Author response: Antagonism between parasites within snail hosts impacts the transmission of human schistosomiasis
crossref(2019)
摘要
Article Figures and data Abstract Introduction Results Discussion Materials and methods Data availability References Decision letter Author response Article and author information Metrics Abstract Human disease agents exist within complex environments that have underappreciated effects on transmission, especially for parasites with multi-host life cycles. We examined the impact of multiple host and parasite species on transmission of the human parasite Schistosoma mansoni in Kenya. We show S. mansoni is impacted by cattle and wild vertebrates because of their role in supporting trematode parasites, the larvae of which have antagonistic interactions with S. mansoni in their shared Biomphalaria vector snails. We discovered the abundant cattle trematode, Calicophoron sukari, fails to develop in Biomphalaria pfeifferi unless S. mansoni larvae are present in the same snail. Further development of S. mansoni is subsequently prevented by C. sukari’s presence. Modeling indicated that removal of C. sukari would increase S. mansoni-infected snails by two-fold. Predictable exploitation of aquatic habitats by humans and their cattle enable C. sukari to exploit S. mansoni, thereby limiting transmission of this human pathogen. Introduction Infectious diseases exist in complex ecological settings and for some disease agents, successful transmission may require sequential colonization of multiple obligatory hosts. This is particularly important for digenetic trematodes because obligatory hosts must overlap in space and time and in sufficient numbers for transmission to be successful (Dobson, 2004; Begon, 2008; LoGiudice et al., 2008). In addition, the environment may harbor a diversity of host species, some of which might serve as alternative competent hosts thus potentially favoring parasite persistence, or conversely, such species may act as non-productive sinks for the parasite thereby diminishing its transmission. As shown by an increasing number of studies, the net effect of biotically complex environments on transmission success for a particular parasite may be hard to predict, particularly for parasites that use multiple hosts to complete their life cycles (Lafferty et al., 1994; Suzán et al., 2009; Lafferty, 2012; Johnson et al., 2013; Salkeld et al., 2013; Rohr et al., 2015; Frainer et al., 2018). Furthermore, the impact may be highly contextual, depending on the density of host species, leading to dilution or amplification of transmission (Luis et al., 2018). Another factor influencing the success of a particular parasite is the diversity of other infectious agents present that colonize the same host species, thereby setting up the potential for within-host interactions. Such interactions can range from mutual dependence or facilitation to overt competition and even predation of one parasite by another (Lim and Heyneman, 1972; Combes, 1982; Lafferty et al., 1994; Hechinger et al., 2011). The purpose of this study is to combine field observations, experimental infections and use of a mathematical model parameterized by our empirical data to understand how transmission of the widespread human-infecting trematode Schistosoma mansoni is influenced by other trematode species present in the aquatic habitats in and around Lake Victoria. We are particularly interested in those trematode species that share with S. mansoni a dependence on Biomphalaria snails for their larval development. These additional Biomphalaria-dependent trematodes also infect a variety of wild or domestic host species to complete their respective life cycles. Therefore, strong interactions occurring among trematode species in the snail host could have cascading effects on the level of parasitism occurring in many host species, including humans and domestic animals. S. mansoni is the most geographically widespread causative agent of intestinal schistosomiasis, and the Lake Victoria Basin is one of the largest hyperendemic areas of schistosomiasis in the world (Gouvras et al., 2017; Wiegand et al., 2017). Despite repeated treatments with the anthelmintic praziquantel, children living in villages near the lakeshore often exhibit prevalence of S. mansoni infection of >50%, and up to 90% in some areas (Woodhall et al., 2013). A textbook-like portrayal of the S. mansoni life cycle highlighting the role of humans and a generic ‘Biomphalaria’ in transmission belies a more complex reality in the Lake Victoria Basin in that multiple options exist for transmission. With respect to mammalian definitive hosts, humans are certainly the most important in maintaining transmission (Colley et al., 2014), but baboons and common rodents like Mastomys natalensis can also play a role and may assume greater significance in ongoing chemotherapy-based control programs targeting human transmission (Hanelt et al., 2010; Catalano et al., 2018). Likewise, S. mansoni in and around the lake also infects three Biomphalaria taxa that exist in different habitats. Schistosoma mansoni eggs are passed into aquatic habitats in human feces. A miracidium hatches from an egg and if successful in finding and penetrating a Biomphalaria snail, then initiates a long-term, proliferative period of asexual development. This development culminates in the production of human-infective cercariae which are released or ‘shed’ into the surrounding water. These obligatory vector hosts include B. pfeifferi in streams and small impoundments, B. sudanica along the lakeshore, and B. choanomphala, now generally considered to be a distinct ecophenotype of B. sudanica, found especially but not exclusively in deeper water (Magendantz, 1972; Standley et al., 2011; Zhang et al., 2018). All three species have been found naturally infected with S. mansoni, (Magendantz, 1972; Standley et al., 2011; Mutuku et al., 2017; Mutuku et al., 2019). In addition to exploiting multiple definitive and intermediate host species, S. mansoni is, like many infectious agents, influenced in a myriad of ways by the diverse species with which it co-occurs. For instance, several other non-host species of freshwater snails co-occur with Biomphalaria and may act as sinks for S. mansoni as its miracidia will not develop in these snail species. Likewise, there are other species of digenetic trematode species in Kenya with an obligatory dependence on Biomphalaria snails for their larval development and their larval stages may strongly interact with S. mansoni larvae for access to the resources offered by these snail hosts. It is well-known from other studies that complex interactions usually dominated by antagonism via predation (and by indirect antagonism) can occur amongst the larvae of trematode species sharing a particular individual snail, and these interactions often have predictable outcomes that can influence patterns of abundance among trematode species in particular communities (Fernandez and Esch, 1991; Lafferty et al., 1994; Soldánová et al., 2012; Mordecai et al., 2016). Relevant to considerations of intra-snail interactions is that not all larval trematodes have the same pattern of development within their host snail. Some like S. mansoni develop sac-like sporocysts, whereas others produce rediae possessing a mouth surrounded by a powerful sucker, and a gut (Schell, 1985). Rediae can move within the snail and may ingest host tissue, including gonadal tissue (Lim and Lie, 1969). For example, the rediae of echinostomes (Echinostomatoidea) in particular tend to be dominant in intramolluscan interactions, with some echinostome species able to produce small, motile rediae specialized for attacking and killing larvae of other trematodes species, including S. mansoni sporocysts (Lim and Heyneman, 1972; Moravec et al., 1974; Hechinger et al., 2011). Amphistome trematodes (Paramphistomoidea) also produce rediae, and these tend to have more complex antagonistic or facilitation interactions with other trematodes (Southgate et al., 1989; Spatz et al., 2012). Sporocyst-producing species including strigeids and schistosomes have also been shown to have more subtle indirect antagonistic effects on larvae of other trematodes mediated by as yet uncharacterized soluble factors (Basch et al., 1969). Given the complex nature of the guilds of trematodes, we found to be associated with Biomphalaria snails in Kenya, we hypothesized that some of these non-schistosome trematode species could negatively impact the development and transmission of S. mansoni in Biomphalaria. To better understand these interactions, we initiated a survey at two stream sites and one lake site in western Kenya to define the trematode diversity from Biomphalaria. Morphological features and molecular markers were used to aid explicit definition of trematode species diversity. We also used sequential observations and experimental infections to reveal the dominance hierarchy among the trematodes that use B. pfeifferi, the most widely distributed S. mansoni vector in Africa. The same approaches also enabled us to observe striking antagonistic interactions perpetrated on S. mansoni by the most common trematode recovered from our habitats, the cattle-transmitted amphistome Calicophoron sukari. We then integrated our field survey results and experimental studies into a mathematical model to quantify the influence of C. sukari on S. mansoni transmission. These studies collectively highlight the importance of animal husbandry practices and of a diverse community of wild animals and the trematodes they support, particularly those dependent on Biomphalaria, on downstream transmission of S. mansoni infection to people in west Kenya. Results Field surveys Upon screening Biomphalaria from both stream and lake habitats, several trematode species were found, verified as distinct taxa using morphological features and molecular markers (Figure 1a). A total of 19 species were found among 19,914 B. pfeifferi (overall prevalence 12.3%) from the perennial Asao Stream, 7 species from 1,136 B. pfeifferi (15.5%) from the highly seasonal Kasabong stream, and from Lake Victoria, 21 species of cercariae from 3,369 B. sudanica (10.3%), (Figure 1b). Some species, like S. mansoni, were recovered from all habitats, but many were habitat specific, such that in aggregate, 29 taxa were recovered. The life cycles of most of these trematodes have yet to be clarified, but by inferring probable life cycles from relatives with known life cycles (Yamaguti, 1958; Schell, 1985), a broad variety of invertebrate and wild and domestic vertebrate species are involved as hosts in supporting these life cycles (Figure 1c). A portion of the field-collected Biomphalaria were also exposed to S. mansoni to assess compatibility and we found that all three taxa of Biomphalaria were susceptible to S. mansoni, but found that B. pfeifferi was the most compatible (Figure 1d). At Asao and Kasabong, the most common trematode recovered from B. pfeifferi was the cattle-transmitted amphistome Calicophoron sukari (Laidemitt et al., 2017) (1,035/2439 total infections, or 42.4% of all infections at Asao, and at Kasabong 97/176, or 55.1% of all infections) followed closely by S. mansoni (1014 of 2439 total infections at Asao or 41.6% of all infections and at Kasabong, 68/176 or 38.6% of all infections) (Figure 1e). Both species were also commonly recovered from B. pfeifferi, but C. sukari was not recovered from B. sudanica or B. choanomphala. Also noteworthy is the overall prevalence of S. mansoni infections at stream sites (5.09% and 5.89%) was much higher than from the lakeshore (0.22%). Figure 1 Download asset Open asset Trematode biodiversity in the S. mansoni hyperendemic area of western Kenya. (A) The different types of trematode cercariae recovered either uniquely from B. pfeifferi from streams or from B. sudanica from the lakeshore, or that were recovered from both snail species (in red), confirmed with mitochondrial barcodes. (B) Kasabong (ephemeral stream) and Asao (perennial stream), the overall prevalence of larval trematode infections in B. pfeifferi is shown along with pie charts showing the composition of the trematode infections. Note the large proportions of infections comprised of C. sukari or S. mansoni. (C) A sample of six trematode with inferred life cycles (in some cases directly documented) to point out the variety of invertebrate and vertebrate hosts (and plants) involved in such cycles. (D) Peak prevalence of S. mansoni (indicated on vertical axis as the percent shedding cercariae) from three different Biomphalaria taxa to experimental infection with S. mansoni miracidia (five miracida/snail) derived from local school children. (E) The prevalence of C. sukari, S. mansoni, and other trematodes from bimonthly surveys of Kasabong and Asao. Note C. sukari is the most abundant trematode in both locations. The turtle, carp and frog images in panel C were reproduced from Pixabay (https://pixabay.com/photos/turtle-animal-wildlife-wild-nature-1517920/, https://pixabay.com/vectors/animal-carp-fish-freshwater-lake-2029698/, and https://pixabay.com/photos/tree-frog-anuran-frog-amphibians-299886/ respectively), under the terms of the Pixabay license (https://pixabay.com/service/license/). Dominance hierarchy By observing natural takeover events (Biomphalaria shedding one type of cercariae and then later shedding a different type of cercariae only) and through experimental infections, we discovered that at Asao Stream S. mansoni occupies an intermediate position in the hierarchy, and echinostomes and C. sukari were the more dominant species (Figure 2). In general, amphistomes of domestic ruminants like C. sukari were more commonly recovered from B. pfeifferi from stream sites whereas echinostomes, mostly transmitted by birds (Laidemitt et al., 2019), were more likely to be recovered from B. sudanica from the lakeshore. Figure 2 Download asset Open asset Trematode hierarchy in B. pfeifferi at Asao Stream. The dominance hierarchy was worked out through both experimental superinfections of snails with existing infections (green numbers), and by maintaining infected snails from the field to see if they switched from shedding one type of cercaria to another (orange numbers). Percentages shown are the prevalence of infected B. pfeifferi (shedding/total number of B. pfeifferi collected) for each group. We discovered that if B. pfeifferi from stream habitats that were not shedding cercariae of any kind at the time of collection were then exposed to S. mansoni miracidia, surprisingly they were almost as likely to subsequently shed C. sukari as S. mansoni cercariae (p<0.001) (Figure 3a). Control snails, otherwise similar except not exposed to S. mansoni, were subsequently much less likely to shed cercariae of either species. Figure 3 Download asset Open asset Human and cattle parasite interactions at Asao Stream. (A) Field-derived B. pfeifferi shown not to be shedding any cercariae at the time of collection were either left as unexposed controls or were exposed to S. mansoni (five miracidia/snail). Note that, unexpectedly, exposed snails were just as likely to subsequently shed C. sukari as S. mansoni cercariae compared to the control groups (p=<0.001). A few unexposed snails also shed cercariae, indicating that some of the snails had prepatent snails at the time of infection. (B) The prevalence of lab-reared B. pfeifferi exposed to various combinations of miracidia (see horizontal axis) of C. sukari and/or S. mansoni that subsequently shed cercariae of either species. Exposures to either species were with five miracidia/snail, 50 or 60 snails were used for each of 5 treatments for three separate experiments (total of 850 snails used). Separate ANOVAs were done for S. mansoni and C. sukari (each involving comparison of four groups), followed by pairwise comparisons. (C) Histological section of B. pfeifferi exposed to C. sukari for 8 days. Note the undeveloped sporocyst and the layer of hemocytes around it. (D) Histological section of B. pfeifferi exposed to S. mansoni for 8 days. The sporocyst has grown considerably and has developing daughter sporocysts within. C. sukari and S. mansoni Experimental Exposures Three additional experiments were undertaken with laboratory-bred F1 B. pfeifferi exposed to S. mansoni (originating from primary school children), to C. sukari (from cow dung samples collected from the banks of the streams), or to both species in various combinations (Figure 3b) and see methods. In control experiments, peak S. mansoni shedding was 7 weeks post exposure (86.7%, 92/106 surviving B. pfeifferi) and peak C. sukari shedding was at 8 weeks post exposure (0.68%, 1/114 surviving B. pfeifferi). In control experiments, S. mansoni shedding prevalence was 75.9% (63/83 surviving snails) 10 weeks post exposure and C. sukari shedding prevalence was 1.3% (1/79 of surviving snails). In the experiment ‘Sm first then two weeks later Cs,’ 24.2% (16/66) surviving B. pfeifferi shed S. mansoni and 21.2% (14/66) shed C. sukari cercariae. In the experiment ‘Cs then two weeks later Sm,’ 35.1% (26/74) of surviving B. pfeifferi shed C. sukari and 2.7% (2/74) shed S. mansoni cercariae. In the simultaneous experiment 9.2% (7/76) surviving B. pfeifferi shed S. mansoni and 15.8% (12/76) shed C. sukari cercariae. The results demonstrated that S. mansoni miracidia were capable of infecting B. pfeifferi on their own as expected (Mutuku et al., 2014), but C. sukari miracidia were poorly infective to B. pfeifferi on their own. From these co-exposure experiments the prevalence of B. pfeifferi shedding S. mansoni was significantly less than in the simultaneous (p=0.0478) and ‘Cs then two weeks later Sm’ experiments (p=0.0292) compared to S. mansoni controls. In comparison, C. sukari prevalence was significantly higher (p=0.0192) in the ‘Cs then two weeks later Sm group’. Examination of histological sections of B. pfeifferi taken 8 days after exposure to only C. sukari miracidia revealed the presence of sporocysts that had undergone little or no growth or development and had host hemocytes around them (Figure 3c). By contrast, S. mansoni sporocysts were much larger at the same age and germinal development was underway (Figure 3d). The success of C. sukari sporocysts in B. pfeifferi increased significantly (p=<0.0192) if S. mansoni miracidia were also allowed to infect the snails, particularly so if the S. mansoni exposures followed the exposure of snails to C. sukari. Importantly, this was accompanied by a sharp reduction in the number of snails shedding S. mansoni cercariae as compared to the snails exposed just to S. mansoni (Figure 3b). A further indication of the antagonistic interactions between the two trematode species was provided by the observation that although infections of both S. mansoni and C. sukari were common in B. pfeifferi in west Kenyan stream habitats, double infections (snails simultaneously shedding cercariae of both species) were rare, and occurred less than expected by chance (p=<0.001) (Figure 4a). Some field snails found to be naturally shedding S. mansoni cercariae would, upon further observation in the lab, permanently switch over to producing C. sukari cercariae instead. Similarly, exposure of field-collected snails shedding C. sukari cercariae to S. mansoni miracidia rarely resulted in conversion of the infections to production of S. mansoni cercariae. However, exposure of snails that were shedding S. mansoni cercariae to miracidia of C. sukari more often resulted in production of C. sukari cercariae. Lastly, our survey data also showed that numbers of S. mansoni and C. sukari infections were positively correlated (r = 0.622) (Figure 4b). This is not surprising, since C. sukari relies on S. mansoni for its own transmission. Figure 4 Download asset Open asset Field observations supported experimental results. (A) Graph showing the number of single infections of B. pfeifferi with either S. mansoni or C. sukari and the number of observed double infections, which was significantly fewer than the number of double infections expected by chance (p=<0.001). (B) Correlation between the abundance of S. mansoni and C. sukari infections (XY pairs = 25, Pearson r = 0.622, p=0.0009) in B. pfeifferi from our stream survey data (prevalence of each parasite from 25 collection time points). Mathematical model To estimate the potential impact of C. sukari on S. mansoni transmission, we developed a mechanistic mathematical model that explicitly accounts for parasite-parasite interactions within B. pfeifferi and is parameterized using values obtained from our experimental and field studies (supplementary data). In particular, our modeling framework tracks the outcomes for each parasite in the case of both simultaneous and sequential infections of B. pfeifferi with C. sukari and S. mansoni, based on survival rates from our empirical data. Furthermore, the model includes two snail size classes to account for size-dependent variation in snail survival and fecundity and cercariae output, which we have previously shown is important for accurately assessing the risk of infection to humans (Anderson et al., submitted). By varying the input of either S. mansoni or C. sukari miracidia to the model, we can examine how the relative abundance of S. mansoni to C. sukari larvae in the environment impacts the production of new parasites within the snail portion of the transmission cycle. The model predicts that the force of infection of S. mansoni, as measured by the proportion of cercariae-producing (shedding) snails, is reduced by approximately half in the presence of the antagonist C. sukari (Figure 5a). Only inputs of miracidia biased unrealistically heavily towards S. mansoni over C. sukari permit the highest numbers of S. mansoni cercariae to be produced (Figure 5b). We assume that the presence of C. sukari infections in B. pfeifferi represent ‘lost’ S. mansoni infections. Therefore, our survey results (Figure 2c) suggest that if C. sukari were absent then the prevalence of S. mansoni shedders in streams would increase by 50% or more. Also, the model predictions highlight that, dependent on the background levels of infection, there may be increases of several hundred percent of S. mansoni cercariae in the absence of C. sukari. Figure 5 with 3 supplements see all Download asset Open asset Mathematical model combining field and experimental observations. (A) At three different levels of S. mansoni prevalence in snails, the predicted reduction in prevalence of shedding snails from our transmission model due to the presence of C. sukari is estimated. In each case, the proportion of snails shedding S. mansoni is reduced by at least one half in the presence of C. sukari. (B) Relationship from model showing how S. mansoni cercariae production is maximized in this system only when the input of S. mansoni miracidia is very high relative to the input of C. sukari miracidia. Discussion Schistosoma mansoni is common in sub-Saharan Africa, aided by multiple transmission options, including humans, rodents and baboons as definitive hosts and by the widespread presence of several species of Biomphalaria vector snails occupying diverse aquatic habitats. Additionally, open human defecation and inadequate sanitation ensure widespread contamination of freshwater habitats with S. mansoni eggs (Nagi et al., 2014). We discovered at least 29 additional species of digenetic trematodes cycling through wild and domestic vertebrate definitive hosts that depend on Biomphalaria snails in western Kenya, creating inevitable opportunities for interactions if the larvae of two or more species co-occur in the same snail. In the rural settings where S. mansoni thrives, people often stand side-by-side with domestic ruminants to access water in streams or other water bodies, water that contains schistosome-infected B. pfeifferi. Domestic ruminants also predictably pass huge quantities of helminth eggs into the water, including those of C. sukari (we estimate ~10,000 eggs/cowpat). As C. sukari also develops in B. pfeifferi, a common and recurrent situation arises in which these two digenetic trematode species vie for access to their shared obligatory snail host. We have shown that S. mansoni occupies an intermediate position in a trematode dominance hierarchy in B. pfeifferi in west Kenya and can be routinely displaced if present in co-infections by echinostomes and amphistomes, although particular species may vary in their predatory tendencies and the degree of their dominance (Hechinger et al., 2011; Garcia-Vedrenne et al., 2016). Surprisingly, even though much of the previous work focusing on interspecific trematode antagonism has been undertaken in the context of exploiting it as a potential control strategy for schistosomiasis (Combes, 1982; Moravec et al., 1974; Pointier and Jourdane, 2000; Toledo and Fried, 2011) ours is the first study undertaken in which all the species involved are extant in sub-Saharan Africa, where schistosomiasis assumes its greatest public health significance. With respect to this hierarchy, the dominant trematodes are not the species we most commonly recovered from snails, for at least two reasons. One is that co-infections by no means inevitably occur within snails so that a species with a subordinate position is not always required to compete. This is of relevance for a species like S. mansoni occupying an intermediate hierarchical position in that it is not inevitably confronted in snails by predatory or inhibitory rediae of other species. Secondly, input of eggs from competitively dominant species like echinostomes often originates from birds (Laidemitt et al., 2019) and is likely dwarfed by the input of eggs from large definitive hosts like people, goats or cattle. This helps explain how the prevalence achieved by S. mansoni or by C. sukari is much higher than achieved by echinostomes despite the latter’s competitive dominance in snails. From the point of view of S. mansoni, colonizing a snail harboring a preexisting trematode infection, including even those snails infected with subordinate trematodes, is bound to interfere with its transmission to some extent. Successful infection will either be preempted by presence of a dominant species, or if take-over of a subordinate species occurs, the process likely takes weeks. In the interim, production of S. mansoni cercariae will be delayed or reduced, and the infected snail could well expire before the take-over is complete. Of the many trematodes coexisting with S. mansoni, C. sukari is most noteworthy for it is both abundant and dominant. Furthermore, the unusual facilitating effect provided to C. sukari by S. mansoni that we observed carries some provocative consequences. For example, from our experimental exposures of field-derived snails we found that B. pfeifferi were already colonized by C. sukari larvae, but the larvae were unable to complete development without a follow-up exposure to S. mansoni. This implies that most of the C. sukari infections we observed in streams represent snails that had been penetrated by S. mansoni miracidia and in which some degree of schistosome sporocyst development took place: the sporocysts were either stymied early in their development by the pre-existing presence of C. sukari larvae, or were able to develop to the point of cercariae production only to be taken over by a subsequent C. sukari infection. It is possible that other trematode species like strigeids or xiphidiocercariae may also facilitate C. sukari infections though they are much less abundant in our stream habitats than S. mansoni. By using our empirical work to parameterize a novel mathematical model that explicitly accounts for interaction between parasites within snail hosts, we were able to quantify the role of antagonistic trematodes in field snails on the force of schistosomiasis transmission in an endemic setting. Specifically, our mathematical model predicted that C. sukari could dampen the force of transmission of S. mansoni by as much as 65 percent. The presence of C. sukari-infected cattle thereby provides a significant protective effect for humans. Furthermore, the amphistome would not persist or would persist at much lower levels without the facilitation provided by S. mansoni. Consequently, in current mass drug administration (MDA) campaigns in Kenya, treating humans with praziquantel (with presumed downstream effects on limiting S. mansoni infections in snails) would then also decrease the number of successful C. sukari infections in snails and in turn the number of cattle infected with C. sukari. Conversely, if cattle were targeted in MDA campaigns to control Fasciola gigantica and other flukes like C. sukari, we would expect assuming no concomitant S. mansoni control, to observe an increase in the number of S. mansoni infections in snails. In particular, our model predicts at least a 40% increase (Figure 5a). The peculiar dependencies between C. sukari and S. mansoni provide an important example of how intramolluscan encounters could have significant downstream effects on the prevalence of infection of either parasite in their respective definitive hosts. The interactions we have noted between S. mansoni and C. sukari favor the latter species, but in other contexts, including in Kenya, the presence of another amphistome species Calicophoron microbothrium in the snail Bulinus tropicus facilitates infections with the cattle schistosome Schistosoma bovis (Southgate et al., 1989). Schistosomes and amphistomes, possibly because their respective positions in the dominance hierarchy lie close together (Figure 2), clearly engage in distinctive interactions that seem to favor the colonization of a snail species that might otherwise not easil
更多查看译文
关键词
human schistosomiasis,parasites,snail hosts,antagonism
AI 理解论文
溯源树
样例
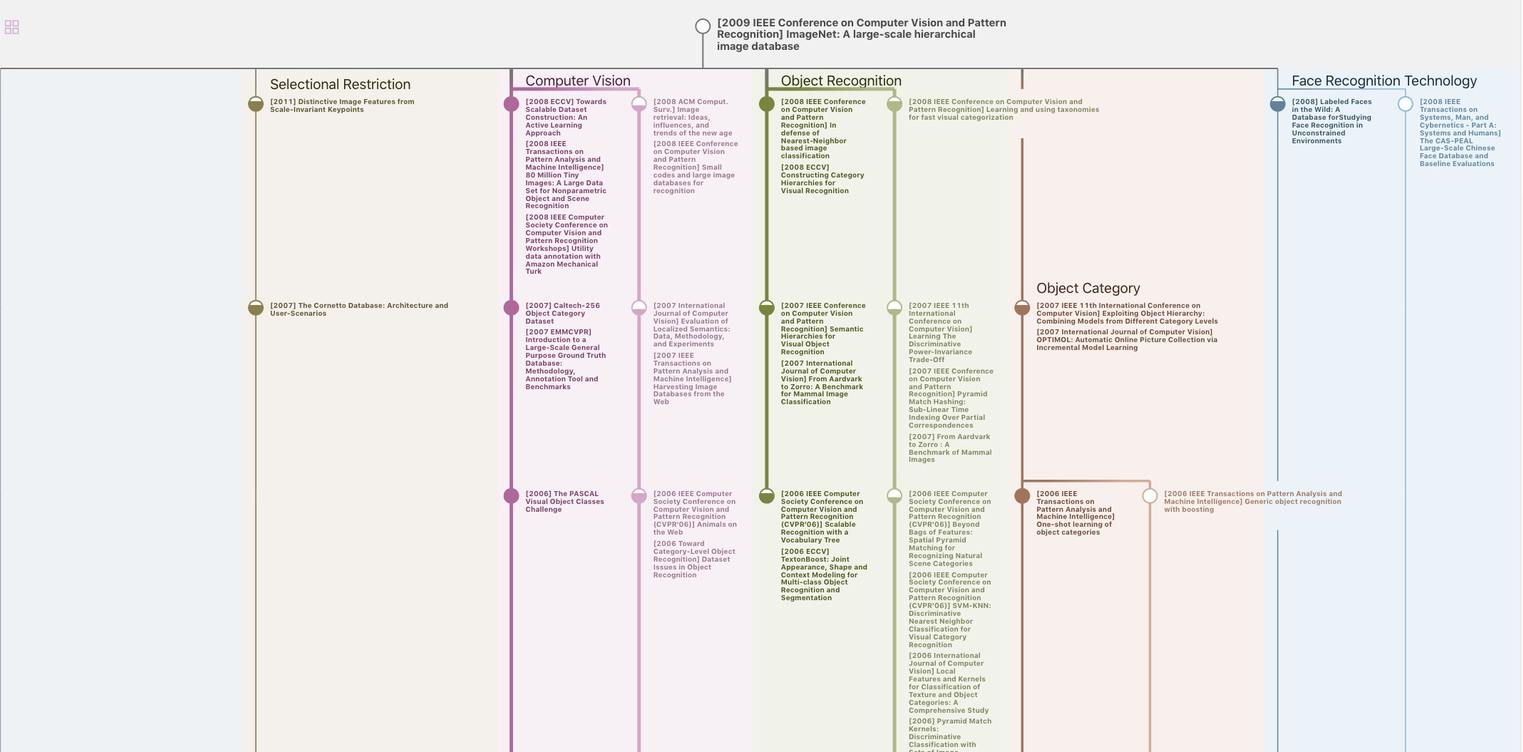
生成溯源树,研究论文发展脉络
Chat Paper
正在生成论文摘要