The impact of imaging speed of MR-guided punctures and interventions in static organs—A phantom study
European Journal of Radiology(2011)
摘要
Materials and methods We measured the duration, accuracy and error rate of simulated interventions for different acquisition-times using a simplified interventional setup. All measurements were performed in a 1.0 T open MRI scanner. Imaging was performed with a gradient-echo sequence (flipangle = 20°; TR/TE = 12/6 ms; voxelsize = 1 mm × 1 mm; slicethickness = 5 mm; FOV = 230 mm × 200 mm; acquisition-time = 1 s). Variable acquisition times were simulated with intermediate pauses of 0, 1, 2, 3, 4 and 5 s. The interventions were performed by a total of 20 volunteers including 7 experienced interventionalists. Results The mean duration of the intervention was 2 min. Significant differences between experienced and unexperienced volunteers were limited to the localization of the image plane and corrections made. The mean accuracy was 5.6 mm. The time to localize the image plane increased with deceleration of imaging from 24 s to 49 s. A similar increase was observed for the intervention time (55–108 s). A significant influence of the acquisition-time on durations and corrections was only found with acquisition-times greater than 4 s per image. Conclusion Even image rates of several seconds per image are sufficient enough for efficient interventions in static organs. Thus, the main attention has to be turned on the visibility of the needle when sequences are optimized for MR-guidance. The minimization of imaging speed is rather of secondary interest. Keywords MR guidance Open MRI Interventional MRI Real-time imaging 1 Introduction Image-guided interventions have gained in importance over the past years. This is owed to the increasing number of minimally invasive procedures in modern medicine, which require new monitoring techniques [1–5] . The advantages of real-time fluoroscopic guidance of biopsies, punctures or tumor ablations with X-ray, computer tomography (CT) and ultrasound (US) are well described [1,2,6–11] . With the introduction of open magnetic resonance imaging (MRI) scanners and improved patient access, MR-guided interventions have become increasingly popular [12–15] . Compared to CT, MRI especially simplifies a cranio-caudal intervention approach [12,16] . Hence, MR-fluoroscopy guidance can represent a significant improvement for cross-sectional interventions. If no additional navigation devices are used, the interventional procedure normally includes the adjustment of the image plane and the intervention itself. The image plane is fixed so that the access point of the medical device and the target of the intervention are in line with the field of view (FOV). To perform the intervention, the interventionalist has to localize the image plane and access point. The most basic way of accomplishing this is the free hand technique – also known from CT-guided interventions: the interventionalist moves the tip of his finger along the patient's skin until both finger and target are visualized on one image. To date, no specific analysis has been performed on how fast imaging should be to maximize the safety and efficiency of image guided interventions and what a minimum frame-rate should be. On this account, our study was designed to systematically measure the influence of the acquisition speed of MR-guidance on the duration and error rate of interventions solely. In an in vivo intervention, various other factors like changing tissue contrast, mechanical tissue properties, different tissue motions, patient access, the angle and distance of the puncture conduits are influencing the efficiency of the procedure. Therefore, a simplified static interventional setup in a phantom study was chosen to exclude these factors and to get a reference to the degree of impact of the imaging speed on the success of an in vivo intervention. 2 Materials and methods The study included two procedures: (a) locating the current MR-image plane using the free hand technique and (b) the actual intervention starting with the penetration of the puncture phantom's surface and ending after a target was hit. The intervention was completed when the interventionalist was sure to have reached the target point. A total of 20 volunteers performed the interventions for each of six different acquisition times resulting in different imaging rates. Seven of the volunteers were experienced in image guided interventions (either CT or MRI). 2.1 Puncture phantom The phantom was made of two compartments of gel filled in a 20 cm × 15 cm × 15 cm container of synthetic material ( Fig. 1 a). The lower compartment of the phantom consisted of a 3 cm layer of Wirogel (Bego, Bremen, Germany) and the upper compartment of 1.5% agarose gel. As a target we used a plastic hollow cone with a 3 cm diameter and 2.5 cm length. The aperture of the hollow cone was placed at the center of the horizontal plane, 3 cm from the bottom within the Wirogel and 6 cm from the surface of the agarose gel. Thus, the agarose gel could be easily renewed without changing the position of the target. To ensure a constant angle and distance of the puncture conduit, possible insertion points were restricted by a circular aperture 12.5 cm in diameter at the center of the container's cover. Thus, in-plane angulations relative to the main magnetic field resulted in maximum angulations of 28.5° with a distance of 13 cm from the target, i.e. the hollow cone. Since the phantom did not provide any anatomical land marks, visual reference points were marked around the cover aperture, similar to those on the face of a watch, to facilitate orientation for the interventionalist. These marks also simulated restrictions, which are normally provided by anatomical structures in an in vivo intervention. Controller orientation at the MR-console was achieved with corresponding MR markers attached to the bottom of the box's cover ( Fig. 1 b). The MR markers were two opposed cylinders ( Ø = 3 cm, 0.5 cm length) filled with water and ten equally spaced vitamin E capsules in between. 2.2 Experimental set-up All measurements were performed in a 1.0 T open Panorama HFO MRI scanner (Philips Healthcare, Best, The Netherlands) using an abdominal coil with a 40 cm aperture (vertical diameter, Fig. 2 ). An MR-compatible in room monitor (Philips Healthcare, Best, The Netherlands) was placed in front of the scanner's aperture to display the MR-images in the interventionalist's line of sight. 2.3 Sequence A T1 weighted gradient echo sequence (flip angle = 20°; TR/TE = 12/6 ms; voxel size = 1 mm × 1 mm; slice thickness = 5 mm; FOV = 230 mm × 200 mm; acquisition time ta = 1 s) was used as an interventional sequence. The phase encoding direction was vertical (along columns) for all interventions. To analyze the dependence of safety and efficiency of the intervention versus the imaging speed variable acquisition times were simulated by time intervals of 0, 1, 2, 3, 4 and 5 s between each image acquisition. This resulted in acquisition times of ta = 1, 2, 3, 4, 5 and 6 s, while minimizing changes of the contrast-to-noise ratio (CNR) and SNR. 2.4 Puncture procedure We used a 15 cm 16G titanium-based needle (Somatex, Teltow, Germany) in all interventions. The image plane was aligned with the main magnetic field at the center of the hollow cone (target) by the controller at the MR-console, while varying the degree of rotation around the symmetry axis of the hollow cone for each intervention. By varying the rotation angel, a potential influence of puncture channels from preceding interventions on the current intervention was precluded. In addition, the upper gel compartment was renewed after every 12 puncture procedures. Image plane orientation was not modified throughout the puncture process. Image plane localization began with a starting point of the fingertip at the boundary of the aperture 10 cm from the predefined image plane. To attain reproducible distances, the interventionalist was instructed by the MR controller on what “time” marker to begin with as starting point for every intervention. Thereby, the correspondence of MR-markers and visual reference points on the cover enabled an alignment of the coordinate systems of the MR-controller and the interventionalist. The interventionalist was further instructed to search for the predefined image plane in a clock-wise along the circumference of the cover aperture. The total distance of this search was constantly 6.5 cm in all experiments. The intended final position of the needle tip after the puncture procedure was defined to be at the center of the hollow cone at the level of its’ upper rim. All interventions were repeated three times, resulting in a total of 360 punctures. 2.5 Measuring parameters SNR and CNR values were measured by the ratios of the mean MR-signal in gel and the needle and the standard deviation in air to confirm consistent values. Parameters to define the efficiency of the intervention were: duration of plane localization: tl; duration of the actual intervention: tp and any corrections made: cn. The number of corrections made cn was augmented each time the needle was retracted and readjusted. The number of corrections is especially important for the judgment of the invasiveness of the procedure. To validate the success of the intervention, the spatial accuracy of the puncture was defined by the distance of the needle tip from the middle of the hollow cone in a horizontal image plane. Statistical evaluation was performed with respect to the specified parameters. Particular significances were calculated by analysis of variance with repetitions. 3 Results No significant increase of SNR or CNR was found with increasing acquisition times. The SNR (9.5) and CNR (6) values remained constant with acquisition times beyond 3 s. The artifact width was 7 ± 1 mm. Due to the soft structure of the agarose, the gel did not have an influence on the integrity of the needle. No bending or needle abrasions were observed. Every intervention was performed successfully within 5 min. As expected, there were significant dependencies of the acquisition time ta and the parameters tl and tp. At the shortest acquisition time ta = 1 s the mean duration to localize the image plane was 24 (±12) s. With acquisition times of 6 s, we found an increase of tl up to 49 (±18) s ( Fig. 3 a) corresponding to 8 (±3) dynamic images. The selective statistical analysis of the measurement parameters yielded high significances of tl comparing each value with the value at ta = 1 s ( Table 1 ). For experienced volunteers significant dependencies for acquisition times beyond 4 s were observed, whereas the dependencies were weaker than for inexperienced volunteers. A similar increase was observed for the puncture time tp, which increased from 55 (±31) s to 108 (±18) s ( Fig. 3 b), but no significant differences between the two groups were found. Hence, the number of images needed per puncture decreased from 55 (±31) to 18 (±3). Significant dependencies were observed starting at ta = 4 in both groups for puncture time. We found no significant difference between both groups regarding the puncture time tp, although the mean duration of the inexperienced volunteers was higher (inexperienced: tp = 82 s; experienced: tp = 67 s). The mean duration of the entire procedure over all volunteers and acquisition times was 2 min (±80 s). A significant influence of the acquisition time on the performed corrections was only found for inexperienced volunteers with an acquisition time of 4 s. The analysis of accuracy yielded no significant changes (data not shown). Only a minor increase in the number of corrections was seen: cn = 2.5 (±1.8) at ta = 1 s and cn = 4 (±3.3) at ta = 6 s ( Fig. 3 c). The mean accuracy was dist = 5.6 ± 3 mm. Neither a significant difference between inexperienced (dist = 5.9 ± 2.8 mm) and experienced volunteers (dist = 5.1 ± 2.5 mm), nor a significant influence of the acquisition time on the accuracy was found ( Table 2 ). Thus, significant differences between the two groups were limited to the localization times tl ( p = 0.01) and corrections made cn ( p = 0.01). All assessment parameters had wide distributions in both groups. 4 Discussion Today, MRI is also used in the guidance of complex interventions, such as (tumor) ablations and pain therapy [12,17–19] . However, MR imaging is still a comparatively slow technique. Techniques to improve MR image-rates, like echo planar imaging, decreased k -space acquisition or non-cartesian acquisition of the k -space is often related to a loss of signal-to-noise ratio (SNR) [20–26] . Therefore, interventional MR-sequences are a compromise between reduced spatial and maximized temporal resolution. Sequences used in interventions vary in image acquisition-rates from 0.5 s up to 5 s per image. Therefore, monitoring interventions is only possible “near” real-time [27,28] . Slower sequences with acquisition times of less than 2 s have been used to monitor LASER and radio frequency induced thermal therapy of the liver [29,30] . Acquisition times of 3.5 s were sufficient for MR-guided needle placement during cryo-ablation of liver lesions [31] . Furthermore, acquisition times of 6 s per image have been used to monitor the LASER-ablation of brain tumors in “real-time” [32] . It seems ambitious to maintain using the term “real-time” or “fluoroscopy” in case of MRI where acquisition times are often greater than one second per image. Alternatively, the meaning of real-time is if a system is “predictably fast enough for use by processes being serviced” [33] . Hence, depending on the respective time scale of the process, the desired response time may vary. The requirements for the minimum frame rate of MR-guided interventions are usually not clearly defined. An important restriction is the motion speed of the subject of study. Due to the diversity of degrees of organ motion in vivo, the required frame rates for interventions in moving organs can differ as well. The frame rate restrictions for interventions in static organs are even less obvious and not determined yet. Acquisition times faster than 1 second per image may be amenable for some procedures, even in static organs (i.e. lesions near large vessels, monitoring of fluid collections or cyst drainage). Thus, our aim was to analyze solely the effect of acquisition time on the efficiency of MR-guided punctures on static targets. On this account, some simplifications and restrictions had to be made in our experimental setup to ensure that the acquisition time was the only crucial variable in the study. The consistency of SNR and CNR with rising acquisition times was especially important for comparable results. Therefore, the choice of the imaging parameters and type (gradientecho, spinecho) was addressed to rule out side effects while providing a short initial acquisition time per image. Again, the needle artifact and thus the visibility of the needle are strongly influenced by the orientation of the needle with respect to the main magnetic field and the field strength of the scanner. In cylindrical scanners with horizontal field orientation, both CNR and SNR values, as well as the angulations of the needle would differ. Hence, a generalization of our results and transfer to higher field strengths and cylindrical systems is limited. In contrast to the applied image sequence, spin echo sequences are more prone to saturation effects and were therefore excluded. An interesting imaging sequence for MR-guided interventions is the balanced steady state free procession sequence (bSSFP). A characteristic of this sequence is the dependency of SNR on inhomogeneities of the magnetic field resulting in stripes of lower SNR (banding). Hence, different positions and shapes of banding in different interventions hampered with a reproducible visualization of the needle in this study. The rotational symmetry of the homogeneous phantom did not offer any anatomic landmarks for the interventionalist. Hence, the accurate puncture was more demanding with respect to the spatial imagination and orientation of the volunteers. Thus, the chosen experimental setup was different to in vivo interventions. Though, the rapid performance of all volunteers showed that every volunteer was able to manage the increased difficulty. The measurement of the horizontal distance between the needle tip and the aimed at end position showed high precision for all volunteers and acquisition times. The vertical variation was negligible since it was mostly determined by the study design. Therefore, the vertical accuracy was owed to the in-plane resolution and the size of the needle artifact (tip shift error). The horizontal tolerance was within the limits of slice thickness and needle artifact diameter. The accuracy of a puncture in an in vivo intervention is highly dependent on the visibility of the needle and its contrast to the surrounding tissue. These factors are only indirectly affected by the acquisition speed but, i.e. directly by the spatial resolution, and were not considered in this study. Nevertheless, a lower CNR could increase the sensitivity of the efficiency of an intervention on the imaging speed reducing targeting accuracy and optimal speed. The acquisition time did not have a significant influence on the number of corrections for other acquisition times than ta = 4 s. Thus, the dependency of the frame rate on the need for corrections will be minor. The operating experience had a more important impact on the time to locate the current image plane with the free hand technique and the corrections made. Interventional practice seemed to improve spatial orientation and memory. Thus, especially the preparation of the puncture process was accelerated with more experience. The significant changes regarding the influence of the frame rate on the assessment parameters of experienced interventionalists suggest that the efficiency of a puncture decreases only moderately with acquisition times of up to 4 s. With higher acquisition times, the accuracy did not deteriorate and the number of corrections remained almost constant. The MR-image was mainly used as cross-check during the needle insertion. Thereby, the visibility of the needle was of more importance than the imaging speed. In contrast, the frame rate was more relevant for inexperienced volunteers who had specific difficulties locating the image plane with lower frame rates. In conclusion: Although the imaging speed is an important factor for image guided interventions, even image rates of several seconds per image are sufficient enough for efficient interventions in static organs. Longer acquisition times still allow safe interventions regarding the accuracy and the probability of errors but will prolong the total duration of the intervention. The findings of our study suggest, that the main attention has to be turned on the visibility of the needle when sequences are optimized for MR-guidance. The minimization of imaging speed is rather of secondary interest. References [1] J.J.V. Gompel D.F. Kallmes J.M. Morris N.C. Fode-Thomas J.L.D. Atkinson Dyna-CT as an imaging adjunct to routine percutaneous balloon compression for trigeminal neuralgia Stereotact Funct Neurosurg 87 2009 330 333 [2] D.E. Heron R.P. Smith R.S. Andrade Advances in image-guided radiation therapy – the role of PET-CT Med Dosim 31 2006 3 11 [3] F.T. Mangano D.D. Limbrick J.R. Leonard T.S. Park M.D. Smyth Simultaneous image-guided and endoscopic navigation without rigid cranial fixation: application in infants: technical case report Neuro-surgery 58 2006 ONS–E377 [4] F.G. Rocha E. Benoit M.J. Zinner Impact of radiologic intervention on mortality in necrotizing pancreatitis: the role of organ failure Arch Surg 144 2009 261 265 [5] V.A. Sahni S.G. Silverman Biopsy of renal masses: when and why Cancer Imaging 9 2009 44 55 [6] S. Gupta M. Sultenfuss J.E. Romaguera CT-guided percutaneous lung biopsies in patients with haematologic malignancies and undiagnosed pulmonary lesions Hematol Oncol 2009 PMID:19728397 [7] J. Hänsler D. Neureiter M. Wasserburger Percutaneous US-guided radiofrequency ablation with perfused needle applicators: improved survival with the VX2 tumor model in rabbits Radiology 230 2004 169 174 [8] H. Hoppe S.L. Wang B.D. Petersen Intravascular US-guided direct intrahepatic portocaval shunt with an expanded polytetra-fluoroethylene-covered stent-graft Radiology 246 2008 306 314 [9] F. Laspas E. Sotiropoulou S. Mylona Computed tomography-guided radiofrequency ablation of hepatocellular carcinoma: treatment e-cacy and complications J Gastrointestin Liver Dis 18 2009 323 328 [10] B.D. Lewis J.W. Charboneau C.C. Reading Ultrasound-guided biopsy and ablation in the neck Ultrasound Q 18 2002 3 12 [11] Z. Morvay E. Szabó L. Tiszlavicz J. Furák I. Troján A. Palkó Thoracic core needle biopsy using ultrasound guidance Ultrasound Q 17 2001 113 121 [12] J.A. Carrino B. Khurana J.E. Ready S.G. Silverman C.S. Winalski Magnetic resonance imaging-guided percutaneous biopsy of musculoskeletal lesions J Bone Joint Surg Am 89 2007 2179 2187 [13] K.A. Smith J. Carrino MRI-guided interventions of the musculoskeletal system J Magn Reson Imaging 27 2008 339 346 [14] S. Clasen A. Boss D. Schmidt Magnetic resonance imaging for hepatic radiofrequency ablation Eur J Radiol 59 2006 140 148 [15] T.J. Vogl H.P. Mayer S. Zangos J.B. Selby H. Ackermann F.B. Mayer Prostate cancer: MR imaging-guided galvanotherapy – technical development and first clinical results Radiology 245 2007 895 902 [16] J. Fritz C. Thomas S. Clasen C.D. Claussen J.S. Lewin P.L. Pereira Freehand real-time MRI-guided lumbar spinal injection procedures at 1.5 T: feasibility, accuracy, and safety AJR Am J Roentgenol 192 2009 W161 W167 [17] P.R. Eby C.D. Lehman Magnetic resonance imaging-guided breast interventions Top Magn Reson Imaging 19 2008 151 162 [18] U. Fischer L. Schwethelm F.T. Baum S. Luftner-Nagel J. Teubner Effort, accuracy and histology of MR-guided vacuum biopsy of suspicious breast lesions-retrospective evaluation after 389 interventions Rofo 181 2009 774 781 [19] F. Streitparth T. Walter U. Wonneberger Image-guided spinal injection procedures in open high-field MRI with vertical field orientation: feasibility and technical features Eur Radiol 2009 395 403 [20] R.R. Edelman P. Wielopolski F. Schmitt Echo-planar MR imaging Radiology 192 1994 600 612 [21] J.E. Bishop G.E. Santyr F. Kelcz D.B. Plewes Limitations of the keyhole technique for quantitative dynamic contrast-enhanced breast MRI J Magn Reson Imaging 7 1997 716 723 [22] M. Busch A. Bornstedt M. Wendt J.L. Duerk J.S. Lewin D. Grönemeyer Fast “real time” imaging with different k -space update strategies for interventional procedures J Magn Reson Imaging 8 1998 944 954 [23] Z. Chen J. Zhang K. Pang Adaptive k -space updating methods for dynamic MRI sequence estimation Conf Proc IEEE Eng Med Biol Soc, vol. 7 2005 7401 7404 [24] J.L. Duerk J.S. Lewin D.H. Wu Application of keyhole imaging to interventional MRI: a simulation study to predict sequence requirements J Magn Reson Imaging 6 1996 918 924 [25] W.H. Perman E.V. Heiberg V.M. Herrmann Half-Fourier, three-dimensional technique for dynamic contrast-enhanced MR imaging of both breasts and axillae: initial characterization of breast lesions Radiology 200 1996 263 269 [26] A. Shankaranarayanan M. Wendt A.J. Aschoff J.S. Lewin J.L. Duerk Radial keyhole sequences for low field projection reconstruction interventional MRI J Magn Reson Imaging 13 2001 142 151 [27] S. Terraz A. Cernicanu M. Lepetit-Coiffé Radiofrequency ablation of small liver malignancies under magnetic resonance guidance: progress in targeting and preliminary observations with temperature monitoring Eur Radiol 2010 886 897 [28] J. Fritz P.L. Pereira MR-guided pain therapy: principles and clinical applications Rofo 179 2007 914 924 [29] D. Meister F. Hübner M. Mack T.J. Vogl MR thermometry for laser-induced thermotherapy at 1.5 Tesla Rofo 179 2007 497 505 [30] Y. Kurumi T. Tani S. Naka MR-guided microwave ablation for malignancies Int J Clin Oncol 217 2007 85 93 [31] S.G. Silverman K. Tuncali D.F. Adams MR imaging-guided percutaneous cryotherapy of liver tumors: initial experience Radiology 2000 657 664 [32] A. Carpentier R.J. McNichols R.J. Stafford Real-time magnetic resonance-guided laser thermal therapy for focal metastatic brain tumors Neuro-surgery 63 2008 ONS21–8 [33] J. Marsh J. Greenwood Real-time AI: software architecture issues Conf Proc IEEE NAECON 1986 67 77
更多查看译文
关键词
MR guidance,Open MRI,Interventional MRI,Real-time imaging
AI 理解论文
溯源树
样例
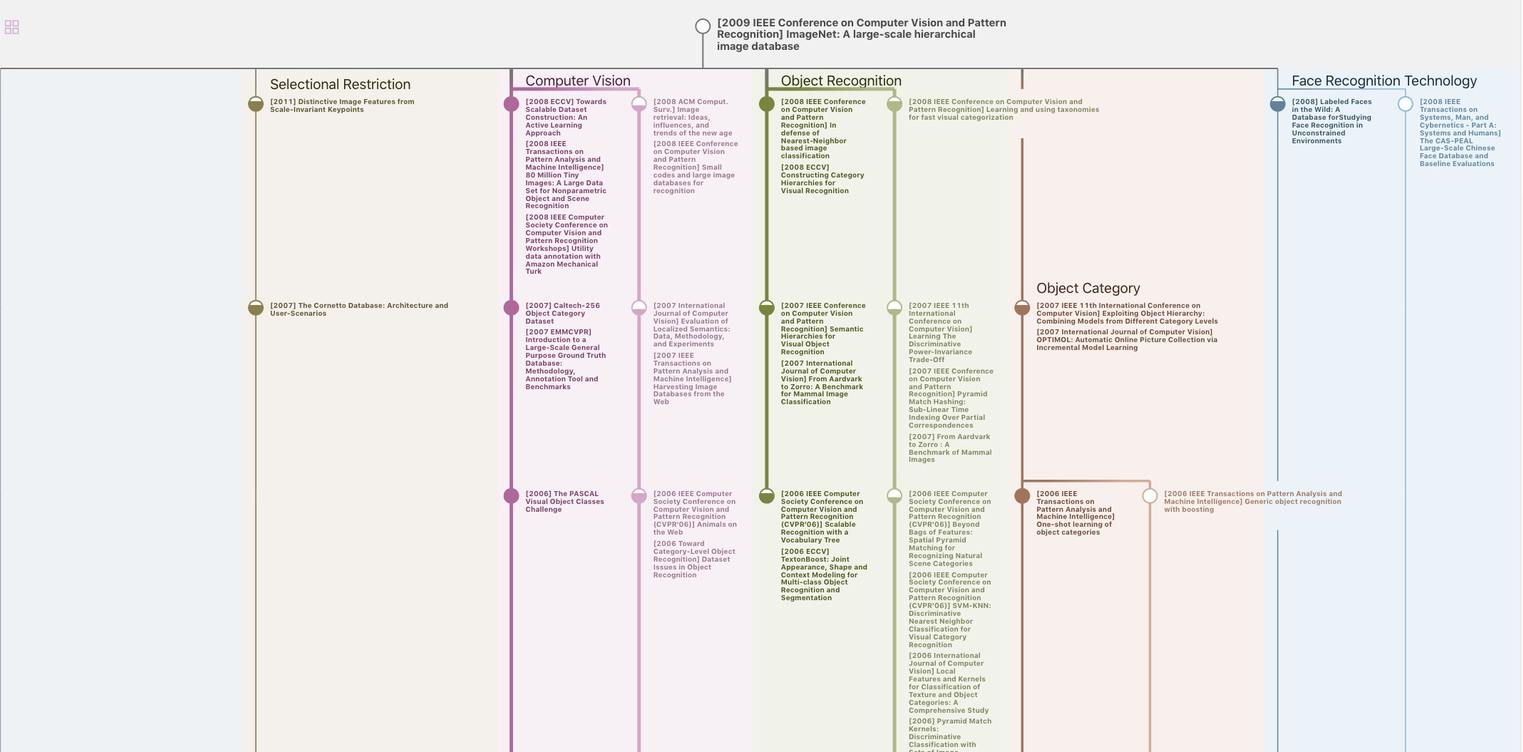
生成溯源树,研究论文发展脉络
Chat Paper
正在生成论文摘要