Activation and survival of immature neurons in the dentate gyrus with spatial memory is dependent on time of exposure to spatial learning and age of cells at examination
Neurobiology of Learning and Memory(2011)
摘要
Neurogenesis continues to occur throughout life in the dentate gyrus of the hippocampus and may be related to hippocampus-dependent learning. We have recently reported that there is an enhancement of neurogenesis in the hippocampus only when BrdU is administered 6 days prior to starting spatial training but not when training started either 1 day or 11 days following BrdU administration. In that study, all rats were perfused on day 16 after BrdU injection in order to compare cells of the same age (i.e. 16 day old cells) and thus the survival time after learning was different between groups. This study was designed to address whether the amount of time that passed following training could also contribute to the effects of spatial learning on hippocampal neurogenesis and whether there was differential new neuron activation in response to spatial learning that depended on the age of new cells at the time of spatial learning. Here we tested whether a survival period of 5 days following spatial learning at either 1–5, 6–10 or 11–15 days following BrdU administration would alter cell survival and/or activation of new neurons. Our results indicate that 5 days after training in the Morris water task cell survival is unaltered by training on days 1–5, increased by training at days 6–10 and decreased when training occurs on days 11–15. Furthermore spatial learners trained on days 6–10 or 11–15 show greater activation of new neurons compared to cue-trained rats during a probe trial 5 days after training. In addition, rats trained on the spatial task on days 11–15 had a greater number of activated new neurons compared to rats trained on the spatial task on days 6–10. These results suggest there is a gradual removal of older BrdU-labeled new neurons following spatial learning perhaps due to a competitive interaction with a population of younger BrdU-labeled new neurons. Keywords Neurogenesis Hippocampus Dentate gyrus Morris water task Critical period Immediate early genes fos BrdU 1 Introduction It is now widely accepted that in the adult mammalian brain, neurogenesis occurs throughout life in the dentate gyrus of the hippocampus ( Altman & Das, 1965; Cameron, Woolley, McEwen, & Gould, 1993; Eriksson et al., 1998; Kaplan & Hinds, 1977 ). The function of these new cells remains uncertain and controversial. However, mounting evidence points towards a role in hippocampus-dependent learning and memory (for review see Leuner, Gould, and Shors (2006) ). New neurons produced in the subgranular zone of the dentate gyrus migrate into the granule cell layer where they integrate and extend axons into the CA3 region, ( Hastings & Gould, 1999; Markakis & Gage, 1999; Stanfield & Trice, 1988 ), become electrophysiologically active and mature into functional granule cells ( van Praag et al., 2002 ). Numerous studies have demonstrated that training rats in certain hippocampus-dependent tasks alters the number of cells that survive to maturity ( Ambrogini et al., 2000; Epp, Haack, & Galea, 2009; Epp, Spritzer, & Galea, 2007; Gould, Beylin, Tanapat, Reeves, & Shors, 1999; Hairston et al., 2005; Leuner et al., 2004; Olariu, Cleaver, Shore, Brewer, & Cameron, 2005 ). Gould and colleagues (1999) first showed that rats trained in a spatial navigation version of the Morris water task (MWT) had a greater number of new surviving cells compared to rats trained in a non-spatial (hippocampus-independent) version of the MWT. However, several studies have reported that hippocampus-dependent learning does not alter cell survival ( Dobrossy et al., 2003; Mohapel, Mundt-Petersen, Brundin, & Frielingsdorf, 2006; Van der Borght, Wallinga, Luiten, Eggen, & Van der Zee, 2005 ) or decreases cell survival following spatial learning ( Ambrogini, Orisini et al., 2004; Epp et al., 2009 ). We and others have shown that the effect of spatial learning on neurogenesis in the hippocampus is dependent on a variety of factors such as timing, quality of learning and task difficulty ( Epp et al., 2007, 2009; Sisti, Glass, & Shors, 2007 ). Recent evidence suggests that timing may be critical in explaining why only some studies have found enhanced cell survival in response to spatial learning. We have recently demonstrated that spatial learning increases cell survival specifically when training occurs 6–10 days following bromodeoxyuridine (BrdU) administration but there was no change in cell survival when training occurred either 1–5 days or 11–15 days after BrdU administration ( Epp et al., 2007 ). However, another study described a decrease in cell survival following spatial learning that occurred 11–15 days following BrdU administration ( Ambrogini, Orisini et al., 2004 ). One possible reason for this discrepancy may be the length of time between training and examination of new cells in the dentate gyrus. In the Ambrogini (2004) study, BrdU-labeled cells were examined three days after training on days 11–15 while in our study BrdU-labeled cells were examined one day after training ( Epp et al., 2007 ). This difference in the time after training may have allowed for a delayed decrease in cell survival in the Ambrogini study that had not yet occurred one day following training in our earlier study. The first aim of the current study was to determine whether the time windows between labeling new cells with BrdU, training and examination of cells is important for detecting changes in cell survival. The second aim of the current study was to examine whether changes in cell survival that result from spatial learning at different times after BrdU administration cause a change in the number of new cells that are activated by memory retrieval. Several studies have used immediate early gene (IEG) activation as a marker of cellular activation in new neurons in response to hippocampus-dependent learning and memory ( Kee, Teixeira, Wang, & Frankland, 2007; Ramirez-Amaya, Marrone, Gage, Worley, & Barnes, 2006; Snyder, Choe et al., 2009; Snyder, Radik, Wojtowicz, & Cameron, 2009; Tashiro, Makino, & Gage, 2007 ). In the current study we sought to determine whether the critical period for enhancing cell survival (6–10 days after BrdU administration) has a corresponding increase in activation of these new cells compared to training on days 1–5 or 11–15. To address these aims, we trained rats in the MWT at one of three time points following BrdU administration (1–5, 6–10 or 11–15 days) and examined all groups five days following training in order to allow sufficient time for any delayed changes in cell survival to occur after the completion of training. Two hours prior to perfusion all rats were given a single probe trial in the MWT to assess spatial memory and to determine whether memory retrieval differentially activates new neurons dependent on the age of the cells at the time of training. 2 Materials and methods 2.1 Subjects Subjects were 75 male Sprague Dawley rats (Charles River; Saint-Constant, Quebec, Canada) 70–75 days old and weighed between 300 and 350 g at the beginning of testing. Rats were housed individually in standard cages with a polyvinylchloride tube, paper towels, cedar bedding and free access to food and water. Rats were habituated to their housing conditions for 1 week, and then were handled five minutes per day for 5 days prior to the start of the experiment. All testing was carried out in accordance with the Canadian Council for Animal Care guidelines and was approved by the animal care committee at the University of British Columbia. All efforts were made to reduce the number of animals used and to minimize their suffering. 2.2 Apparatus A circular pool, 180 cm in diameter, was filled with approximately 21 °C water to a depth of 30 cm. Nontoxic white tempura paint was added to the water to render it opaque. Numerous large cues were placed around the room on all sides of the pool (see Epp et al., 2009 ). A camera mounted above the pool was connected to Anymaze tracking software (Stoelting Co; Wood Dale, IL, USA) which was used to record the distance to reach the platform during training, as well as the percentage of time spent in each quadrant and in the vicinity of the platform location during the probe trial. 2.3 Procedure All rats were given a single intraperitoneal injection of BrdU (200 mg/kg; Sigma, Oakville, ON, Canada) on day 0 of the experiment. Then, rats were divided into three groups to be tested at three different time points, days 1–5 (Cue n = 16; Place n = 16), 6–10 (Cue n = 8 Place n = 13) or 11–15 (Cue n = 12; Place n = 10) after BrdU administration (see Table 1 for a timeline of the training procedure). Half of the rats at each time point were trained on the place (hippocampus-dependent) version of the task and half were trained on the cue (hippocampus-independent) version of the task. In the place version of the task, a hidden platform was located in the center of one quadrant of the pool, 2 cm below the surface of the water. In the cue version of the task, the top of the platform extended 2 cm above the surface of the water and moved randomly to the center of a new quadrant following each trial so that a spatial strategy could not be used. In either case, all rats were given one daily training session for 5 days with four trials per session starting from a different one of the four cardinal compass points on each trial. Five days following the final training session all rats were given a single probe trial. During this trial the rats swam for 30 s in the same pool without an escape platform to assess retention of the platform location. We compared the amount of time spent in the quadrant of the pool that had previously contained the hidden platform (the target quadrant) as a measure of probe trial performance. We also compared the amount of time spent in a smaller area comprising 10% of the pool area centered over the platform location (the platform zone). Two hours following the probe trial the rats were deeply anesthetized with sodium pentobarbital and then were perfused transcardially with 60 ml of 0.9% saline followed by 120 ml of 4% formaldehyde (Sigma) in 0.1 M phosphate buffered saline (PBS; Sigma). The brains were extracted and placed in 4% formaldehyde solution for 24 h and then were transferred into a solution containing 30% sucrose (Sigma) in 0.1 M PBS for at least four days. A Leica vibratome (VT1000S; Richmond Hill, ON, Canada) was used to section the brains. Throughout the rostral–caudal extent of the hippocampus 10 series of 40 μm sections were collected. The sections were then stored in antifreeze (ethylene glycol/glycerol; Sigma) at −20 °C until processing. 2.4 Immunohistochemistry 2.4.1 BrdU labeling The tissue was rinsed three times and left overnight in 0.1 M PBS to remove the antifreeze solution. Tissue sections were incubated at room temperature in 0.6% H 2 O 2 (Sigma) for 30 min followed by three rinses in 0.1 M PBS prior to a 30 min incubation at 37 °C in 2 N HCl (Sigma). The tissue was then rinsed in 0.1 M borate buffer for 10 min (pH 8.5) followed by three more rinses in 0.1 M PBS. The tissue sections were then transferred to a primary antibody solution containing 1:200 mouse anti-BrdU (Roche; Mississauga, ON, Canada), 3% normal horse serum (Vector Laboratories; Burlingame, CA, USA) and 1% Triton-X (Sigma) in 0.1 M PBS for 24 h at 4 °C on a shaker. The tissue was rinsed 3 times in 0.1 M PBS and then transferred to a secondary antibody solution containing a 1:500 dilution of horse anti-mouse (Vector Laboratories) in 0.1 M PBS. The tissue was incubated in the secondary solution for 4 h at room temperature. Following three rinses in 0.1 M PBS, an ABC kit (Vector Laboratories) was used and prepared according to the kit instructions. BrdU labeling was visualized by incubating tissue in nickel-enhanced diaminobenzidine (DAB; Sigma) for approximately 3 min. Finally the sections were mounted on glass slides and coverslipped with Permount (Fisher Scientific; Ottawa, ON, Canada). 2.4.2 BrdU/doublecortin double labeling To determine the percentage of new neurons BrdU and doublecortin (DCX) double labeling was performed. The tissue was rinsed three times and left overnight in 0.1 M PBS to remove the antifreeze solution. Tissue was incubated at 4 °C for 24 h in a primary antibody solution containing a 1:1000 dilution of goat anti-DCX (Santa Cruz Biotechnology; Santa Cruz CA, USA), 3% Normal donkey serum (Vector Laboratories) and 3% Triton-X in 0.1 M PBS. The sections were then rinsed three times in PBS and incubated in a solution containing a 1:500 dilution of donkey anti-goat Alexa 488 (Jackson ImmunoResearch; West Grove, PA, USA) in 0.1 M PBS for 16 h at 4 °C. The tissue was rinsed three times in PBS, fixed in 4% paraformaldehyde for 10 min and rinsed three times in 0.9% saline. DNA denaturation was accomplished by incubating the tissue for 30 min at 37 °C in 2 N HCl. The tissue was rinsed three times in PBS prior to incubation in a primary antibody solution containing rat anti-BrdU (AbD Serotec; Raleigh, NC, USA), 3% normal donkey serum and 3% Triton-X in 0.1 M PBS for 24 h at 4 °C. The tissue was then rinsed three times in PBS and then incubated in a secondary antibody solution containing donkey anti-rat Cy3 (Jackson ImmunoResearch) for 16 h at 4 °C. The tissue was rinsed three times, mounted on glass slides and coverslipped with PVA-DABCO. 2.4.3 BrdU/c-fos double labeling To determine the number of activated cells we chose to use the IEG c-fos because this marker has been previously used in a number of studies to examine the activation of new cells ( Kee et al., 2007; Snyder, Choe et al., 2009; Snyder, Radik et al., 2009; Stone et al., 2010; Tashiro et al., 2007 ). In addition, within the dentate gyrus this marker is expressed almost exclusively in neurons ( Kee et al., 2007 ). The tissue was rinsed three times and left overnight in 0.1 M PBS to remove the antifreeze solution. Then, a 1/10 series of tissue was first incubated in 2 N HCl for 30 min at 37 °C and was rinsed three times in PBS. Then the tissue was transferred to a primary antibody solution containing 1:500 mouse anti-BrdU (Roche), 1:1000 rabbit anti-c-fos (Santa Cruz Biotechnology), 3% normal donkey serum and 3% Triton-X in 0.1 M PBS and incubated for 24 h at 4 °C. The tissue was rinsed three times in PBS and transferred to a secondary antibody solution containing 1:1000 dilutions of donkey anti-mouse Alexa 488 (Jackson ImmunoResearch) and donkey anti-rabbit Cy3 (Jackson ImmunoResearch) for 16 h at 4 °C. The tissue was rinsed three times in PBS and was then mounted on glass slides and coverslipped with PVA-DABCO. 2.4.4 Cell counting BrdU-labeled cells were counted in every 10th section throughout the entire granule cell layer (GCL; includes the 50 μm thick subgranular zone) and the hilus to obtain an estimate of the total number of BrdU-labeled cells. The counting of BrdU-labeled cells was performed with a Nikon E600 brightfield microscope and a 100× oil immersion objective lens. To obtain an estimate of the total number of cells we multiplied by the sampling fraction of 10 using the same procedure as in previous studies ( Eadie, Redila, & Christie, 2005; Epp et al., 2007, 2009; Kronenberg et al., 2003 ). The percentage of BrdU/DCX double-labeled cells ( Fig. 1 A) was obtained by selecting 50 BrdU cells arbitrarily from at least five sections per brain and determining whether those cells also expressed DCX. BrdU/c-fos double labeling ( Fig. 1 B) was carried out with the same procedure as for BrdU/DCX labeling except that 100 BrdU-labeled cells were examined in each brain. Fluorescent imaging was performed on a Nikon E600 epifluorescent microscope under 400× magnification. Double labeling was confirmed by confocal microscopy (Olympus, FV10i). Z-sections were captured at 60× magnification to confirm colocalization of BrdU with doublecortin and BrdU with c-fos. 2.4.5 Data analyses All analyses were performed using the software package Statistica v.6 (Statsoft). We analyzed dependent variables of distance to reach the platform and swim speed using repeated-measures analysis of variance (ANOVA). Day of training (1–5, 6–10, 11–15) and type of training (place, cue) were used as between-subjects factors and session (1–5) was the within-subject factor. Volume and number of BrdU-labeled cells were each analyzed with repeated-measures ANOVAs using day of training and type of training as between-subjects factors and region (GCL, hilus) as the within-subjects factor. The percentage of BrdU/DCX and BrdU/c-fos double labeling and probe trial performance (percent time in the target quadrant and platform zone) were analyzed using ANOVAs with time of training and type of training as between-subjects factors. Post-hoc tests utilized the LSD procedure. Pearson product moment calculations were performed on the percentage of BrdU/c-fos labeled cells and the percentage of time in the platform zone and target quadrant during the probe trial. 3 Results 3.1 Place-trained rats had longer swim distances in the Morris water task than cue-trained rats Rats trained on the place task swam further (greater distances) to reach the platform than rats trained on the cue version of the task across all trials (main effect of training on total distance swum: F (1, 51) = 260.30, P ⩽ 0.000001; but no other significant effects P ’s ⩾ 0.35; Fig. 2 D). For distance traveled to reach the platform across sessions there was a three-way interaction between session, day of training and type of training, F (8204) = 2.38, P ⩽ 0.018. Post-hoc tests indicated that cue-trained rats swam shorter distances than place-trained rats during all sessions and at all three time points ( P ⩽ 0.040) except for session five at the 1–5 day time point ( P = 0.091; Fig. 2A–C ). Rats trained at the different time points did not significantly differ in terms of distance traveled ( P ’s ⩾ 0.60). 3.2 Place-trained rats spent more time in the target quadrant and the platform zone than cue-trained rats Place-trained rats spent a greater percentage of time swimming in the target quadrant than cue-trained rats ( Fig. 2 E; main effect of type of training ( F (1, 51) = 10.18, P ⩽ 0.0024) but there were no other significant effects (all P ’s ⩾ 0.38)). Place-trained rats also spent a greater percentage of time in the platform zone compared to cue-trained rats ( Fig. 2 F; main effect of type of training ( F (1, 51) = 17.97, P ⩽ 0.000094) with no other significant main effects or interactions (all P ’s ⩾ 0.17)). 3.3 Morris water task training increased and decreased cell survival depending on the age of the new cells at the time of exposure to training There was a three-way interaction between region, type of training and day of training ( F (2, 51) = 4.54, P ⩽ 0.015). Post-hoc tests showed that place-trained rats had increased cell survival in the GCL (number of BrdU-labeled cells) compared to cue-trained rats when this training occurred on days 6–10 ( P = 0.0084) but decreased cell survival when training occurred on days 11–15 ( P = 0.014; Fig. 3 ). However there was no significant difference in cell survival between place- and cue-trained rats when training occurred on days 1–5 ( P = 0.089). There were no significant differences between place and cue-trained rats in cell survival in the hilus at any of the training time points ( P ⩾ 0.78). We next correlated the number of BrdU-labeled cells in the GCL with the total distance traveled during the training sessions as an indicator of overall learning ( Fig. 3 D). With place training there was a significant negative correlation for rats trained on days 11–15 ( r = −0.75, P = 0.013) but not for rats trained on days 1–5 ( r = −0.55, P = 0.20 ) or days 6–10 ( r = −0.057, P = 0.86). Thus, rats trained on the place task on days 11–15 that had shorter total swim distances had higher numbers of BrdU-labeled cells. There were no significant correlations for rats trained on the cue task on days 1–5 ( r = −0.15, P = 0.75), 6–10 ( r = 0.49, P = 0.22) or 11–15 ( r = −0.057, P = 0.86). 3.4 Place training increases the activation of BrdU-labeled cells We were unable to identify any BrdU/c-fos double-labeled cells in the groups of rats trained on days 1–5 following BrdU administration and perfused on day 10. Thus we statistically analyzed only the 6–10 and 11–15 day time points. In response to a probe trial, rats trained on the place task had a greater activation of BrdU-labeled cells than cue-trained rats, as measured by the percentage of BrdU-labeled cells that co-expressed c-fos, ( Fig. 4 ; main effect of type of training ( F (1, 39) = 4.54, P ⩽ 0.039). As expected, there was greater activation in 20 day old BrdU cells compared to16 day old BrdU cells (main effect of day of training ( F (1, 39) = 26.57, P ⩽ 0.000008 but there were no other significant main or interaction effects P ’s ⩾ 0.05). A priori we predicted that there would be an increase in the number of BrdU-labeled cells activated by place training on days 11–15 (20 day old cells) versus days 6–10 (15 day old cells) based on the reasoning that more of the older BrdU-labeled cells will have matured to the point that they are physically capable to being activated. We found that significantly more BrdU-labeled cells expressed c-fos after place training on days 11–15 compared to place training on days 6–10 ( P = 0.00003) . 3.5 The number of new activated 20 d old neurons was positively correlated with spatial performance in the MWT Next we correlated probe trial performance in the MWT with the percentage of BrdU/c-fos labeled cells. Rats with better retention of the correct quadrant of the pool had a greater number of activated BrdU-labeled cells when trained on days 11–15 l ( r = 0.63, P = 0.05; Fig. 5 ), but not in the platform zone ( P = 0.46). No other significant correlations were found between groups or time of training between percentage of BrdU/c-fos double-labeled cells and percentage of time spent in the target quadrant or the platform zone (all P ’s ⩾ 0.2). We also correlated the percentage of BrdU/c-fos double-labeled cells with the total distance traveled during the training sessions and found a significant positive correlation for rats trained on the place task on days 11–15 ( r = 0.83, P = 0.003). However, there was a significant negative correlation for place-trained rats on days 6–10 between total distance swum and BrdU/c-fos double-labeled cells ( r = −0.59, P = 0.05; with two outliers removed). No significant correlations were found for rats trained on the place task on days 6–10, the cue task on days 6–10 or the cue task on days 11–15 (all P ’s ⩾ 0.3). Finally, we correlated the percentage of BrdU/c-fos double-labeled cells with the total number of BrdU-labeled cells in the GCL and we found a significant negative correlation in the rats trained on the place task on days 11–15 ( r = −0.62, P = 0.05). Thus rats with fewer surviving 20-day-old BrdU-labeled cells following place training on days 11–15 show greater activation of the cells that do survive. There were no other significant correlations between training type or time of training ( P ’s ⩾ 0.08). 3.6 The percentage of DCX/BrdU-labeled cells decreases with the age of the BrdU-labeled cells Not surprisingly, the percentage of BrdU-labeled cells that expressed the immature neuronal marker DCX decreased with age ( Fig. 6 ; main effect of day of training ( F (2, 47) = 239.5, P ⩽ 0.00001) with more BrdU/DCX double-labeled cells at 10 days old (1–5 day training time) compared to 15 and 20 day old cells (the 6–10 day group ( P = 0.00012) and the 11–15 day group ( P ⩽ 0.00013), respectively). There was no significant difference between place and cue-trained rats in the percentage of immature neurons (main effect of type of training ( P = 0.34); day by training interaction effect ( P = 0.27)). We also examined BrdU and NeuN coexpression and found a corresponding increase in double labeling with increasing age of cells but no significant effect of type of training (see Supplementary data ). 3.7 No significant differences in granule cell layer volume between groups There were two significant two-way interactions (region and type of training ( F (2, 51) = 4.29, P ⩽ 0.043) and region and day of training ( F (2, 51) = 3.35, P ⩽ 0.0043) on dentate gyrus volume. Post-hoc tests showed that cue-trained rats had a significantly greater hilus volume compared to place-trained rats ( P = 0.0048; Table 2 ) but no significant difference in GCL volume ( P = 0.15). However post-hoc tests failed to find significant differences in the volumes of the GCL ( P ’s ⩾ 0.76) or hilus ( P ’s ⩾ 0.078) at any of the training time points. There was also a significant main effect of type of training ( F (1, 51) = 11.90, P ⩽ 0.0011) and a significant main effect of region ( F (1, 51) = 1059.48, P ⩽ 0.000001). All other main or interaction effects were not significant ( P ’s ⩾ 0.35). 4 Discussion The goal of the current study was to determine whether spatial learning altered cell survival and the activation of new cells dependent on the developmental stage of the new cells at time of exposure. Our current results demonstrate that spatial training, compared to cue training, in the MWT enhanced cell survival but only when training occurred 6–10 days following BrdU administration. Furthermore, spatial learning decreased cell survival compared to cue training when the rats received training 11–15 days following BrdU administration and cells were examined 5 days after training. Early spatial training (days 1–5) was found to have no significant effect on cell survival. Furthermore, place training, in comparison to cue training, increased activation of new cells that were at least 15 days old. 4.1 Cell survival may respond to spatial learning in a competitive fashion The decrease in neurogenesis in the hippocampus of place-trained rats at 11–15 days is in agreement with the findings of Ambrogini, Orisini et al., 2004 but differed from our previous results ( Epp et al., 2007 ). The fact that we observed a decrease in survival of BrdU-labeled cells when rats were trained 11–15 after BrdU in the current study may be due to the fact that we allowed a period of 5 days instead of 1 day ( Epp et al., 2007 ) to elapse following training before perfusing rats. A delayed decrease in cell survival has been seen before in response to NMDA-receptor activation. Tashiro and colleagues (2006) demonstrated that while the survival of new cells is dependent on NMDA-receptor activation, death of new cells that do not receive NMDA-receptor activation did not occur until 18 days post infection. In addition Ambrogini and colleagues (2004) also found an increase in apoptosis following spatial training compared to non-spatial training. Thus it may take several days following spatial training for the cells that are not incorporated into the hippocampus to be removed via cell death pathways and it is possible that the additional days following training (5 days in the present study) versus 1 day ( Epp et al., 2007 )) allowed sufficient time for the removal of degenerating BrdU-labeled cells. Interestingly, a recent study has demonstrated that spatial learning requires both an increase and a reduction in cell survival depending on the age of the new cells ( Dupret et al., 2007 ). In the present study we did not examine cell death because any cells that underwent cell death in direct response to training in the Morris water task would likely have been cleared from the brain by the time of perfusion (5 days after training). Although it is not immediately clear why cell survival would have been reduced when training occurred on days 11–15 and perfusions occurred on day 20 it may be due to an interaction/competition with other non-labeled populations of cells. In rats injected with BrdU 11–15 days before training there is a population of cells 6–10 days old that may preferentially be incorporated into the hippocampus as a result of spatial learning. As a result, the older population of new cells receives less input and is eliminated in a competitive manner. This interpretation is consistent with findings showing that the selective integration of new neurons is based on competition for NMDA-receptor activation ( Tashiro et al., 2006 ). A previous study has also shown that spatial learning may both increase and decrease cell survival ( Dobrossy et al., 2003 ). In that study, it was demonstrated that the early phase of learning (the first 4 days of training) caused an increase in cell survival but continued training (a further 4 days) resulted in a compensatory decrease in cell survival. It is important to note that the findings of the present study and that of Dobrossy et al. (2003) show that there is likely a competitive interaction between new cells of different ages that are exposed to spatial learning. Although it is possible that there is only minimal glutamatergic connectivity in 6–10 day old cells ( Esposito et al., 2005 ), the change in cell survival that occurs with training on days 6–10 could also be due to GABAergic transmission (which begins by 8 days of age in mice; Esposito et al., 2005 ). Spatial exploration can increase GABAergic transmission from interneurons onto the dendrites of granule cells ( Moser, 1996 ). Thus, it is possible that spatial learning may have an excitatory impact on immature granule cells through this type of mechanism because immature neurons are excited by GABA ( Ge, Pradhan, Ming & Song, 2007 ). Although one could argue that cell survival was increased in place compared to cue-trained rats because place-trained rats swam greater distances to reach the platform compared to cue-trained rats, we do not believe this to be the case for several reasons. First in order to see an increase in cell survival one needs to see a minimum of 7 days of 1 h of wheel running a day ( Holmes, Galea, Mistlberger, & Kempermann, 2004 ). In our study the amount of exercise amounted to less than 20 min of swimming over 5 days. Furthermore training on days 6–10 after BrdU increased cell survival while training on days 11–15 after BrdU decreased cell survival and there were no significant differences in distance swum between groups indicating that despite similar levels of exercise spatial learning at different time points resulted in opposed effects on cell survival. 4.2 Immature neurons are activated following hippocampus-dependent memory recall In the present study we also demonstrate that compared to cue training, rats trained on the place version of the MWT have a greater percentage of new neurons that are activated following a probe trial. The increase in BrdU/c-fos co-labeled cells in place-trained rats compared to cue-trained rats shows that new neurons are activated by spatial memory retrieval and may indicate that the new neurons could play a role in the retention of spatial memories. Regardless of type of training there was also an increase in the number of BrdU-labeled cells that express c-fos at the 11–15 day time point. This is likely due to the fact that the cells examined in the 11–15 day group were more mature (20 day old compared to 15 day old cells in the 6–10 group) at the time of learning and retention testing. In mice, the onset of glutamatergic connectivity occurs once the immature neurons are approximately 18 days old ( Esposito et al., 2005 ). However, this may occur earlier in rats as it has been shown that new neurons develop slightly faster in terms of IEG activation in rats compared to mice ( Snyder, Choe et al., 2009; Snyder, Radik et al., 2009 ). Thus, the activated cells observed in the rats trained on days 6–10 and perfused on day 15 may be active in response to the probe trial only. However, the cells activated in the rats trained on days 11–15 and perfused on day 20 may be active in response to retrieval of the previously acquired memory. This would provide a potential explanation for why we saw greater activation in place versus cue learning in the 11–15 day group but not in the 6–10 day group. The number of activated 20-day-old cells may be predictive of how well the hidden platform location is remembered. Only rats trained on the place task on days 11–15 and given a probe trial on day 20 had evidence of a positive correlation between probe trial performance and the percentage of BrdU-labeled cells expressing c-fos. This indicates that although there are fewer remaining BrdU-labeled cells those new cells that do survive are more likely to be used in the formation of new memory traces. Because the BrdU/c-fos double labeling at the 6–10 day time point did not correlate significantly with memory retention (on day 15) it is possible that although more of these cells survive they are not as likely as a slightly older population of cells to participate in the formation or retention of spatial memories. Alternatively, it may simply be the case that fewer of the cells from the 6 to 10 day training group were activated because they were younger and were possibly too young to be effective at either the time of training or memory recall. This is certainly a possibility and fits with the complete absence of activated cells following training on days 1–5. In the current study we did not utilize an untrained control group. However, previously we found that using the same training procedures that increased activation of new neurons in response to spatial memory is seen in place-trained rats compared to both cue-trained and untrained rats, and that there was no significant difference between these groups ( Epp, Scott, & Galea, 2011 ). 4.3 Impact of quality of learning on cell survival Several previous studies have examined whether the survival of BrdU-labeled cells is related to the quality of learning ( Dobrossy et al., 2003; Epp et al., 2007; Sisti et al., 2007 ). Although there is some indication that poor learning produces greater cell survival ( Dalla, Papachristos, Whetstone, & Shors, 2009; Dobrossy et al., 2003; Epp et al., 2007 ) this is not conclusive because another study has shown that cell survival is enhanced in good learners but not poor learners ( Sisti et al., 2007 ). In the current study we found that better performance is associated with greater numbers of BrdU-labeled cells in place-trained rats only when trained on days 11–15. Thus the current data lend support to the idea the age of the new cells at the time of training may be one factor that interacts with the quality of learning and the number of surviving cells. In addition we also found that more activated new neurons were associated with better memory for the platform (but worse performance during training) when training occurred on days 11–15. However, when training occurred on days 6–10 the opposite relationship between learning ability and activation of BrdU-labeled cells was found. At the earlier time point (days 6–10) better learning was related to more activated cells during the probe trial. This provides further support of a differential effect of quality of learning on the incorporation and function of new neurons dependent on the age of the cells at the time of training. 5 Concluding remarks In summary, our main findings are that spatial learning has different effects on the survival of cells and activation of new cells when training occurs at different times during cell maturation. Training that occurs when cells are very immature (days 1–5) has no effect on cell survival, training at an intermediate age (days 6–10) increases cell survival and late training (days 11–15) decreases cell survival when cells are examined 5 days after training. Finally, the current study provides evidence that despite a decrease in cell survival with late training, cells of this age are activated by recall of the spatial location of the hidden platform. Thus it appears that the percentage of new cells that are active may be more important than the total number of new cells that survive following spatial learning. Acknowledgements The authors wish to thank Stephanie Lieblich for technical assistance and Dr. Adele Diamond for stimulating discussions on this topic. Funding for this research was provided by a Discovery operating grant from NSERC to LAMG. JRE is the recipient of a Michael Smith Foundation for Medical Research senior trainee award. LAMG is a Michael Smith senior scholar. Appendix A Supplementary material Supplementary data associated with this article can be found, in the online version, at doi:10.1016/j.nlm.2011.01.001 . Appendix A Supplementary material Supplemenatry data The percentage of NeuN/BrdU-labeled cells increases with the age of the BrdU-labeled cells. References Altman and Das, 1965 J. Altman G.D. Das Autoradiographic and histological evidence of postnatal hippocampal neurogenesis in rats Journal of Comparative Neurology 124 1965 319 335 Ambrogini et al., 2000 P. Ambrogini R. Cuppini C. Cuppini S. Ciaroni T. Cecchini P. Ferri Spatial learning affects immature granule cell survival in adult rat dentate gyrus Neuroscience Letters 286 2000 21 24 Ambrogini et al., 2004 P. Ambrogini L. Orsini C. Mancini P. Ferri S. Ciaroni R. Cuppini Learning may reduce neurogenesis in adult rat dentate gyrus Neuroscience Letters 359 2004 13 16 Cameron et al., 1993 H.A. Cameron C.S. Woolley B.S. McEwen E. Gould Differentiation of newly born neurons and glia in the dentate gyrus of the adult rat Neuroscience 56 1993 337 344 Dalla et al., 2009 C. Dalla E.B. Papachristos A.S. Whetstone T.J. Shors Female rats learn trace memories better than male rats and consequently retain a greater proportion of new neurons in their hippocampi Proceedings of the National Academy Sciences of the United States of America 106 8 2009 2927 2932 Dobrossy et al., 2003 M.D. Dobrossy E. Drapeau C. Aurousseau M. Le Moal P.V. Piazza D.N. Abrous Differential effects of learning on neurogenesis: Learning increases or decreases the number of newly born cells depending on their birth date Molecular Psychiatry 8 2003 974 982 Dupret et al., 2007 D. Dupret A. Fabre M.D. Dobrossy A. Panatier J.J. Rodriguez S. Lamarque Spatial learning depends on both the addition and removal of new hippocampal neurons Public Library of Science Biology 5 2007 e214 Eadie et al., 2005 B.D. Eadie V.A. Redila B.R. Christie Voluntary exercise alters the cytoarchitecture of the adult dentate gyrus by increasing cellular proliferation, dendritic complexity, and spine density Journal of Comparative Neurology 486 2005 39 47 Epp and Haack, 2009 J.R. Epp A.K. Haack L.A. Galea Task difficulty in the Morris water task influences the survival of new neurons in the dentate gyrus Hippocampus 20 2009 866 876 Epp et al., 2011 J.R. Epp N.A. Scott L.A. Galea Strain differences in neurogenesis and activation of new neurons in the dentate gyrus in response to spatial learning Neuroscience 172 2011 342 354 Epp et al., 2007 J.R. Epp M.D. Spritzer L.A. Galea Hippocampus-dependent learning promotes survival of new neurons in the dentate gyrus at a specific time during cell maturation Neuroscience 149 2007 273 285 Eriksson et al., 1998 P.S. Eriksson E. Perfilieva T. Bjork-Eriksson A.M. Alborn C. Nordborg D.A. Peterson Neurogenesis in the adult human hippocampus Nature Medicine 4 1998 1313 1317 Esposito et al., 2005 M.S. Esposito V.C. Piatti D.A. Laplagne N.A. Morgenstern C.C. Ferrari F.J. Pitossi Neuronal differentiation in the adult hippocampus recapitulates embryonic development J Neurosci 25 2005 10074 10086 Ge et al., 2007 S. Ge D.A. Pradhan G.L. Ming H. Song GABA sets the tempo for activity-dependent adult neurogenesis Trends Neurosci 30 2007 1 8 Gould et al., 1999 E. Gould A. Beylin P. Tanapat A. Reeves T.J. Shors Learning enhances adult neurogenesis in the hippocampal formation Nature Neuroscience 2 1999 260 265 Hairston et al., 2005 I.S. Hairston M.T. Little M.D. Scanlon M.T. Barakat T.D. Palmer R.M. Sapolsky Sleep restriction suppresses neurogenesis induced by hippocampus-dependent learning Journal of Neurophysiology 94 2005 4224 4233 Hastings and Gould, 1999 N.B. Hastings E. Gould Rapid extension of axons into the CA3 region by adult-generated granule cells Journal of Comparative Neurology 413 1999 146 154 Holmes et al., 2004 M.M. Holmes L.A. Galea R.E. Mistlberger G. Kempermann Adult hippocampal neurogenesis and voluntary running activity: Circadian and dose-dependent effects Journal of Neuroscience Research 76 2 2004 216 222 Kaplan and Hinds, 1977 M.S. Kaplan J.W. Hinds Neurogenesis in the adult rat: Electron microscopic analysis of light radioautographs Science 197 1977 1092 1094 Kee et al., 2007 N. Kee C.M. Teixeira A.H. Wang P.W. Frankland Preferential incorporation of adult-generated granule cells into spatial memory networks in the dentate gyrus Nature Neuroscience 10 2007 355 362 Kronenberg et al., 2003 G. Kronenberg K. Reuter B. Steiner M.D. Brandt S. Jessberger M. Yamaguchi Subpopulations of proliferating cells of the adult hippocampus respond differently to physiologic neurogenic stimuli Journal of Comparative Neurology 467 2003 455 463 Leuner et al., 2006 B. Leuner E. Gould T.J. Shors Is there a link between adult neurogenesis and learning? Hippocampus 16 2006 216 224 Leuner et al., 2004 B. Leuner S. Mendolia-Loffredo Y. Kozorovitskiy D. Samburg E. Gould T.J. Shors Learning enhances the survival of new neurons beyond the time when the hippocampus is required for memory Journal of Neuroscience 24 2004 7477 7481 Markakis and Gage, 1999 E.A. Markakis F.H. Gage Adult-generated neurons in the dentate gyrus send axonal projections to field CA3 and are surrounded by synaptic vesicles Journal of Comparative Neurology 406 1999 449 460 Mohapel et al., 2006 P. Mohapel K. Mundt-Petersen P. Brundin H. Frielingsdorf Working memory training decreases hippocampal neurogenesis Neuroscience 142 2006 609 613 Moser, 1996 E.I. Moser Altered inhibition of dentate granule cells during spatial learning in an exploration task J Neurosci 16 1996 1247 1259 Olariu et al., 2005 A. Olariu K.M. Cleaver L.E. Shore M.D. Brewer H.A. Cameron A natural form of learning can increase and decrease the survival of new neurons in the dentate gyrus Hippocampus 15 2005 750 762 Ramirez-Amaya et al., 2006 V. Ramirez-Amaya D.F. Marrone F.H. Gage P.F. Worley C.A. Barnes Integration of new neurons into functional neural networks Journal of Neuroscience 26 2006 12237 12241 Sisti et al., 2007 H.M. Sisti A.L. Glass T.J. Shors Neurogenesis and the spacing effect: Learning over time enhances memory and the survival of new neurons Learning & Memory 14 2007 368 375 Snyder et al., 2009 J.S. Snyder J.S. Choe M.A. Clifford S.I. Jeurling P. Hurley A. Brown Adult-born hippocampal neurons are more numerous, faster maturing, and more involved in behavior in rats than in mice Journal of Neuroscience 29 2009 14484 14495 Snyder et al., 2009 J.S. Snyder R. Radik J.M. Wojtowicz H.A. Cameron Anatomical gradients of adult neurogenesis and activity: Young neurons in the ventral dentate gyrus are activated by water maze training Hippocampus 19 2009 360 370 Stanfield and Trice, 1988 B.B. Stanfield J.E. Trice Evidence that granule cells generated in the dentate gyrus of adult rats extend axonal projections Experimental Brain Research 72 1988 399 406 Stone et al., 2010 S.S. Stone C.M. Teixeira K. Zaslavsky A.L. Wheeler A. Martinez-Canabal A.H. Wang Functional convergence of developmentally and adult-generated granule cells in dentate gyrus circuits supporting hippocampus-dependent memory Hippocampus 2010 10.1002/hipo.20845 [Epub ahead of print] Tashiro et al., 2007 A. Tashiro H. Makino F.H. Gage Experience-specific functional modification of the dentate gyrus through adult neurogenesis: A critical period during an immature stage Journal of Neuroscience 27 2007 3252 3259 Tashiro et al., 2006 A. Tashiro V.M. Sandler N. Toni C. Zhao F.H. Gage NMDA-receptor-mediated, cell-specific integration of new neurons in adult dentate gyrus Nature 442 2006 929 933 Van der Borght et al., 2005 K. Van der Borght A.E. Wallinga P.G. Luiten B.J. Eggen E.A. Van der Zee Morris water maze learning in two rat strains increases the expression of the polysialylated form of the neural cell adhesion molecule in the dentate gyrus but has no effect on hippocampal neurogenesis Behavioral Neuroscience 119 2005 926 932 van Praag et al., 2002 H. van Praag A.F. Schinder B.R. Christie N. Toni T.D. Palmer F.H. Gage Functional neurogenesis in the adult hippocampus Nature 415 2002 1030 1034
更多查看译文
关键词
Neurogenesis,Hippocampus,Dentate gyrus,Morris water task,Critical period,Immediate early genes,fos,BrdU
AI 理解论文
溯源树
样例
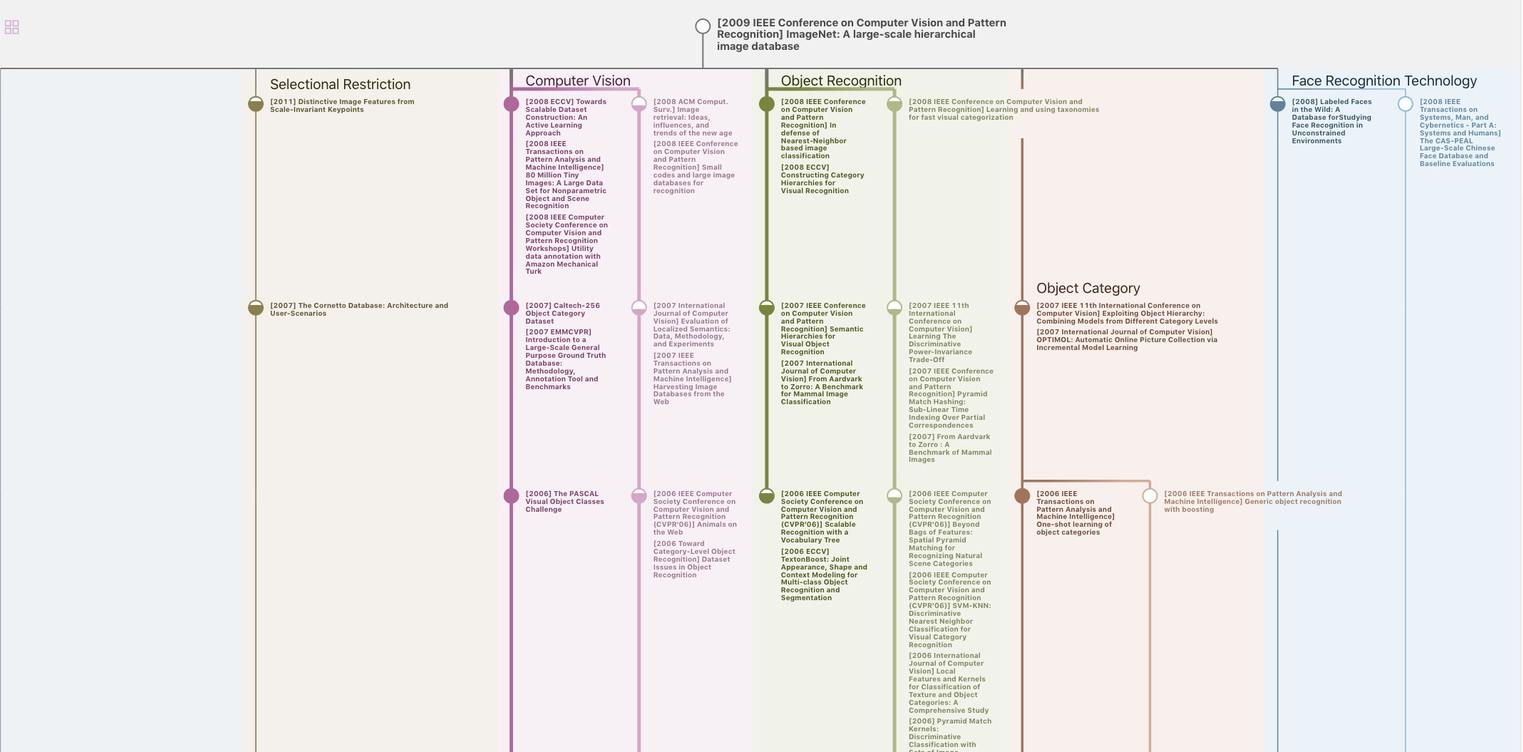
生成溯源树,研究论文发展脉络
Chat Paper
正在生成论文摘要