Error Quantification in Dynamic Applications of Weakly Nonlinear Transducers
semanticscholar(2021)
摘要
Characterization and quantification of dynamic measurements is an ongoing area of research in the metrological community, as new calibration methods are being developed to address dynamic measurement applications. In the development undertaken to date, one largely assumes that nominally linear transducers can be used with linear assumptions in deconvolution of the input from the response and in system identification. To quantify the errors that arise from these assumptions, in this article, the effects of weak nonlinearities in transducers that are assumed to behave linearly during dynamic excitations are studied. Specifically, a set of first-order and second-order systems, which can model many transducers with weak nonlinearities, are used to numerically quantify the systemic errors due to the linear assumptions underlying the deconvolution. We show through the presented results the evolution of different error metrics over a large parameter space of possible transducers. Additionally, an example of quantification of the errors due to linear assumptions in system identification is demonstrated by using a time-series sparse regression system identification strategy. It is shown that ∗Address all correspondence to this author. the errors generated from linear identification of a nonlinear transducer can counteract the systemic errors that arise in linear deconvolution when the linear system identification is performed in similar loading conditions. In general, the methodology and results presented here can be useful for understanding the effect of nonlinearity in single degree of freedom transient dynamics deconvolution and specifically in specifying certain metrics of errors in transducers with known weak nonlinearities. INTRODUCTION Dynamic calibrations and signal deconvolution for transducers are a major area of development in the metrology community over the past decade [1, 2]. The developed framework for evaluating measurement uncertainty relies on the principle of superposition [3]. However, this principle does not apply for finding a response of a nonlinear system. Hence, weak nonlinearities in transducers are a source of unaccounted error. Much work has been done to analyze the effects of weak nonlinear terms on the system response when the system is subjected to harmonic forcing. As an example, it is mentioned that Nayfeh and Mook [4] have shown how the frequency response of 1 Copyright © 2020 by ASME a system with weak cubic nonlinearity can be approximated near a primary resonance, a sub-harmonic resonance, and a superharmonic resonance by using perturbation methods. In general, these methods are meant for characterizing the responses of nonlinear systems subjected to a persistent excitation rather than a transient excitation such as an impulse. There are methods such as Volterra series based approaches, which have been used for determining the system response when subjected to an impulse input. Volterra series is a generalization of the convolution integral for nonlinear systems, and the Generalized Frequency Response Function (GFRF) may be considered as the equivalent one in the frequency domain [5]. A Volterra Series and GFRF consist of an infinite number of Volterra kernels; in practice they require truncation for actual application and can be computationally expensive for even low-order approximations [6, 7]. There has been previous work done on defining the generalized impulse response function for Gaussian impulses in applications related to the dynamic behavior of the economy [8]. In part due to the challenges encountered in identifying the effect of nonlinearity under arbitrary loading conditions, many transducers are designed to operate as linearly as possible, and in some cases, these transducers are subjected to nonlinear dynamics compensation strategies [9, 10]. Despite the efforts undertaken to date, transducers generally have some degree of nonlinearity in the responses which can lead to measurement errors. In this context, it is mentioned that we have found no previous work on quantification of the errors that arise in deconvolution when one assumes the responses of weakly nonlinear systems subjected to impulse loading conditions to be linear. Here, we use numerical methods to analyze a parameter space that we posit to be applicable to real transducers, and may be useful in quantifying the errors that arise due to the neglect of nonlinearities in nominally linear transducers. In order to isolate the uncertainty due to the nonlinearity, other sources of potential measurement error are neglected. We assume noiseless measurements and that the dynamics of the system is exactly described by a first-order or second-order system, as shown in equation (1) and (2), respectively [11]. We focus primarily on transducers that are modeled as first-order systems, which can also be a suitable model for filtered certain secondorder and higher systems. Some representative second-order system results are also included.
更多查看译文
AI 理解论文
溯源树
样例
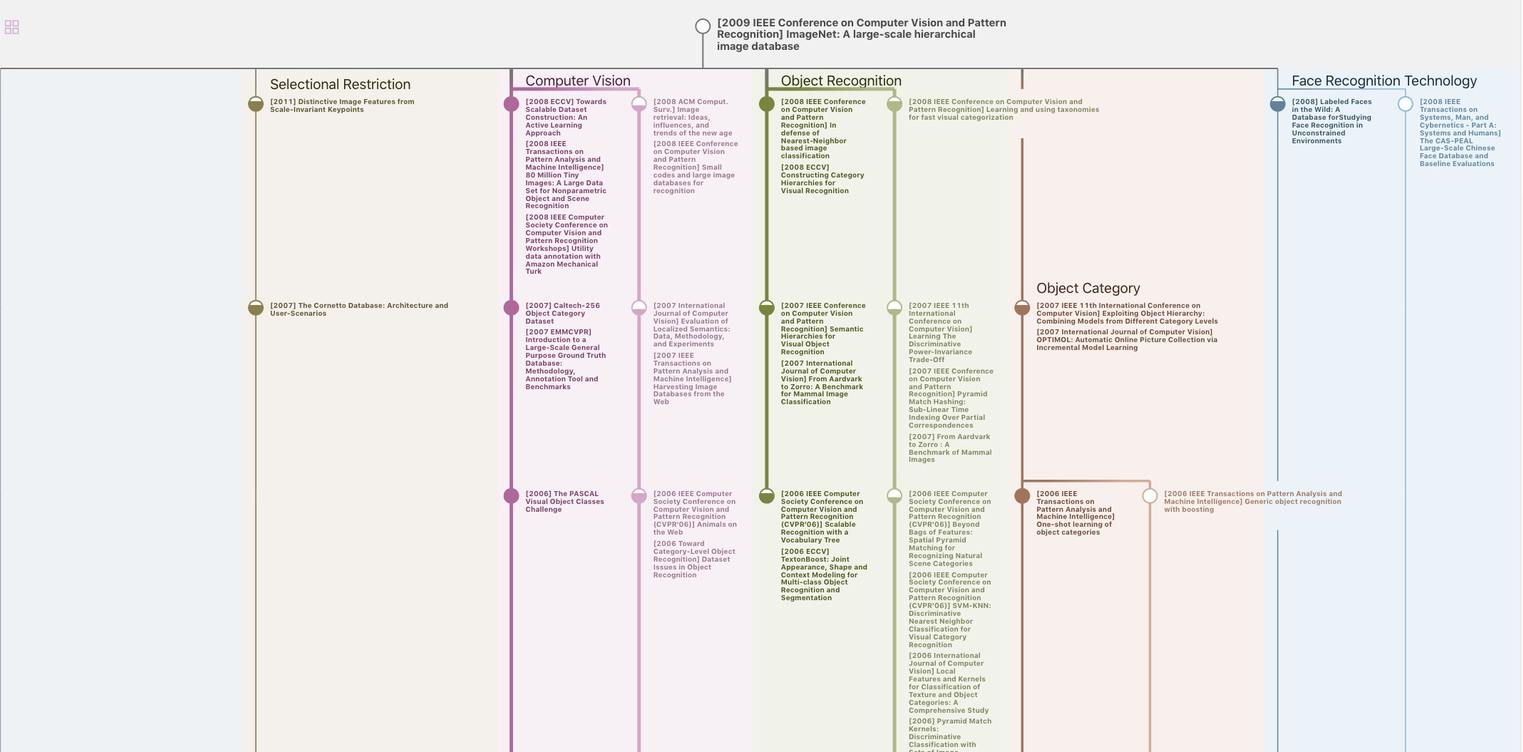
生成溯源树,研究论文发展脉络
Chat Paper
正在生成论文摘要