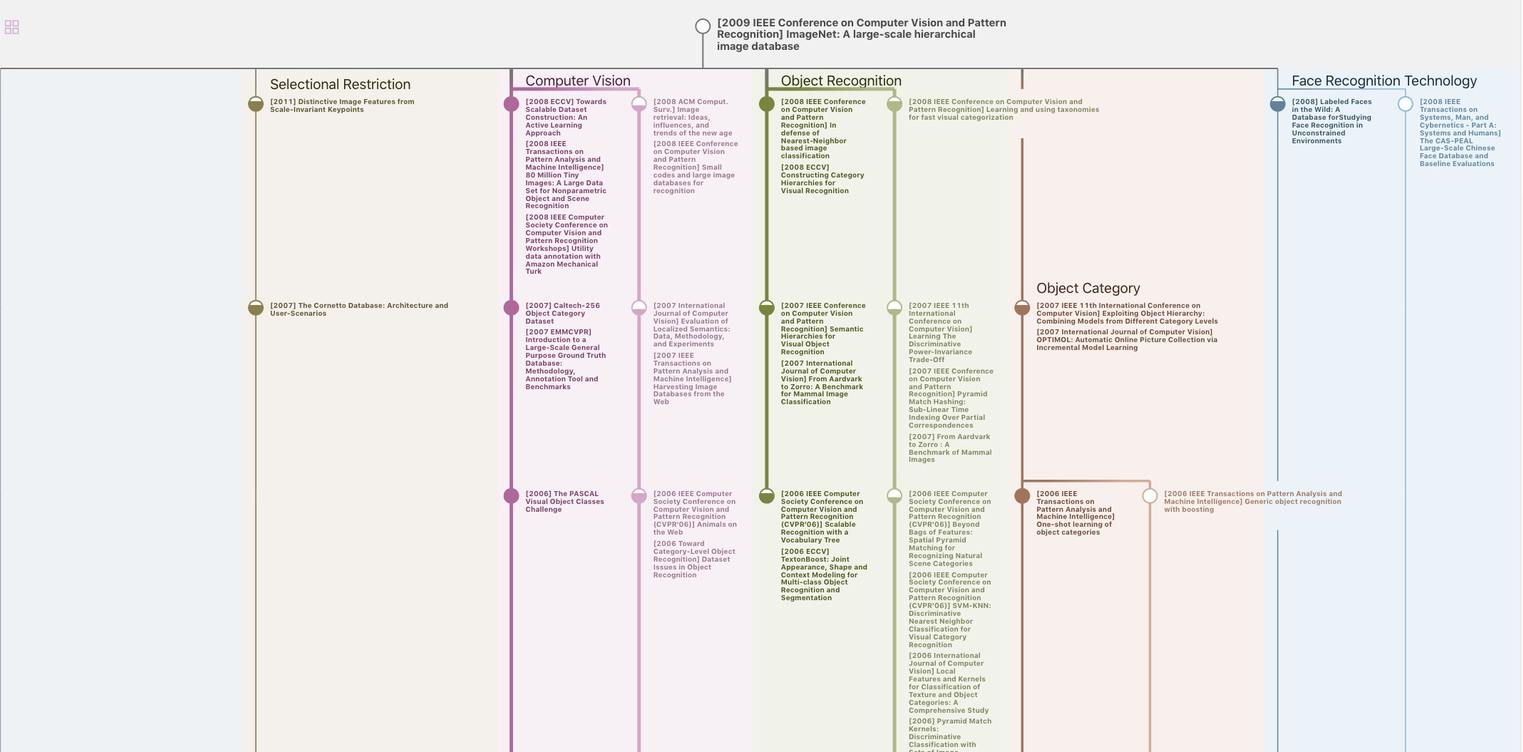
生成溯源树,研究论文发展脉络
Chat Paper
正在生成论文摘要