Compound analysis of gallstones using dual energy computed tomography—Results in a phantom model
European Journal of Radiology(2010)
摘要
Materials and methods 35 gallstones were analyzed with DECT using a phantom model. Stone samples were put into specimen containers filled with formalin. Containers were put into a water-filled cylindrical acrylic glass phantom. DECT scans were performed using a tube voltage/current of 140 kV/83 mAs (tube A) and 80 kV/340 mAs (tube B). ROI-measurements to determine CT attenuation of each sector of the stones that had different appearance on the CT images were performed. Finally, semi-quantitative infrared spectroscopy (FTIR) of these sectors was performed for chemical analysis. Results ROI-measurements were performed in 45 different sectors in 35 gallstones. Sectors containing >70% of cholesterol and no calcium component ( n = 20) on FTIR could be identified with 95% sensitivity and 100% specificity on DECT. These sectors showed typical attenuation of −8 ± 4 HU at 80 kV and +22 ± 3 HU at 140 kV. Even the presence of a small calcium component (<10%) hindered the reliable identification of cholesterol components as such. Conclusion Dual energy CT allows for reliable identification of gallstones containing a high percentage of cholesterol and no calcium component in this pre-clinical phantom model. Results from in vivo or anthropomorphic phantom trials will have to confirm these results. This may enable the identification of patients eligible for non-surgical treatment options in the future. Keywords Dual energy computed tomography Compound analysis Tissue differentiation Gallstones Cholesterol 1 Introduction Gallstones and their medical consequences represent a relevant cost factor in healthcare systems of Western countries. In Germany, 10.5–24.5% of the female and 4.9–13.1% of the male population are estimated to carry gallstones, and about 170,000 cholecystectomies are performed annually [1] . In the United States of America, gallstone disease causes over 700,000 cholecystectomies per year, which is reflected in the health care budget with annual expenses of 6.5 billion USD [2] . The diagnosis of gallstone disease is usually made by clinical presentation, laboratory constellation, and the proof of stone by imaging modalities (mostly ultrasound, endoscopic retrograde or magnetic resonance cholangio-pancreaticography, or computed tomography). Most of the persons carrying gallstones will never develop symptoms. But if gallstone disease becomes evident – mostly as acute cholecystitis or biliary colic – the therapy is very straight forward: whenever possible, definite cure will be sought by removing stones. However, not every patient is eligible for invasive treatment due to personal risk profiles. Further, surgical treatment bears a certain potential of risk inherent in its invasive nature and required anaesthesia. For the above reasons, early detection of gallstones, that may be suitable for non-invasive treatment options, has always been of great interest. Established methods are extra-corporal shockwave lithotripsy (ESWL) and pharmacological dissolution therapy with gall acids [3,4] – either alone or in combination – and contact dissolution with methyl tert-butyl ether [5] . Recently, first experiences with ezetimibe could show promising results [6] . However, for successful dissolution the identification of high content cholesterol stones is important, as only these stones seem to show satisfying result [3–13] . In the past, several authors have described computed tomography (CT) for compound differentiation of gallstones [9,14–22] . Results were inconsistent in terms of reliable differentiation of cholesterol to other components, because confidence intervals overlapped, attenuation thresholds to define a cholesterol stone varied, and scanner settings and methods for chemical analysis differed markedly. Further, regular single source scanners with only one X-ray tube were used and stones were mainly scanned at only one predefined tube voltage. Recently a dual source CT (DSCT) system has become available for clinical routine [23,24] . The possibility to operate both tubes at different potentials (dual energy CT; DECT) has brought the option for material and tissue differentiation into clinical routine [25,26] . In this proof-of-concept study, we investigated the potential of DECT for compound analysis of gallstones in a phantom model under optimized in vitro conditions. Findings were compared to semi-quantitative Fourier transform infrared spectroscopy (FTIR), which served as the reference standard for chemical gallstone analysis. The main goal of this study was to define parameters that could reliably define gallstone components with a high content (>70%) of cholesterol and no calcium components (“pure” cholesterol components) on DECT. 2 Materials and methods 2.1 Sample collection and phantom model This study was approved by the ethic committee of the hospital and in compliance with the declaration of Helsinki. In total, 35 gallstones were analyzed with dual energy computed tomography (DECT) and semi-quantitative Fourier transform infrared spectroscopy (FTIR). The stones were taken from a gallstone collection from the Department of Forensic Medicine of our hospital which was built up for teaching purposes. Samples were collected from autopsies performed at the department over a time span of 2 years. Every sample originated from the gallbladder of a different corpse. The gallstones differed from size, colour, surface, and shape. Right after collection, each sample was put into a separate standard plastic specimen container (length: 5.5 cm, diameter: 3.3 cm) filled with regular 10% formalin solution to prevent stones from drying and rotting. A potential interaction of gallstone components with formalin needs to be considered and might be overcome by storage in native bile or freezing stones after collection. However, with the underlying source of gallstones for this study, this shortcoming was inevitable this time. All stones of this collection were completely anonymous, as no patient related information was documented at the time of collection. Further, the samples for this study were randomly numbered. In our experimental set-up, the specimen containers were put into a regular cylindrical acrylic glass phantom with a diameter of 20 cm and a length of 30 cm. The phantom was filled with regular drinking water at room temperature. For one scan, six containers were lined up in a row along the z -axis. Hence, for 35 samples, in total 6 scans were performed. The phantom was orientated in x - and y -plane so that the stones were placed in the isocenter of the scanner. 2.2 Dual energy computed tomography physical background and scan protocol A commercially available dual source CT scanner (Somatom Definition, Siemens Healthcare, Forchheim, Germany) equipped with 2 X-ray tubes (tubes A and B) and 2 detector arrays mounted perpendicularly to each other within the gantry was used for CT analysis. Both tubes can be operated at different potentials at the same time, generating different X-ray spectra (dual energy CT). With this setting, it is possible to scan the very same voxel at the same time at two different spectra. Attenuation depends beside density, and chemical composition also on the X-ray spectrum a material is exposed to an effect that is routinely used for plane film X-ray depending on clinical questions and modality (mammography vs. chest X-ray vs. conventional trauma imaging). On CT, some materials show characteristic changes in CT value (Hounsfield Units; HU) depending on the X-ray spectrum exposed to [27] . Based on this physical background, it is theoretically possible to differentiate between materials and tissues by this so called dual energy effect. Scans were performed operating the dual source CT system in dual energy mode using a dual energy abdomen protocol with the following settings: tube voltage/current of 140 kV/83 mAs on tube A and 80 kV/340 mAs on tube B, gantry rotation time of 0.5 s, and a pitch of 0.55. As the underlying experiment set-up with a 20 cm water phantom represents a noise optimized condition, a collimation of 2 × 32 × 0.6 mm on both detectors was used for improved spatial resolution and less influence of partial volume averaging. For regular clinical in-patient use a collimation of 14 × 1.2 mm is recommended by the manufacturer and in recent literature [25] , because of higher expected noise and cross scattered radiation. Three image series were reconstructed, one series each for 80 kV, 140 kV, and one mixed series merging 70% of the 140 kV and 30% of the 80 kV data to generate the impression of a regular 120 kV image. Images were reconstructed at a slice thickness of 0.75 mm with an increment of 0.4 mm and a dedicated medium smooth kernel (D30f) in a standard abdomen window (width 400 HU, center 50 HU). However, for image interpretation, window settings could be freely adjusted for both the 80 kV and 140 kV image series. 2.3 Image interpretation Dual energy images were analyzed with dedicated software (Syngo workstation, Siemens). Both the 80 kV and the 140 kV series are loaded into the application simultaneously. The software supports a regular 3D view (axial, sagittal, coronal) for the gray scale images and allows to mix the 80 kV and 140 kV data in steps of 1%. First, stones were simply classified according to their CT patterns by visual impression at both 80 kV and 140 kV as homogenous hypodense, homogenous isodense, homogenous hyperdense, or mixed, if they showed more than one CT pattern—all compared to the surrounding formalin. The maximum diameter of each stone was measured. Then region of interest (ROI) measurements were performed using a circle tool. The software automatically displays the referring CT attenuation (Hounsfield units, HU) for 80 kV and 140 kV, simultaneously. ROI were drawn as large as possible. In homogenous stones, only one ROI was placed. In stones that consisted of various sectors of different densities on CT – e.g. core and shell – ROI measurements were performed separately for every visually identified sector. Further, the attenuation of water and formalin was determined. Beside mean CT number within a ROI also standard deviation (i.e. noise) was recorded. All measurements were performed in axial, sagittal, and coronal plane for each area (i.e. 3 measurements of the same area in total) to minimize the influence of potential measurement errors; thereof, a mean value was calculated, which later statistical analysis was based on. 2.4 Chemical analysis After DECT phantom scans were completed, the stones were analyzed with Fourier transform infrared spectroscopy (FTIR; IFS 28, Bruker, Karlsruhe, Germany). FTIR spectroscopy is an analogue to regular photometry, but instead of the visible spectrum of light, infrared light is used. The substance to be analyzed is embedded in a ground matrix (potassium bromide–KBr), which is lucent for IR light. Afterwards, the sample is exposed to a known IR spectrum and transmission and absorption of certain wavelengths are measured behind the sample. By transmission/absorption characteristics, the substances contained in the sample can be identified. Preparation of the stones was done with the help of the DECT images. Stones that appeared homogenous on CT were powdered in toto, embedded in the KBr matrix, and analyzed. For stones with more than one component on DECT, each identified sector was analyzed separately. For that, representative parts of the stones were cut or scratched off with a scalpel as accurately as possible in concordance with the DECT images. FTIR spectroscopy measurements were allocated and verified with the help of computer-based libraries for reference spectra. Results were displayed semi-quantitatively: stone composition could be described in steps of ten percent. As only gallstones with a high percentage of cholesterol and without major calcification seem to show good results with dissolution therapy, the main goal of this work was to find criteria for the reliable identification of such pure cholesterol compounds on DECT. We constituted a threshold of 70% of cholesterol and no calcium compound to define “pure” cholesterol on FTIR, whereas less than 70% of cholesterol or the presence of any percentage of calcium defined “non-cholesterol” sectors [28] . 2.5 Statistical analysis Dedicated software was used for statistical analysis (BiAS 8.4, Epsilon, Frankfurt, Germany). Mean ± standard deviation was calculated for the measured CT attenuations and compared using the Wilcoxon–Mann–Whitney- U test. The corresponding CT values for 80 kV and 140 kV were plotted in a scattergram. Based on the attenuation measurements, sensitivity and specificity were calculated for DECT compared to FTIR to detect gallstone components with a high content (>70%) of cholesterol and no calcium components (“pure” cholesterol components). 3 Results The mean attenuation of formalin was determined to be 16 ± 2 HU with a standard deviation (SD) (i.e. noise) measured within the ROI of 12 ± 1 HU at 80 kV and 15 ± 2 HU with a SD of 10 ± 1 HU at 140 kV. Water showed attenuation of −5 ± 0 HU with a SD of 13 ± 0 HU at 80 kV and −4 ± 0 HU and a SD of 12 ± 0 HU at 140 kV. CTDIvol was 17.8 mGy. Of total 35 stones, 12 were not visible at 140 kV regardless of window settings, but all stones were visible at 80 kV. Because of that phenomenon, further analysis and ROI measurements were based on the 80 kV image series. At 80 kV, 25 stones showed homogenous attenuation ( n = 13 hyperdense, n = 12 hypodense), whereas in 10 stones shell and core could be differentiated (7 stones with hypodense core and hyperdense shell, 3 stones with hyperdense core and more hyperdense shell) ( Fig. 1 ). Following that, ROI measurements were performed in totally 45 sectors. Results were plotted in a diagram ( Fig. 2 ). Mean stone diameter was 1.3 cm (range 0.4–2.4 cm). The smallest single sector that was measured was a 2 mm thick hyperdense shell; the referring ROI had a size of 3 mm 2 . The smallest homogenous hyperdense and hypodense stones that were measured had a diameter of 4 mm and 5 mm with a size of the referring ROI of 17 mm 2 and 20 mm 2 , respectively. All 19 sectors that appeared hypodense at 80 kV and were invisible at 140 kV were grouped in the lower left part of the curve and showed slightly negative attenuation values at 80 kV (−1 to −15 HU) and positive attenuation values at 140 kV (+17 to +29 HU). On FTIR spectroscopy, identified stone components for the 45 sectors were cholesterol, bilirubin, protein, carbonates, and phosphates. The carbonates turned out to be modifications of calcium carbonate. Phosphates were accompanied with calcium as counter ion as well. Based on that, FTIR spectroscopy determined 20 sectors with >70% of cholesterol and no calcium compound and 25 sectors with either <70% of cholesterol or any calcium compound. DECT recognized 19 out of these 20 sectors with certain characteristics of attenuation: Every sector showed slightly negative attenuation at 80 kV (mean −8 ± 4 HU, range −15 to −1 HU, with a SD (i.e. noise) within the ROI of 12 ± 2 HU) and positive attenuation at 140 kV (mean 22 ± 3 HU, range 17–29 HU, with a SD within the ROI of 11 ± 2 HU). Attenuation at both, 80 kV and 140 kV was significantly different from that of formalin and water ( p < 0.001), whereas noise was not ( p > 0.9). These 19 sectors showed the above mentioned phenomenon, that they were visible at 80 kV and invisible at 140 kV. In contrast, 25 of the 26 sectors that were visible at 140 kV contained somewhat amount of calcium or less than 70% of cholesterol. Table 1 provides a detailed overview of the results obtained by DECT and FTIR spectroscopy. Thus, DECT could differentiate 44 out of 45 measurements correctly: 19 as being “pure” cholesterol sectors (true positive) and another 25 as being “non-cholesterol” sectors (true negative). Based on these values, we determined a sensitivity of 95% and specificity of 100% for DECT compared to FTIR spectroscopy to differentiate between “pure cholesterol” and “non-cholesterol” sectors ( Table 2 ). The attenuation values of the 25 sectors that were classified as “non-cholesterol” sectors by FTIR spectroscopy ranged from 26 HU to 2176 HU at 80 kV (mean 429 ± 478 HU) and from 40 HU to 1491 HU at 140 kV (mean 315 ± 328 HU) and were significantly higher than the attenuation of “pure” cholesterol sectors ( p < 0.001), and there was no overlap of attenuation values, neither at 80 kV, nor at 140 kV. Likewise, sectors with >70% cholesterol but with up to 10% calcium ( n = 5/25) also showed significantly ( p < 0.001) higher attenuation values than “pure” cholesterol sectors (80 kV: mean 241 ± 195 HU, range 26–520 HU; 140 kV: mean 186 ± 129 HU, range 40–364 HU), also with no overlap. 4 Discussion Predicting the composition of gallstones by CT has been widely explored in the past. To our knowledge, these studies were mostly based on single source CT using only one predefined tube potential [7,9,12,14–18,20–22,29] . Inverse correlation between stone density and cholesterol content could be demonstrated. But especially attenuation threshold for cholesterol largely diversified in these studies ranging from 50 HU to 140 HU, and showed a relevant overlap with other stone components [9,15,17,18,20,22] . In this study we found out that with DECT it is possible to circumscribe attenuation for cholesterol gallstones without a wide diversity. Restricting CT numbers from −1 to −15 HU at 80 kV and from +17 to +29 HU at 140 kV, we defined stone components with >70% of cholesterol and no calcium under in vitro conditions. These values allow the detection of “pure” cholesterol components of gallstones (>70% of cholesterol and no calcium) with a sensitivity of 95% and enable the differentiation to “non-cholesterol” stone components with a specificity of 100%. As we have also shown, the detection of gallstones with >70% of cholesterol is limited to the fact that even a small percentage of calcium components (1–10%) influences attenuation significantly, and it was not possible to detect them with DECT as being cholesterol components in this study. The core of stone 1 showed hyperdense attenuation around 60 HU at both tube voltages and was therefore not identified as cholesterol sector on DECT. But spectroscopy revealed a composition of 80% cholesterol, 10% protein, 10% of bilirubin, and no calcium compound (false negative). Assuming that both DECT and FTIR measurements were correct, no reliable explanation for this finding can be given. A significant influence of bilirubin seems to be unlikely, as stone 3 consisted of 20% bilirubin with 70% cholesterol and was correctly classified as cholesterol stone. In general, the observed results led us to the conclusion that the presence and ratio of cholesterol and calcium compounds strongly affect attenuation characteristics on DECT. For example, the shell of stone 19 showed non-cholesterol characteristics, although containing only 10% of calcium but 80% of cholesterol ( Table 1 ). Vice versa, stone 32 offered no cholesterol and no calcium compounds on FTIR spectroscopy, but could also be successfully classified as a non-cholesterol stone ( Table 1 ). Showing both conditions, containing no cholesterol, but 40% of calcium, stone 12 resulted in higher attenuation values than the shell of stone 19 (sector no. 19-2) and 32 ( Table 1 ). In this context, proteins and bilirubin do not seem to have significant influence on the appearance of gallstone on DECT. Gallstone disease concerns abdominal medicine day by day. Associated with evaluated risk factors like body mass index (BMI), increasing age, diabetes, high triglyceride, and low HDL cholesterol [30] , the incidence of gallstones is rising among an ageing population and upcoming metabolic syndrome [31] . Imaging the stone is important to ensure diagnosis, and comparisons between different imaging modalities have been drawn. Although CT is significantly superior to plane film X-ray and seems to be on par with US in the detection of gallstones [32] , not every stone is detected by single source single energy CT. This may be due to the fact, that the standard default tube voltage for most of the CT protocols in adults is 120 kV, and that this X-ray spectrum may not be suitable to detect “pure” cholesterol stones. In this study, 19 of 20 “pure” cholesterol sectors were only visible at 80 kV and could not be delineated at 140 kV regardless of window settings. With CT, many authors especially focussed on the detection of cholesterol in order to change therapy for affected patients [7–10,12,16,17,22] . Although nowadays the therapy of choice is cholecystectomy, symptomatic patients who refuse surgery or show a contraindication to surgery or anaesthesia are to be treated with therapeutical alternatives (oral gall acids, extracorporeal shockwave lithotripsy, contact litholysis, ezetimibe) [3–6,11,13,29] . The above mentioned studies have described a correlation between low CT numbers and gallstone clearance, arising thereby that these options demand non- or only low-calcified, high content cholesterol stones for a successful therapy. Dual source CT (DSCT) with the option of operating both tubes in dual energy mode offers unseen perspectives compared to single energy CT regarding material differentiation in clinical routine: different authors have successfully applied DECT for the differentiation of urinary tract stones (uric acid from calcified stones) [33–36] and the detection of iodinated contrast material in different tissues of the body [37–40] . The dual energy technique is based on the fact that attenuation of different materials and tissues varies with the tube potential, and therefore X-ray spectrum, they are scanned with. The spread and ratio of attenuation values at a high and low tube voltage – and therefore the dual energy effect – depend on effective atomic number and will expand with a bigger difference in X-ray spectrum to a certain point and can be characteristic for certain materials and tissues [27] . However, as for clinical routine use compromises need to be made in terms of image noise and applied dose, a tube voltage of 140 kV and 80 kV on tubes A and B, respectively, is used for most clinical DECT applications, so far. Different tube potential settings with single source CT have been tested for gallstone differentiation in the past. Chan et al. [19] scanned gallstones in vitro with voltages of 80 kV, 100 kV, 120 kV, and 140 kV, separately, and found the detection rate of gallstones at 140 kV to be the highest. They further described a relevant overlap of CT values of cholesterol and pigment stones, so that a reliable identification was not possible in every case, although cholesterol showed lower attenuation at 80 kV than at 140 kV—like in our study. The discrepancy with our findings may be explained by some substantial differences to our study concept. First, on FTIR spectroscopy stones were analyzed in toto and not sectorwise—a core/shell appearance on CT was not considered for chemical analysis. Further, stones were classified only according to their main component into cholesterol ( n = 71) and pigment ( n = 15) stones. No quantitative analysis was performed, and no information was given about the amount of calcium compounds and how they affected CT density. Second, only a single ROI per stone was placed on CT images encompassing as much of the stone as possible on 5 mm thick slices. This limits spatial resolution and average areas of different density and therefore does not differentiate between areas of different chemical composition. Altogether, this may explain the overlap and wide range of CT values of cholesterol and pigment stones. Third, stones were put into NaCl solution for phantom measurements which has been shown to significantly alter attenuation values of gallstone components compared to measurement in native bile, more than formalin [41] . Further, no information was given how CT values of saline solution at the different kV settings changed. However, the density characteristic of NaCl seems to be very similar to that of water [27] . Based on our results, cholesterol shows somewhat water-like density only at 80 kV, thus leading to higher absolute contrast between cholesterol and NaCl at 140 kV than at 80 kV. This might account for the finding, that gallstone detection rate was significantly higher at 140 kV than at 80 kV. Recently, Voit et al. [42] reported their initial results for gallstone differentiation with the same dual energy scanner as in our study. Forty-three gallstones (13 cholesterol stones) were embedded in ultrasound gel and scanned at 80 kV and 140 kV. While analysis of only the gray scale images by two observers showed a poor sensitivity of 54% with a good specificity of 85–89% for the correct classification of the 13 cholesterol stones, colour-coding of HU differences within the stones improved sensitivity and specificity to 100%. Unfortunately, no mean CT numbers and ranges were given for stone components in the paper. The main limitation of the study was that stone differentiation was only performed according to visual criteria by one pathologist. No chemical analysis was done. 4.1 Study limitations Our study has some limitations that have to be considered. First, 35 stones and 45 measurements represent a small sample size. A larger amount of stones could provide more precise values for sensitivity and specificity. Second, test conditions were optimized in an in vitro set-up with a small phantom diameter of only 20 cm and therefore a noise-optimized setting. This allowed us to use a thin collimation of 32 × 0.6 mm with reconstruction of 0.75 mm slices for improved spatial resolution, whereas for patient use a collimation of 14 × 1.2 mm is recommended by the manufacturer and in literature [25] to compensate for higher noise. In vivo conditions – especially the influence of the patient's body habitus and increased image noise in large patients – have not been examined yet. In this context, radiation dose also has to be considered. The CTDIvol of our scans was 17.8 mGy. We did not change recommended tube current settings of 83 mAs (140 kV) and 340 mAs (80 kV). This was seen as a compromise between the use of a small phantom (which would allow for reduction of tube current) and a thin 0.6 mm collimation (which would require an increase in tube current). It needs to be discussed if in an anthropomorphic phantom also a thin collimation can be applied, how dose and image quality would differ from a thick collimation protocol, and further to what level dose can be reduced to still guarantee diagnostic image quality for in vivo use. Third, formalin could possibly influence the CT attenuation of gallstones, even though stone storage in formalin seems to have the least effect on changes in CT value besides storage in native bile [41] . The use of frozen stones for further trials needs to be considered, as a potential interaction of conservation fluids with stone components can be eliminated that way. Fourth, the way samples for FTIR analysis were generated, may be another source of error: Unfortunately it is not guaranteed that the region of the stone that was measured in the DECT images exactly agreed with the particles cut off the stone and analyzed in laboratory. However, to our knowledge only two prior studies also tried to differentiate and analyze regions of different appearance on CT separately [15,18] . However, we think this represents the right approach for the most exact differentiation of stone components. It appears only logical, that areas of different densities on CT have different chemical compositions. Averaging back a high resolution CT image with placing only one ROI would be against the sense of this study, whose purpose was to determine stone components as exactly as possible. 5 Conclusion In this study we were able to show that compound analysis of gallstones with dual energy CT is feasible. Compared to chemical analysis with infrared spectroscopy serving as reference standard we were able to precisely describe characteristic CT numbers for high-percent cholesterol sectors without calcium components at 80 kV and 140 kV and to detect these sectors with 95% sensitivity and 100% specificity. However, recognizing the above mentioned limits of this study, our results from this phantom trial under optimized conditions may serve following investigations as reference. In vitro studies with anthropomorphic phantoms or better in vivo trials are needed to clarify the value of our findings and their reproducibility for clinical routine use. References [1] Gleisberg C, Bauer H, Fellmann E, et al. Cholezystektomie. Web site of Bundesgeschäftsstelle Qualitätssicherung GGmbH. http://info.bqs-online.de/outcome/12n1/Buaw-2002-12n1-qr.pdf . Published 2002. Updated October 2003. Accessed October 20, 2008. [2] E.A. Shaffer Gallstone disease: epidemiology of gallbladder stone disease Best Pract Res Clin Gastroenterol 20 6 2006 981 996 [3] L. Carrilho-Ribeiro A. Pinto-Correia J. Velosa M. Carneiro De Moura A ten-year prospective study on gallbladder stone recurrence after successful extracorporeal shock-wave lithotripsy Scand J Gastroenterol 41 3 2006 338 342 [4] T. Rabenstein M. Radespiel-Troger L. Hopfner Ten years experience with piezoelectric extracorporeal shockwave lithotripsy of gallbladder stones Eur J Gastroenterol Hepatol 17 6 2005 629 639 [5] A. Hellstern U. Leuschner A. Benjaminov Dissolution of gallbladder stones with methyl tert-butyl ether and stone recurrence: a European survey Dig Dis Sci 43 5 1998 911 920 [6] H.H. Wang P. Portincasa N. Mendez-Sanchez M. Uribe D.Q. Wang Effect of ezetimibe on the prevention and dissolution of cholesterol gallstones Gastroenterology 134 7 2008 2101 2110 [7] A. Caroli G. Del Favero F. Di Mario Computed tomography in predicting gall stone solubility: a prospective trial Gut 33 5 1992 698 700 [8] X.B. Fu J.Y. Liu G.N. Liu X.M. Shao X.S. Zhou Computed tomography in predicting the efficacy of oral cholelitholysis with bile acids Chin Med J (Engl) 106 10 1993 734 738 [9] M.S. Hickman W.H. Schwesinger J.D. Bova W.E. Kurtin Computed tomographic analysis of gallstones. An in vitro study Arch Surg 121 3 1986 289 291 [10] S.P. Pereira M.J. Veysey C. Kennedy S.H. Hussaini G.M. Murphy R.H. Dowling Gallstone dissolution with oral bile acid therapy. Importance of pretreatment CT scanning and reasons for nonresponse Dig Dis Sci 42 8 1997 1775 1782 [11] M.L. Petroni R.P. Jazrawi P. Pazzi Ursodeoxycholic acid alone or with chenodeoxycholic acid for dissolution of cholesterol gallstones: a randomized multicentre trial. The British-Italian Gallstone Study group Aliment Pharmacol Ther 15 1 2001 123 128 [12] R. Polverosi R. Sbeghen C. Zambelli F. Caracciolo F. Spigariol A. Caroli Role of computerized tomography in the densitometric assessment of lithiasis of the gallbladder Radiol Med 84 4 1992 387 392 [13] I. Tuncer M. Harman R. Mercan The effects of ursodeoxycholic acid alone and ursodeoxycholic acid plus low-dose acetylsalicylic acid on radiolucent gallstones Turk J Gastroenterol 14 2 2003 91 96 [14] J.A. Barakos P.W. Ralls S.A. Lapin Cholelithiasis: evaluation with CT Radiology 162 2 1987 415 418 [15] R.L. Baron C.A. Rohrmann Jr. S.P. Lee W.P. Shuman S.A. Teefey CT evaluation of gallstones in vitro : correlation with chemical analysis AJR Am J Roentgenol 151 6 1988 1123 1128 [16] G.D. Bell R.H. Dowling B. Whitney D.J. Sutor The value of radiology in predicting gallstone type when selecting patients for medical treatment Gut 16 5 1975 359 364 [17] K. Brakel J.S. Lameris H.G. Nijs O.T. Terpstra G. Steen B.C. Blijenberg Predicting gallstone composition with CT: in vivo and in vitro analysis Radiology 174 2 1990 337 341 [18] J.A. Brink B. Kammer P.R. Mueller D.M. Balfe E.L. Prien J.T. Ferrucci Prediction of gallstone composition: synthesis of CT and radiographic features in vitro Radiology 190 1 1994 69 75 [19] W.C. Chan B.N. Joe F.V. Coakley Gallstone detection at CT in vitro : effect of peak voltage setting Radiology 241 2 2006 546 553 [20] P. Janowitz A. Zoller W. Swobodnik J.G. Wechsler K.A. Schumacher H. Ditschuneit Computed tomography evaluation of radiolucent gallstones in vivo Gastrointest Radiol 15 1 1990 58 60 [21] A.A. Moss R.A. Filly L.W. Way In vitro investigation of gallstones with computed tomography J Comput Assist Tomogr 4 6 1980 827 831 [22] A. Rambow M. Staritz U. Wosiewitz P. Mildenburger M. Thelen K.H. Meyer zum Buschenfelde Analysis of radiolucent gallstones by computed tomography for in vivo estimation of stone components Eur J Clin Invest 20 4 1990 475 478 [23] T.G. Flohr C.H. McCollough H. Bruder First performance evaluation of a dual-source CT (DSCT) system Eur Radiol 16 2 2006 256 268 [24] T.R. Johnson K. Nikolaou B.J. Wintersperger Dual-source CT cardiac imaging: initial experience Eur Radiol 16 7 2006 1409 1415 [25] A. Graser T.R. Johnson H. Chandarana M. Macari Dual energy CT: preliminary observations and potential clinical applications in the abdomen Eur Radiol 19 1 2009 13 23 [26] T.R. Johnson B. Krauss M. Sedlmair Material differentiation by dual energy CT: initial experience Eur Radiol 17 6 2007 1510 1517 [27] L. Zatz The effect of the kVp level on EMI values Radiology 119 1976 683 688 [28] I.S. Kim S.J. Myung S.S. Lee S.K. Lee M.H. Kim Classification and nomenclature of gallstones revisited Yonsei Med J 44 4 2003 561 570 [29] R.P. Sarva S. Farivar H. Fromm W. Poller Study of the sensitivity and specificity of computerized tomography in the detection of calcified gallstones which appears radiolucent by conventional roentgenography Gastrointest Radiol 6 2 1981 165 167 [30] D. Festi A. Dormi S. Capodicasa Incidence of gallstone disease in Italy: results from a multicenter, population-based Italian study (the MICOL project) World J Gastroenterol 14 34 2008 5282 5289 [31] F. Lammert J.F. Miquel Gallstone disease: from genes to evidence-based therapy J Hepatol 48 Suppl. 1 2008 S124 S135 [32] D. Pickuth R.P. Spielmann Detection of choledocholithiasis: comparison of unenhanced spiral CT, US, and ERCP Hepatogastroenterology 47 36 2000 1514 1517 [33] A. Graser T.R. Johnson M. Bader Dual energy CT characterization of urinary calculi: initial in vitro and clinical experience Invest Radiol 43 2 2008 112 119 [34] B.R. Matlaga S. Kawamoto E. Fishman Dual source computed tomography: a novel technique to determine stone composition Urology 72 5 2008 1164 1168 [35] A.N. Primak J.G. Fletcher T.J. Vrtiska Noninvasive differentiation of uric acid versus non-uric acid kidney stones using dual-energy CT Acad Radiol 14 12 2007 1441 1447 [36] P. Stolzmann H. Scheffel K. Rentsch Dual-energy computed tomography for the differentiation of uric acid stones: ex vivo performance evaluation Urol Res 36 3–4 2008 133 138 [37] C. Fink T.R. Johnson H.J. Michaely Dual-energy CT angiography of the lung in patients with suspected pulmonary embolism: initial results Rofo 180 10 2008 873 879 [38] F. Pontana J.B. Faivre M. Remy-Jardin Lung perfusion with dual-energy multidetector-row CT (MDCT): feasibility for the evaluation of acute pulmonary embolism in 117 consecutive patients Acad Radiol 15 12 2008 1494 1504 [39] B. Ruzsics H. Lee P.L. Zwerner M. Gebregziabher P. Costello U.J. Schoepf Dual-energy CT of the heart for diagnosing coronary artery stenosis and myocardial ischemia-initial experience Eur Radiol 18 11 2008 2414 2424 [40] S.F. Thieme C.R. Becker M. Hacker K. Nikolaou M.F. Reiser T.R. Johnson Dual energy CT for the assessment of lung perfusion-correlation to scintigraphy Eur J Radiol 68 3 2008 369 374 [41] S.J. Schulte R.L. Baron The effect of storage on the computed tomography attenuation of gallstones Invest Radiol 29 3 1994 307 312 [42] H. Voit B. Krauss M.C. Heinrich Dual-source CT: in vitro characterization of gallstones using dual energy analysis Rofo 181 4 2009 367 373
更多查看译文
关键词
Dual energy computed tomography,Compound analysis,Tissue differentiation,Gallstones,Cholesterol
AI 理解论文
溯源树
样例
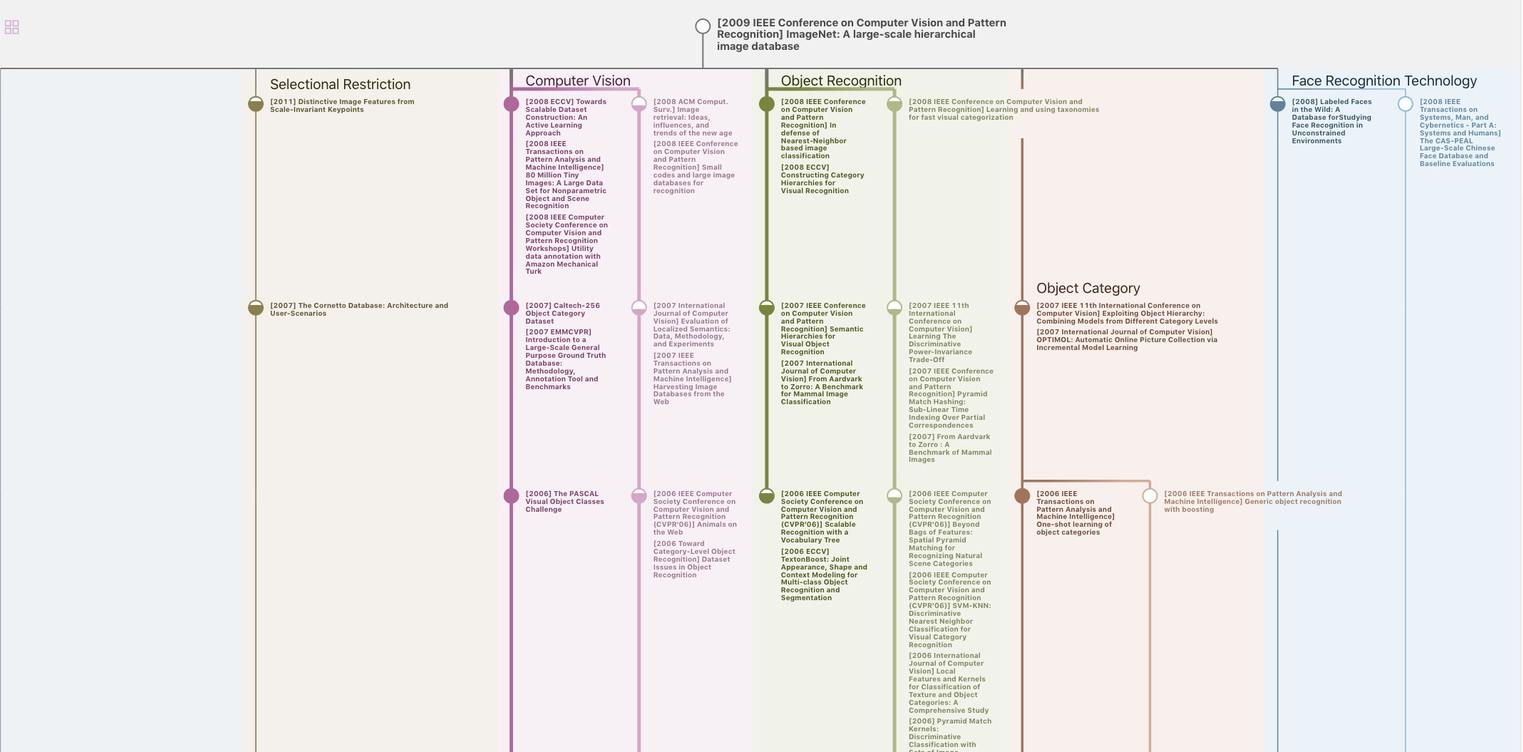
生成溯源树,研究论文发展脉络
Chat Paper
正在生成论文摘要