Breakthroughs in projection-enabled additive manufacturing: From novel strategies to cutting-edge applications.
Innovation (Cambridge (Mass.))(2023)
摘要
As one of the crucial technologies of additive manufacturing (AM), projection technology, which features fast printing speed, high molding accuracy, and low cost, exhibits great potential in cutting-edge applications. The basic principle of projection-enabled AM (PAM) is the stacking of layer-wise structures, in which the dynamic mask pattern is projected onto the surface of the liquid photosensitive resin, triggering polymerization in a specific area to accomplish single-layer printing. This facilitates the layer-wise manufacture of complex three-dimensional (3D) structures. Currently, the projection technologies of PAM can be mainly classified into liquid crystal displays, digital light processing (DLP), and silicon-based light crystal spatial light modulators. Among these, DLP is distinguished by its programmable two-dimensional (2D) projection ability. Initially, DLP was used as an imaging technique in which image signals were digitally processed and then projected. In recent years, DLP technology has been widely employed in optical AM. Figure 1 shows the development of PAM technologies, including typical processing schemes, processable materials, and concrete applications. Compared with conventional voxel-by-voxel AM strategies, DLP enables layer-wise 3D structuring, revealing distinct advantages including high efficiency, reasonable resolution, low cost, and great scalability. To date, DLP-enabled AM (DLP-AM) has opened a new avenue for advanced optical AM, attracting tremendous research interest. To achieve high processing speeds, tremendous breakthroughs have been made in DLP-AM technologies during the past few years. As a successful example, continuous liquid interface production (CLIP) technology has been developed to achieve fast and large-scale 3D printing through a dead zone formed by UV light and oxygen. However, further increase of the printing speed is challenging. When the printing parts rise too fast or the resin is too viscous, the resin does not fill in time, which determines the maximum printing speed. A new injection continuous liquid interface (iCLIP) method was proposed to overcome this limitation.4Lipkowitz G. Samuelsen T. Hsiao K. et al.Injection continuous liquid interface production of 3D objects.Sci. Adv. 2022; 8: eabq3917Crossref PubMed Scopus (7) Google Scholar The core component of this method is the microfluidic channel created and integrated into the growing parts. The resin can be injected into the printed area through the viaduct, overcoming the passive resin flow process of the original CLIP technology and thereby achieving actively controlled mass transfer (Figure 1). Therefore, the printing speed has been increased by 5–10 times, and resins that are an order of magnitude more viscous can be processed. In addition, the iCLIP method facilitates printing using various types of resins by injecting them individually through different channels. To further improve fabrication efficiency and resolution, Regehly et al. introduced a two-color linear volumetric AM (VAM) technology called Xolography. Using photoswitchable photoinitiators, this technology initiates local polymerization in a confined monomer volume by the linear excitation of intersectant light beams of different wavelengths. This yields a characteristic resolution of up to 25 μm and a curing rate of 55 cm3/s.3Regehly M. Garmshausen Y. Reuter M. et al.Xolography for linear volumetric 3D printing.Nature. 2020; 588: 620-624Crossref PubMed Scopus (143) Google Scholar In addition, Taylor and co-workers utilized the computed axial lithography (CAL) technique to develop a microscale CAL (m-CAL) VAM technology, which demonstrates microscale processing capability and possesses several distinct advantages such as high printing speed, layer-free manufacturing, and high-viscosity resin printing.1Toombs J.T. Luitz M. Cook C.C. et al.Volumetric additive manufacturing of silica glass with microscale computed axial lithography.Science. 2022; 376: 308-312Crossref PubMed Scopus (34) Google Scholar In addition to novel AM strategies, the development of processing materials is also critical. For example, glass demonstrates tremendous potential in the optical field owing to its excellent optical transparency, low thermal expansion coefficient, and chemical and thermal resistances. However, the direct fabrication of 3D glass devices via AM is challenging because the surface defects and limited geometric freedom cannot meet the strict surface requirements. Hence, the DLP-based m-CAL system has been employed to process photopolysilica nanocomposites through a tomographic irradiation method and obtain high-quality fused silica with excellent geometric freedom and minimum feature sizes of 50 μm.1Toombs J.T. Luitz M. Cook C.C. et al.Volumetric additive manufacturing of silica glass with microscale computed axial lithography.Science. 2022; 376: 308-312Crossref PubMed Scopus (34) Google Scholar The above-mentioned advantages of m-CAL-based VAM may motivate the integration of this technology with other AM techniques for high-efficiency and cost-effective 3D structuring. To further expand the material system, Saccone et al. reported the DLP-AM of microarchitected metals by hydrogel infusion. In this process, the poly(ethylene glycol) diacrylate-based hydrogel scaffoldings are first printed via DLP-AM, and then used as templates for controllable infusion with metal precursors.5Saccone M.A. Gallivan R.A. Narita K. et al.Additive manufacturing of micro-architected metals via hydrogel infusion.Nature. 2022; 612: 685-690Crossref PubMed Scopus (10) Google Scholar Subsequent calcination and reduction yields metal replicas that exhibit highly twinned microstructures and high hardness. The compositional flexibility of this method further promotes the manufacture of multiple metals and multicomponent alloys. Nevertheless, in most cases, multi-material AM enables the arbitrary spatial arrangement of two or more materials with different properties, which greatly promotes the design and manufacturing capabilities of 3D printing. However, considerable difficulties regarding residual resin removal may arise during material switching. To solve this problem, Ge and co-workers proposed a novel centrifugal multi-material (CM) 3D printing method, in which the centrifugal force generated via rotation can help remove the residual resin in a non-contact manner.2Cheng J.X. Wang R. Sun Z.C. et al.Centrifugal multimaterial 3D printing of multifunctional heterogeneous objects.Nat. Comm. 2022; 13: 7931Crossref PubMed Scopus (6) Google Scholar Consequently, CM 3D printing enables heterostructure prototyping, including hydrogels, polymers, shape memory materials, and ceramics. Recently, the development of adaptive and dynamic materials has attracted significant interest. The combination of DLP-AM and smart materials may enable 4D printing to achieve deformable 3D structures. DLP-AM technologies have opened up a multitude of cutting-edge applications (Figure 1). As a concrete example, DLP-AM holds great promise for developing micro-optics, as it enables the printing of complex 3D profiles based on various transparent materials, as demonstrated by the glass-based optical elements produced via m-CAL (Figure 1).1Toombs J.T. Luitz M. Cook C.C. et al.Volumetric additive manufacturing of silica glass with microscale computed axial lithography.Science. 2022; 376: 308-312Crossref PubMed Scopus (34) Google Scholar Moreover, the integratable processing capability in combination with high spatial resolution allows secondary printing on complex surfaces or devices. For instance, a microprobe can be printed and integrated onto the tip of an optical fiber to serve as an endoscope for disease detection. In addition, considering the 3D structuring capability at the microscale, DLP-AM may find broad applications in the fields of microfluidics and tissue engineering. For instance, DLP-AM can be used to produce human organ chips, biological cell tissues, and organ scaffolds. The application of DLP-AM technologies further depends on the coupled functional materials. Metal and alloy structures produced via DLP-AM may have broad applications in electronics and microelectromechanical systems. The emerging printable smart materials can endow the resultant 3D structures with unique properties, such as self-healing ability, high elasticity, stretchability, and deformability under external stimuli, which highlight their great significance for wearable devices, flexible electronics, and soft robots. In summary, the recent years have witnessed the rapid development of PAM technologies, represented by DLP-AM, which has facilitated many breakthroughs in processing strategies, processable materials, and cutting-edge applications. Nevertheless, DLP-AM is still in an early stage and several challenges must be overcome. For example, DLP-AM enables the printing of 3D structures with feature sizes ranging from tens of microns to a few centimeters. However, for more applications, the printing scale, spatial resolution, accuracy, and surface smoothness are still limited and require further improvement. This problem may be resolved by adopting multiple processing strategies to fabricate different parts of the 3D object via different AM strategies. In most cases, we must simultaneously balance accuracy, efficiency, and processing scale. With the advancement of projection technologies, new-generation PAM is expected to gradually emerge, which may promote the rapid progress of 3D printing technologies. From the viewpoint of materials, currently available photocurable materials for DLP-AM are limited. Therefore, the development of novel materials (such as smart materials) that permit PAM processing is highly desirable. In addition, the continuous processing of multiple materials in a 3D structure is also challenging. To achieve this, the processing systems need further optimization, and the binding force between different materials along with the mismatch of their mechanical, optical, and chemical properties should be considered. Currently, the PAM technology has entered a stage of rapid development. Let us wait and see the rise of this technology in the near future. This work was supported by the National Natural Science Foundation of China (nos. 62275044, 62205174, 61875036). The authors declare no competing interests.
更多查看译文
关键词
additive manufacturing,projection-enabled,cutting-edge
AI 理解论文
溯源树
样例
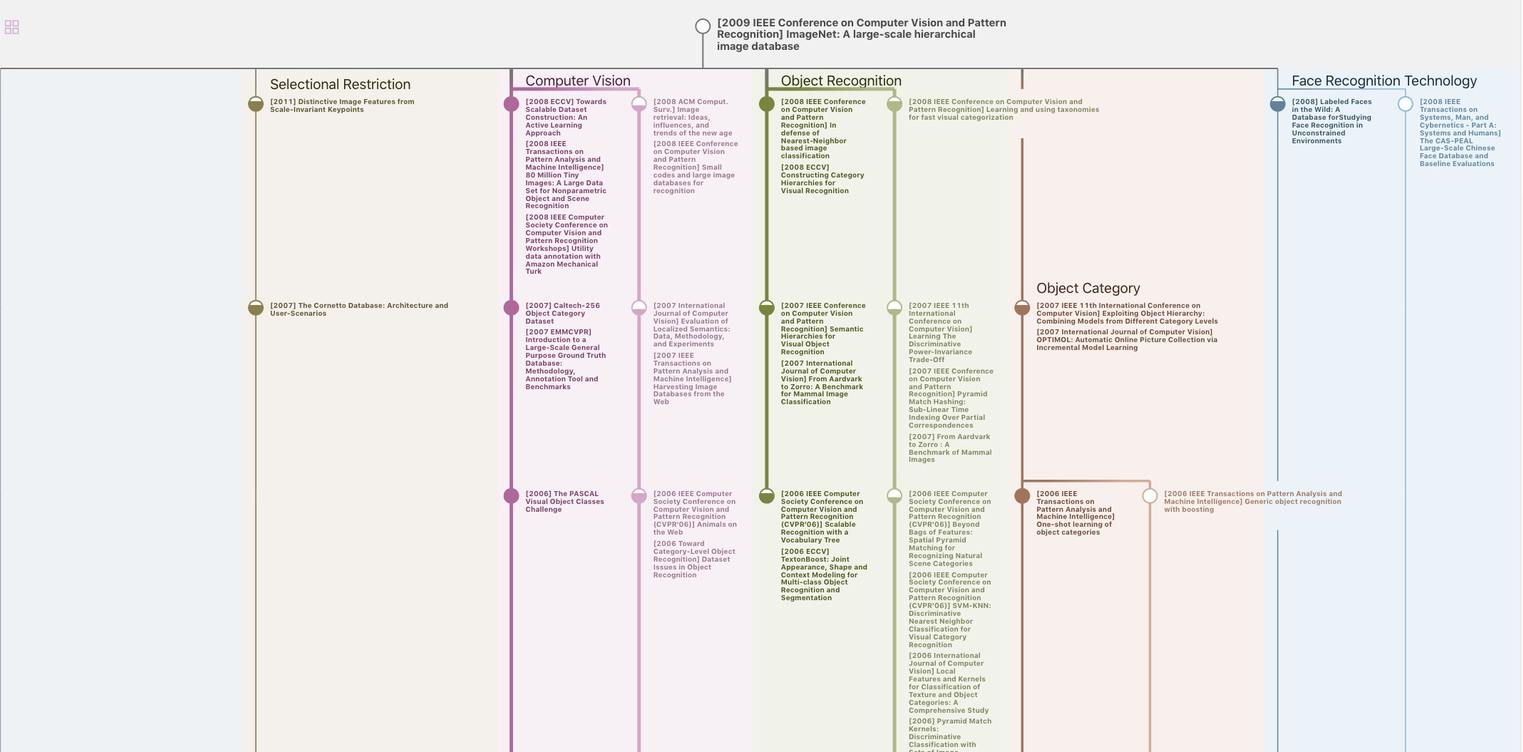
生成溯源树,研究论文发展脉络
Chat Paper
正在生成论文摘要