Optimising Dual Layer Recording Using Antiferromagnetic Exchange Coupling
2018 IEEE INTERNATIONAL MAGNETIC CONFERENCE (INTERMAG)(2018)
摘要
Multiple layer magnetic recording may be possible with the advent of microwave-assisted magnetic recording (MAMR) [1], [2]. In a MAMR system, recording on a medium with high uniaxial anisotropy is made possible by applying a high frequency (HF) magnetic field at the resonance frequency of the medium, leading to a reduction in the switching field. If two discrete recording layers, RL1 and RL2, have different resonance frequencies, selective recording on one or other of the layers may be possible by adjusting the frequency of the spin torque oscillator (STO) used to generate the HF field. The magnitude of the coercivity reduction is related to the strength of the HF field in the medium. As STOs typically have dimensions from 20 – 40 nm the HF field strength decreases rapidly with distance from the STO. For this reason, if multiple recording layers are to be used each layer must be as thin as possible and the separation between the layers cannot be large. However, if the separation between the recording layers is small, magnetostatic interactions between them are large. This can make it difficult to implement selective recording due to the magnetostatic interaction favouring parallel alignment of the magnetisation of both recording layers. It may be possible to counteract the influence of the magnetostatic field by introducing antiferromagnetic exchange coupling between the two recording layers, e.g., by using Ru or Ir spacers [3], and this is the topic of this work. The aim is to optimise the antiferromagnetic exchange coupling and to determine the effect on recording performance. The Model Dual layer recording media with a 7 nm RL1 / 2 nm Spacer / 5 nm RL2 / 3.5 nm Air / Write Head structure were modelled. Each recording layer was an exchange coupled composite (ECC) structure with $M_{s} =750$ emu/cm
3
in all layers and the hard layers below the soft layers. In the model the medium grains were discretised into 1 nm-thick layers with the exchange coupling constant between layers of the same material being 10 erg/cm
2
. After optimisation of the layer thicknesses and other parameters RL1 had a 3 nm hard layer and a 4 nm soft layer. The soft layer had $K_{u}$ of 3 × 10
6
erg/ cm
3
and the exchange coupling between the hard and soft layers was 4 erg/ cm
2
. RL2 was formed of a 2 nm hard layer and a 3 nm soft layer. The soft layer had $K_{u}$ of 5 × 10
6
erg/cm
3
and the exchange coupling between the hard and soft layers was 6 erg/cm
2
. The hard layer $K_{u}$ of both recording layers was a variable parameter. The field distribution from a write head with a 36 nm wide main pole and a 30 nm gap between the main pole and the trailing shield was calculated using a finite element model. A STO was placed 5 nm behind the main pole to generate the HF field in the media. The STO was modelled as a 36 nm wide, 36 nm high and 14 nm thick field generating layer (FGL) with $M_{s}$ of 1591 emu/cm
3
and coherent rotation of the FGL magnetisation was assumed. Results The effect of the magnetostatic interaction on the switching of the magnetisation of RL1 is shown in Fig. 1. In the calculation a 4 ns field pulse was applied to an 8 nm $\times 8$ nm grain containing two discrete recording layers, RL1 and RL2. The hard layer $H_{k} ( = 2 K_{u}/ M_{s})$ was varied and the maximum $H_{k}$ that could be switched by the combination of the head field and STO field was calculated as a function of HF field frequency. The magnetisation of RL2 was fixed either “up” or “down” and, as Fig. 1 shows, the result was to change the maximum switchable $H_{k}$ of RL1 by about 17 kOe: this difference was due to the magnetostatic field from RL2 acting on RL1 and is measured by the quantity $\Delta H_{k}$. Next, antiferromagnetic exchange coupling with a strength $J_{IL}$ was introduced between RL1 and RL2. The inset to Fig. 1 shows the effect of $J_{IL}$ on $\Delta H_{k}$. When $J_{IL}$ was about -0.17 erg/cm
2
the influence of the magnetostatic field was eliminated and $\Delta H_{k}$ was zero. Tracks were written on media with two recording layers using HF field frequencies of 20 GHz and 50 GHz. The bit length was 30 nm (847 kfci) and the head velocity was 5 m/s. The temperature was 300 K and distributions of grain size (16.5%), $M_{s}$ (4.25%), $K_{u}$ (8.5%) and easy axes $(3^{\circ })$ were included. The signal to noise ratio (SNR) was calculated for tracks recorded on each layer using the sensitivity function of a 20 nm wide magnetoresistive (MR) read head. Fig. 2 shows the results as a function of $J_{IL}$. For RL1 the SNR increased by about 1.2 dB when $J_{IL}$ was optimised, compared with the case when $J_{IL}$ was zero. A small amount of ferromagnetic coupling between RL1 and RL2, e.g., $J_{IL} =0.1$ erg/cm
2
, caused a sharp decrease in the SNR of RL1. The SNR of RL2 was higher due to the layer being nearer to the write head and consequently experiencing higher head field gradients and HF fields. The SNR of RL2 was almost unchanged by $J_{IL}$ until $J_{IL}$ reached -0.3 erg/cm
2
. Reversing the order of the hard and soft layers in RL1 to put the hard layer next to the non-magnetic interlayer made RL1 more resistant to the magnetostatic fields from RL2, but reduced the SNR. This was because magnetisation reversal was initiated in the soft layer and this layer experienced a smaller HF field when it was further from the STO. The authors would like to thank the ASRC for their support of this work.
更多查看译文
关键词
dual layer recording,microwave-assisted magnetic recording,high uniaxial anisotropy,high frequency magnetic field,resonance frequency,switching field,spin torque oscillator,coercivity reduction,HF field strength,magnetostatic interactions,magnetisation,ruthenium spacers,iridium spacers,model dual layer recording media,write head structure,exchange coupled composite structure,optimisation,layer thickness,trailing shield,finite element model,HF field frequency,head velocity,grain size distribution,signal-to-noise ratio,sensitivity function,magnetoresistive read head,head field gradients,non-magnetic interlayer,soft layers,hard layers,magnetostatic field,recording layer,multiple recording layers,RL2,discrete recording layers,multiple layer magnetic recording,antiferromagnetic exchange coupling,size 7.0 nm,size 2.0 nm,size 5.0 nm,size 3.5 nm,size 14.0 nm,size 750.0 cm,frequency 50.0 GHz,energy 10.0 erg,size 3.0 nm,size 4.0 nm,energy 4.0 erg,energy 6.0 erg,size 36.0 nm,size 30.0 nm,size 1591.0 cm,time 4.0 ns,size 8.0 nm,size 8.0 nm,velocity 5.0 m/s,temperature 300.0 K,size 20.0 nm,noise figure 1.2 dB,energy 0.1 erg,energy -0.3 erg,size 20.0 nm to 40.0 nm,frequency 20 GHz,Ru,Ir
AI 理解论文
溯源树
样例
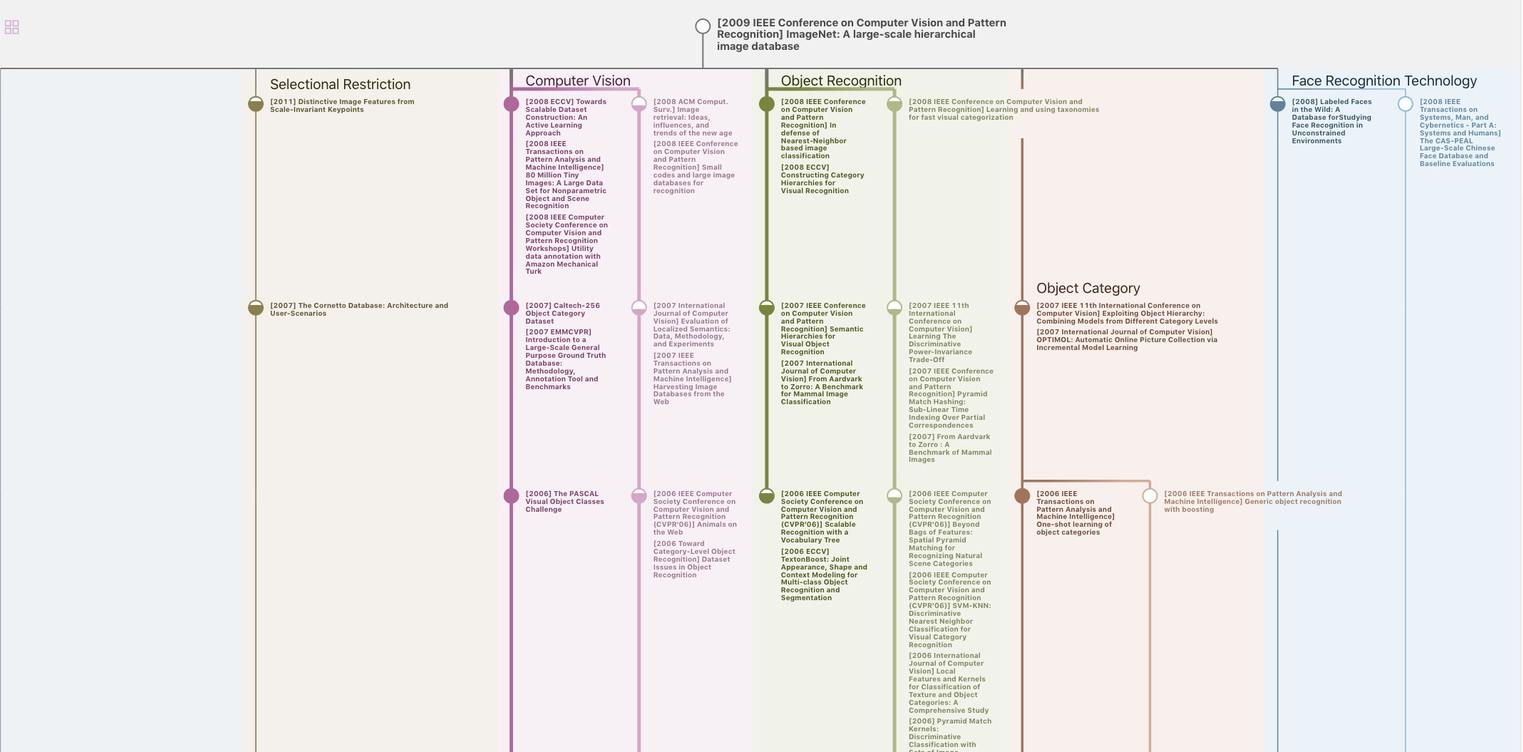
生成溯源树,研究论文发展脉络
Chat Paper
正在生成论文摘要